18.3 Types of Models Used in Intervertebral Disc Research
Intervertebral disc research involving various regions of the spine has been useful in assessing the physical, histological, and biomechanical characteristics of the disc (Panjabi 1998). In vitro models have also played a substantial role in providing an understanding of the unique cellular biology of the disc. Unfortunately, in vitro models generally lack the complexity of the intact system, which may limit the ability of researchers to draw definitive clinical conclusions from the scientific findings. Additionally, in vitro models generally do not provide an understanding of long-term experimental effects of an intervention.
Advances in computer technology have led to the development of some in silico models. These computer-based simulations are suitable for forming initial hypotheses that may serve as the rationale for further research. Computer simulations also allow the investigator a level of control over experimental variables that is typically not possible with in vitro and in vivo studies. With respect to intervertebral disc research, in silico models have seen limited utilization in the study of mechanical properties of the spine through finite element analysis modeling or the modeling of material properties of the disc (Galbusera et al. 2011).
The testing of novel therapeutics for human applications generally requires the use of in vivo studies. With in vivo research, investigators are able to observe the impact of treatment on a living biologic system (Panjabi 1998). Given the complexity of intervertebral disc tissue and the multifactorial nature of the degenerative process, in vivo research plays a crucial role in the development of human therapeutics (Lotz 2004; Singh et al. 2005). Additionally, before most therapeutics are considered suitable for human use, it is required that they are studied in an animal model with reasonable similarity to the human condition; this often requires the use of a large animal model (Alini et al. 2008).
18.3.1 The Clinical Perspective
From a clinical perspective, the treatment of disc degeneration itself is of limited value as most disc degeneration is not directly responsible for patient symptoms (this concept is discussed in great length in Chap. 16). However, disc degeneration associated with significant pain symptoms is common, and it is the associated pain and decreased quality of life that are the targets of therapy.
Unfortunately, a reliable means to quantify pain associated with disc degeneration in any animal model has not yet been defined. Therefore, animal research is generally limited to providing a means to study the effects of a potential therapy on the physical, cellular, or chemical milieu of the disc. The hypothesis of this line of research is that an intervention which provides improvement in objective measures of disc health would be likely to provide improvement in the pain associated with the degenerative process in affected human patients. Therefore, although animal testing of potential human therapeutics is important, favorable results still require validation in humans. Thus, animal model testing is just one step in the process of bringing a therapy from the bench top to the clinic (An et al. 2003).
18.3.2 Animal Models in General
As stated, animal models play a vital role in translational research and provide a crucial step in ensuring that a new therapy is valid and safe prior to considering human usage (Smith et al. 2011).
18.3.2.1 Standardization and Reliability
When an animal model is first introduced, it is essential to evaluate its reliability. Using the new model, independent investigators performing similar experiments should find comparable and reproducible results. Significant variation in results between investigators or unpredictable results from repetitive experiments may indicate that the model used is unreliable.
With animal models, standardization of the test animal’s age plays a key role in achieving adequate reliability for disc research. Other factors such as breed, gender, size, living conditions, vertebral levels studied, and nutrition could potentially affect the outcome of a study intervention, and so these features should be well controlled in the experimental design. These factors should also be reported by the investigator performing the animal research so that others can replicate the study conditions.
18.3.2.2 Model Fidelity/Validity
Issues of validity and fidelity are important in the design of animal trials. There is little benefit in using an animal model with such substantial differences from human disease that the information gained is not clinically relevant. Because all animal models are significantly different from the human condition, this limitation is one of magnitude. The researcher should seek to use a model that provides the closest possible replication of the human condition, understanding that a perfect match is not possible.
For many studies involving therapeutic interventions, an important factor is identifying a model with similar characteristics in terms of disc size and geometry (Beckstein et al. 2008). Wide variations in disc size across species are obvious, and this can affect the mechanical and biologic properties of the disc (Elliott and Sarver 2004; O’Connell et al. 2007). However, Beckstein et al. (2008) demonstrated that mechanical tissue properties of many species are conserved evolutionarily and that variations with regard to the biomechanical properties of the intervertebral disc are not as drastic as previously thought. Unfortunately, the current literature fails to provide comprehensive comparisons between animal models across a wide range of species. Most comparative studies published to date compare the properties of a specific animal species relative to the human.
18.3.2.3 Complications Associated with Validity/Fidelity
One factor which complicates animal model selection is related to intervertebral disc development. The nucleus pulposus is embryologically derived from notochordal cells. In humans, notochordal cells which are common at birth become rare following the first decade of life (Urban et al. 2000). Notochordal cells are believed to produce greater amounts of proteoglycan than other disc cells and contribute positively to the maintenance of disc extracellular matrix integrity (Palmer and Lotz 2004; Aguiar et al. 1999; Kalichman and Hunter 2008). In addition to wide variation in the persistence of these cells on a species-by-species basis, exposure to high biomechanical loads on the spine may hasten the disappearance of notochordal cells (Lotz et al. 1998; Iatridis et al. 1999; Palmer and Lotz 2004).
Variability in the presence and number of notochordal cells across species is important given their potential in resisting disc degeneration (Lotz 2004). In fact, Berry (1961) demonstrated that a breed of pin-tailed mice with low notochordal mitotic rates develops degeneration earlier than those with a more rapid rate of cell replication. This relationship between notochordal cells and disc degenerations may complicate the validity of many current animal models (Omlor et al. 2009) as certain species, particularly small animals (Higuchi et al. 1982) and dogs (Aguiar et al. 1999), have notochordal cells present throughout most of their lives. Differences in the number or qualitative characteristics of notochordal cells across species may manifest itself clinically as variation in the onset of disc degeneration (Hunter et al. 2004).
Animal models may also differ in the mechanism leading to the onset of the degradative process in the disc. Generally speaking, the etiology of disc degeneration in an animal model can be divided into spontaneous and experimentally induced degeneration (Lotz 2004) with the latter being further subdivided into models which are induced by either mechanical or structural alterations (Lotz 2004). Mechanical perturbations involve changes in the distribution or magnitude of loads placed on a disc, whereas structural perturbations involve chemical or physical injury to the disc itself (Lotz 2004). In contrast, human intervertebral disc degeneration usually occurs spontaneously (Lotz 2004) or possibly in response to a poorly defined mechanism of injury. Although spontaneous disc degeneration is present in many animal species, well-characterized models of spontaneous degeneration generally have involved smaller animals such as the Mediterranean sand rat (Silberberg 1988) and genetically altered mice (Lotz 2004). The value of the sand rat in disc research is discussed in considerable detail in Chap. 20. One counterexample, however, is chondrodystrophic dogs which have an abnormality in chondrocyte proliferation and maturation that also impacts the cells of the nucleus pulposus. With chondrodystrophy, there is accelerated disc degeneration, making these dogs a potential preclinical model for the human condition.
18.4 Large Animal Models
Sheep, goats, pigs, mini pigs, runt cows, nonhuman primates, dogs, chickens, kangaroos, and ostriches are all large animal species that have been used or discussed for disc research. However, the most commonly used species to date have been the pig, sheep, goat, and dog (Wilke et al. 1997a, b).
One concern regarding the use of the most commonly used large animal models relates to their quadruped nature (Wilke et al. 1997a, b). Unlike quadrupeds, humans are bipedal, using unique motions and body positions during ambulation and rest (Zhang et al. 2011a, b). This biomechanical limitation must be acknowledged when considering quadrupedal animals for research. Although some animals use bipedal ambulation (e.g., kangaroo, ostrich) and some could be considered partial bipeds (nonhuman primates), none provide an accurate replication of human gait including the upright torso position, sagittal balance of the spine, and the almost horizontally positioned discs during ambulation. Tissue processing of large animal spine specimen can be a challenge at times. To process isolated motion segments containing various devices often requires resorting to un-decalcified histology using plastic embedding. Careful tissue processing starting with adequate tissue fixation yields good-quality histological sections at an average thickness of 8 μm. Figures 18.1, 18.2, and 18.3 represent key features from ovine lumbar intervertebral discs with various degrees of degeneration. While there is little evidence of intervertebral disc degeneration days following the surgical intervention (chemonucleolysis using chondroitinase ABC), at 6 and 18 months, the degenerative process is well advanced. Specimens were stained using a Sirius red/Alcian Blue stain developed in our laboratory as a method for morphologic identification of collagen and proteoglycans in sections of non-decalcified disc specimens embedded in methyl methacrylate and sectioned on a rotary microtome at 4–8 μm.
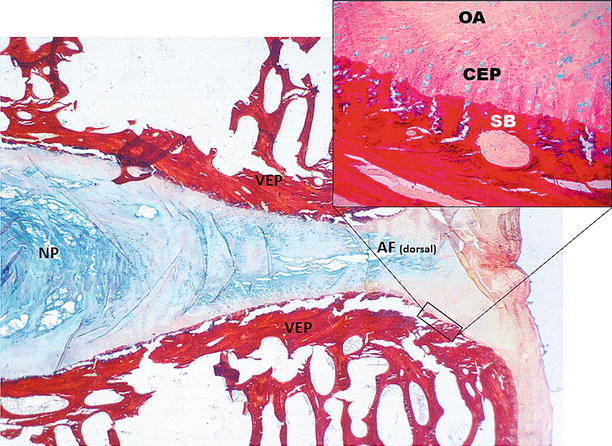
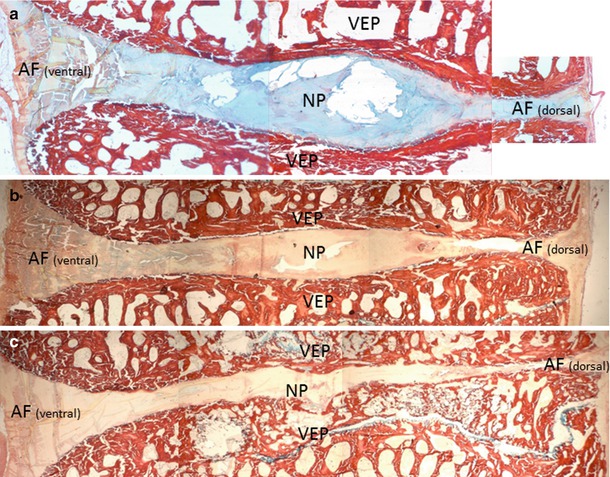
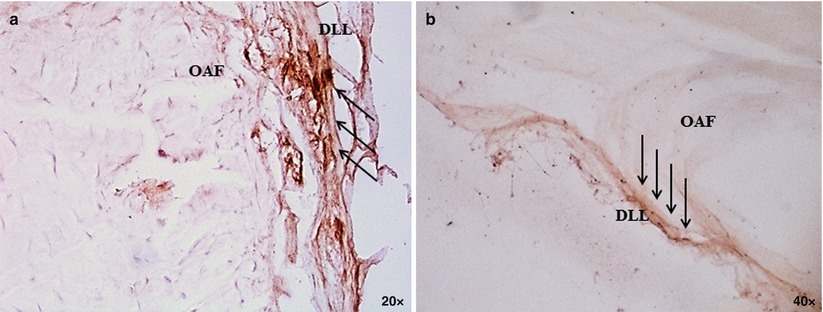
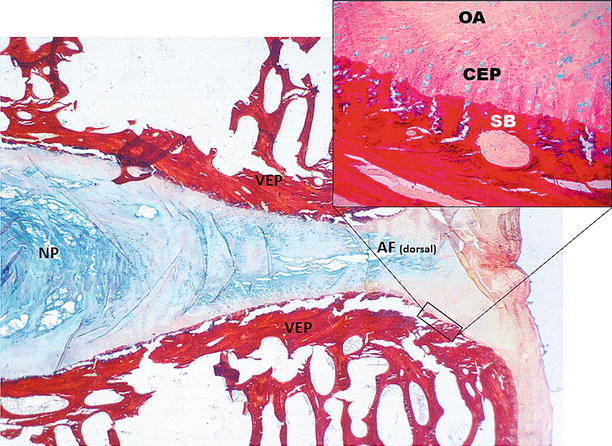
Fig. 18.1
Sections of two ovine vertebral bodies (L4–L5) and intervertebral disc. Low-power (orig mag ×1) view of a section stained with Picrosirius Red/Alcian Blue demonstrates normal intervertebral disc morphology consisting of vertebral endplates (VEP, annulus fibrosus AF, and nucleus pulposus NP). The cartilaginous disc stains an intense blue. Note the high content of collagen (red) in cortical and trabecular bone and the distinctly heterogeneous staining of collagen (red) and proteoglycan (blue) in the disc. Insert: high-power view of Picrosirius Red/Alcian Blue stained section (×40). Proteoglycan-positive chondrocytes and surrounding matrix stain blue. Residual collagen stains red (SB subchondral bone, CEP cartilaginous endplate, OA outer annulus); unstained areas (picric acid) appear yellow
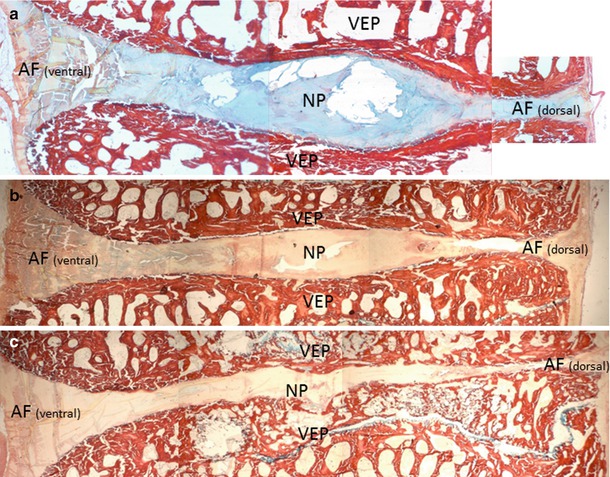
Fig. 18.2
Plastic embedded sagittal sections of ovine lumbar motion segments (L4–5) stained with Picrosirius Red/Alcian Blue at day 0 (a), 6 months (b), and 18 months (c) postoperative. Choosing a left lateral percutaneous approach through a 19-g docking needle, sheep were injected with 300 μl of chondroitinase ABC via the dorsolateral corner into the center of L4–5 discs using a 22-g spinal needle. Figure (a–c) demonstrates progressive loss of overall disc height associated with decreased proteoglycan content in the nucleus pulposus (NP) and loss of vertebral endplate integrity with established subchondral bone thickening (b, c). VEP vertebral endplate. Sections were photographed at 4×
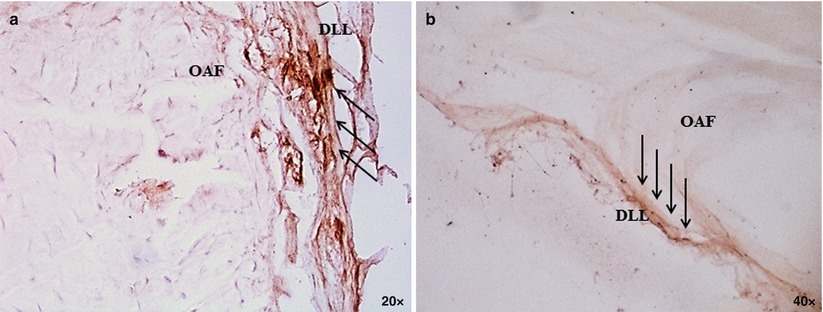
Fig. 18.3
Intervertebral disc degeneration (IVDD) per se is not a basis for clinical intervention: identification of specific features underlying discogenic pain is of the utmost importance to advance the current level of care and identify novel therapeutic targets. One critical deficiency in large animal models (sheep, goat, and cattle) is the lack of perceptible pain during surgically induced IVDD. Symptoms derived from degenerating discs may be classified into two types: (1) radicular pain secondary to stenosis and nerve-root or cord irritation and (2) discogenic pain due to internal disc disruption. These syndromes may have distinct etiologic bases. Certain dog breeds that exhibit an insidious onset of IVDD may provide clues to the pathophysiology of discogenic pain. A preclinical model correlating the clinical course of IVDD as it is seen in the humans would greatly enhance the predictability of clinical outcome when evaluating novel therapeutic concepts. Figure (a) and (b) demonstrates sparse positive immunoreactivity (arrows) to CGRP and SP confined to the dorsal longitudinal ligament (DLL) and outermost annulus fibrosus (OAF) in the lumbar disc of dogs without clinical history of back problems. These findings support current knowledge of neuroanatomic similarities between the healthy human and canine intervertebral discs
18.4.1 Porcine
Multiple studies of the intervertebral disc have relied on porcine models. A number of lines of evidence suggest that the porcine model is a reasonably good model of the human disc. At the gene expression level, changes associated with aging in swine are similar to those observed in humans (Cho et al. 2011). Substantial morphologic similarities have been observed in the porcine disc post-nucleotomy and the human degenerative disc. Omlor et al. (2009) found significant decreases in disc height, MRI signal intensity, and notochordal cell number in the porcine disc after nucleotomy (Omlor et al. 2009). Kaapa et al. (1994b, c) studied the effect of pig disc injury on collagen content and metabolism and found dramatic alterations in collagen levels and collagen biosynthesis along with decreased intradiscal water content. Holm et al. (2007) used a structural porcine disc injury model, in which intradiscal pressure was used to assess degeneration. Using two separate injuries (one to the annulus and the other to the endplate), intradiscal pressure was measured while applying biomechanical loads (Holm et al. 2007). The authors found that the ratio of injured to adjacent disc pressure was highest in the annulus injury model, signifying that the endplate injury was a more severe type of injury. Although this type of injury may lead to morphologic and biochemical changes which replicate human disc degeneration, the traumatic effects of the disc injury may also introduce unintended effects that limit the interpretation of this type of research.
Other studies have used pigs to assess the therapeutic value of various interventions or to examine specific changes associated with degeneration. Buser et al. (2011) evaluated the use of fibrin sealant after nucleotomy to determine if intradiscal injection of the sealant had a therapeutic effect on the degenerating intervertebral disc. With injection of the sealant, the authors observed an inhibition of fibrosis and improvements in proteoglycan levels (Buser et al. 2011). Similarly, Chiang et al. (2011) demonstrated that sealing of annular defects via the modified purse-string suture reduced degenerative changes in the disc following the injury.
Kaapa et al. studied porcine disc innervation and the effects of degeneration on nerve topography. The authors characterized nerve density, finding that in common with humans, the outer annulus contained most of the nerve endings (Kaapa et al. 1994a). Interestingly, this study found that disc injury had no effect on nerve topography (Kaapa et al. 1994c).
18.4.2 Ovine
The ovine model has also been used widely as a model for disc research. Melrose et al. (2012) used annular incisions to create mechanical destabilization and degeneration in sheep. Three months after surgery, MRI examination demonstrated a reduction in disc height. Biomechanical testing to assess range of motion and to measure the neutral zone was performed along with histopathological examination. Measurement of aggrecan breakdown along with the expression of proteoglycan and collagen indicated that there were progressive degradative changes. In an earlier study, 8 months after annular injury of the lumbar discs of sheep, Melrose et al. (1992) found that while proteoglycan and collagen levels had decreased, the amount of non-collagenous protein had increased. The changes were primarily found in the nucleus pulposus and not the annulus fibrosus (Melrose et al. 1992). A subsequent study by the same investigators revealed increased expression of decorin and biglycan after annular damage. These proteins exhibit adverse effects on cell proliferation in vitro and may further suppress the limited ability of the disc to heal after injury (Melrose et al. 1997). A later study by Melrose et al. (2002) suggested that the ovine annular lesion model can be extended to study neural and vascular ingrowth during the degradative process, a phenomenon hypothesized to play a role in the pain experienced by some human patients.
18.4.3 Canine
The canine model has also been popular in disc research. Various insults have been applied to canine discs in order to induce disc degeneration. Hutton et al. (2004) hypothesized that disc degeneration could be produced in canine models by limiting nutritional flow through application of bone cement to the endplates; however, 70 weeks postsurgery, no changes were observed (Hutton et al. 2004). In other studies, canine lumbar discs were subjected to compressive forces using springs (Hutton et al. 1998, 2000). Despite application of springs over an entire year, the authors reported that there were no gross discal changes. They did, however, find differences in proteoglycan and collagen content between the control and experimental groups. A study by Hasegawa et al. (1995) demonstrated that fatigue loading was more detrimental to vertebral bodies adjacent to nucleotomized discs when compared to controls. Cyclic compression loading of nucleotomized discs resulted in an increase in microcrack density on the adjacent vertebral bodies suggesting a possible microtrauma mechanism for progressive disc degeneration (Hasegawa et al. 1995).
Several investigations have tried to slow or reverse intervertebral disc degeneration in canine models via direct transplantation of autologous cells (Ganey and Meisel 2002; Ganey et al. 2003, 2009). One study assessed the value of autologous adipose tissue-derived stem cells in treating damaged discs (Ganey et al. 2009). In each dog, three sequential discs were injured via partial nucleotomy, while two adjacent discs were spared and served as controls (Ganey et al. 2009). Of the three discs with partial nucleotomies, one was injected with stem cells in a hyaluronic acid carrier, one was injected with hyaluronic acid alone, and the last underwent no intervention. Based on assessments of the overall disc morphology and matrix production subsequent to transplantation, the authors reported that treatment with stem cells in a hyaluronic carrier provided superior results. In another study, Ganey et al. (2003) harvested autologous disc cells, cultured them ex vivo, and returned them to the original canine disc via a percutaneous method. Not only did the transplanted cells successfully reintegrate into the disc, they also produced extracellular matrix. With respect to disc water content, follow-up MRI was consistent with disc regeneration. Similarly, Hiyama et al. (2008) were also able to show signs of regeneration in a canine post-nucleotomy model through the injection of autologous disc cells expanded in culture.
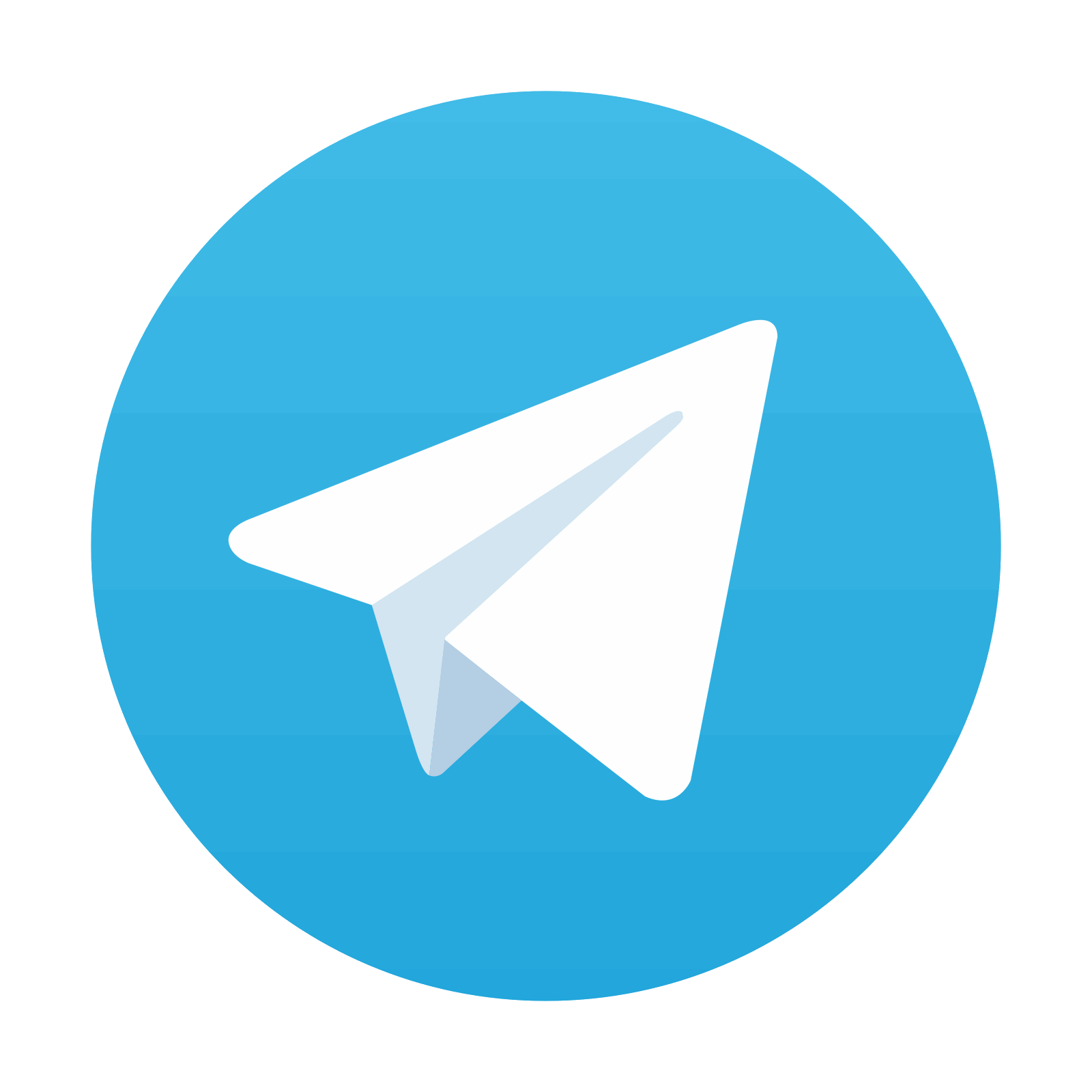
Stay updated, free articles. Join our Telegram channel

Full access? Get Clinical Tree
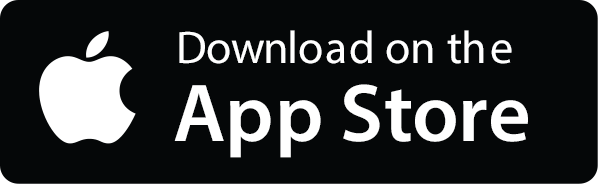
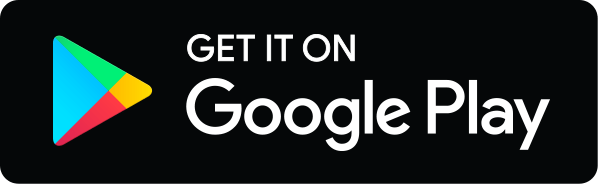