Chapter 18 External fixation offers various advantages compared with other fixation methods.1 It is a fast, relatively simple, and atraumatic technique.2 It enables, in the majority of cases, easy reduction of the fracture, even under unfavorable soft tissue conditions, and secondary corrections can be made.3 Furthermore, it is possible to modify fixation stiffness during treatment.1 This enables proper management of the healing process.4,5 Technological evolution has been a significant contributing factor in improving the external fixator stiffness and eliminating its mechanical inadequacy, a major problem in the past.6 Finally, external fixation treatment is comparatively cost-efficient.7 Despite these positive qualities, there persists a high incidence of complications due to the progressive mechanical deterioration of the bone-pin interface, which leads to pin loosening and infection.8–12 Often, these complications are severe enough to necessitate interruption of treatment due to a lack of fixation strength, or the spread of infection from the pin tracts causing osteomyelitis.5,13–16 Deterioration of the bone-pin interface strength is an inevitable phenomenon with standard pins. Chao and Aro5 stated that “there seems to be a race between the gradually increasing loading capacity of a healing bone and failure of the bone-pin interface.” Pin extraction torque has been measured in many studies.2,9,17,18 In all studies of standard pin types, pin extraction torque was lower than pin insertion torque. This confirms that there is a progressive reduction of pin anchorage during treatment. Aro et al13 demonstrated in an animal study that the extraction torque of Orthofix tapered pins decreased by approximately 80% at 12 weeks compared with the corresponding insertion torque (Orthofix, Bussolengo, Italy). Similar results were found using Apex cylindrical (Howmedica, Rutherford, NJ) and Superfixation bicylindrical (Citieffe, Lippo di Calderara di Reno, Italy) pins.9,17,18 In a clinical study on tibial fractures the extraction torque of Apex cylindrical and Superfixation bicylindrical pins was lower than the corresponding insertion torque. No difference in extraction torque was found between the two pin types.10 The causes of pin loosening and infection are multifactorial. Thermal and mechanical damage of the bone during pin insertion and formation of fibrous tissue at the bone-pin interface have been identified as causes of pin loosening and infection.18 Both damaging factors can be limited but not completely avoided with standard pins. In many studies, fibrous tissue formation at the bone-pin interface has been identified as the most important factor in pin loosening.9,11,19 This fibrous tissue formation is thought to be an inevitable phenomenon with stainless steel and titanium pins.11 However, differences in bone-pin contact related to different metal types have been reported. A higher osteointegration was reported in pins coated with titanium compared with similar pins made of stainless steel.11 To improve pin fixation and consequently avoid infection of the pin tracts, some authors developed the idea of coating the pins. The first example of coated pins dates back to 1913 when Lambotte coated the pins with nickel and gold to protect against rust.20 More recently, Manley et al1 used ultrahigh molecular weight polyethylene as a coating material. With these pins there was a reduction of approximately 50% in maximum compressive stress. However, ultrahigh molecular weight polyethylene is not an osteoconductive material, and furthermore, no in vivo study was performed. Recently, thanks to technological progress in the science of biomaterials, a new technique has been devised. Pins coated with hydroxyapatite have been developed to improve osteointegration and consequently the mechanical stability of the bone-pin interface (Fig. 18–1).10,17,21,22 The literature provides convincing evidence that sintered apatite is capable of forming a tight, durable link with the underlying metallic surface and living bone tissue. Due to its high biocompatibility and low degradation, this bio-ceramic material has been used as a coating for metallic prostheses.18,23 If hydroxyapatite is plasma sprayed onto the prosthesis surface, the time needed to obtain adequate fixation strength is shortened.18,24 Furthermore, the fixation strength is increased and so is the amount of bone-prosthesis apposition.18,24 This is why this coating is now applied to several implant devices, from hip prostheses to dental implants.9 FIGURE 18–1 Photograph showing (A) a hydroxyapatite-coated tapered pin and (B) a standard tapered pin. (Reprinted with permission from Magyar G, Toksvig-Larsen S, Moroni A. Hydroxyapatite coating of threaded pins enhances fixation. J Bone Joint Surg Am 1997;79:487–489.) In this chapter, the results of animal and clinical studies investigating pins coated with hydroxyapatite in different bone types will be reported. The purpose was to compare, in a sheep tibial model, the radiographic, biomechanical, and histomorphometric interface properties of three different groups of tapered external fixation pins: uncoated, coated with hydroxyapatite, and coated with titanium. One hundred and eight stainless steel (ASTM F138)5/6-mm diameter, tapered pins were divided into three groups of 36 (Orthofix, Bussolengo, Italy). Group A pins remained uncoated, Group B pins were plasma sprayed with hydroxyapatite, and Group C pins were plasma sprayed with CP titanium (ASTM F67–89). The crystallinity ratio of hydroxyapatite, calculated by roentgen ray spectroscopy, was greater than 70%, and purity by mass spectroscopy was greater than 97%. The calcium-to-phosphate ratio was 1.67 ± 0.01. The hydroxyapatite powder contained small traces of heavy elements, which were below the limits set by the American Standard for Testing and Materials F1185–88 standard test. The mean coating thickness was 56 ± 19μ m in Group B pins and 47 ± 11μ m in Group C pins. The mean surface roughness was Ra = 0.3 ± 0 in Group A pins, Ra = 4.9 ± 0.3 in Group B pins, and Ra = 6.2 ± 0.8 in Group C pins. Eighteen adult female sheep of similar size and body mass (45 ± 5 kg mean body mass) were selected for this study. Six pins of the same group were implanted in the anterior-lateral part of the left tibia, three in the proximal tibia, and three in the distal. Pins were numbered from 1 to 6, proximal to distal. A 4.8 mm diameter drill was used to predrill a hole in the tibial cortices, and then the pins were manually inserted. After pin implantation, a unilateral external fixator was mounted on the pins (Orthofix, Bussolengo, Italy). The medial tibial middiaphysis was then exposed and a 5 mm long bone cylinder was removed between the proximal and distal pin groups to allow bone load to pass through the bone-pin interfaces. Unrestricted weight bearing was allowed immediately after the operation. The sheep were euthanized 6 weeks after the operation. Five pins from each sheep were used for the biomechanical analyses (pin numbers 1, 2, 3, 5, and 6) and one pin from each sheep for the morphological analyses (pin number 4). Radiographic rarefaction of the bone pin tract was observed in 29 pins of Group A, 15 pins of Group B, and 30 pins of Group C (Group A vs. Group B, P= 0.021; Group B vs. Group C, P= 0.016) (Table 18–1, Fig. 18–2). Healing of the osteotomy site was observed in only two sheep of Group B. Mean final insertion torque was 4360 ± 1050 N•mm in Group A, 3420 ± 676 N•mm in Group B, and 3740 ± 643 N•mm in Group C (nonsignificant). Mean pin extraction torque was 253 ± 175 N•mm in Group A, 3360 ± 1260 N•mm in Group B, and 1720 ± 1030 N•mm in Group C (Group A vs. Group B, P= 0.002; Group A vs. Group C, P= 0.017; Group B vs. Group C, P= 0.03). Extraction torque was significantly lower compared to the corresponding insertion torque in both Group A (P<0.001) and Group C (P= 0.003). In contrast, the hydroxyapatite-coated pins showed no significant difference between extraction and insertion torque (Table 18–1). No pin failed during removal. Bone-pin contact at ×60 magnification was 16 ± 9% in Group A, 30 ± 12% in Group B, and 26 ± 15% in Group C (Group A vs. Group C, P= 0.042) (Table 18–2). At ×60 magnification, histological examination showed many areas of bone resorption, fibrous tissue encapsulation, and low bone-pin contact in Group A (Fig. 18–3). Better osteointegration and many areas of direct bone-pin contact were seen in Group B (Fig. 18–4). At ×60 magnification the osteointegration in Group C looked very similar to Group B but at ×10,000 magnification no real bone-pin contact was observed between titanium and bone (Fig. 18–5). In all titanium-coated pins a gap of 1 to 3μ m was found. In contrast, in Group B contact was seen between bone and hydroxyapatite even at ×10,000 magnification (Fig. 18–6). No infection of the bone-pin tract was seen in the histological observations of any pin. There was no coating breakage or sloughing in the hydroxyapatite-coated pins. Histological observations of the bone holes after pin removal showed no fractures in the bone surrounding the pinhole in any pin group. Neither the uncoated nor the titanium-coated pins left metallic particles in the bone after pin removal. The hydroxyapatite-coated pins left small fragments of hydroxyapatite approximately 10μ m thick in direct contact with the bone holes.
ENHANCED STABILITY OF
EXTERNAL FIXATION WITH
HYDROXYAPATITE-COATED PINS
ANIMAL STUDY
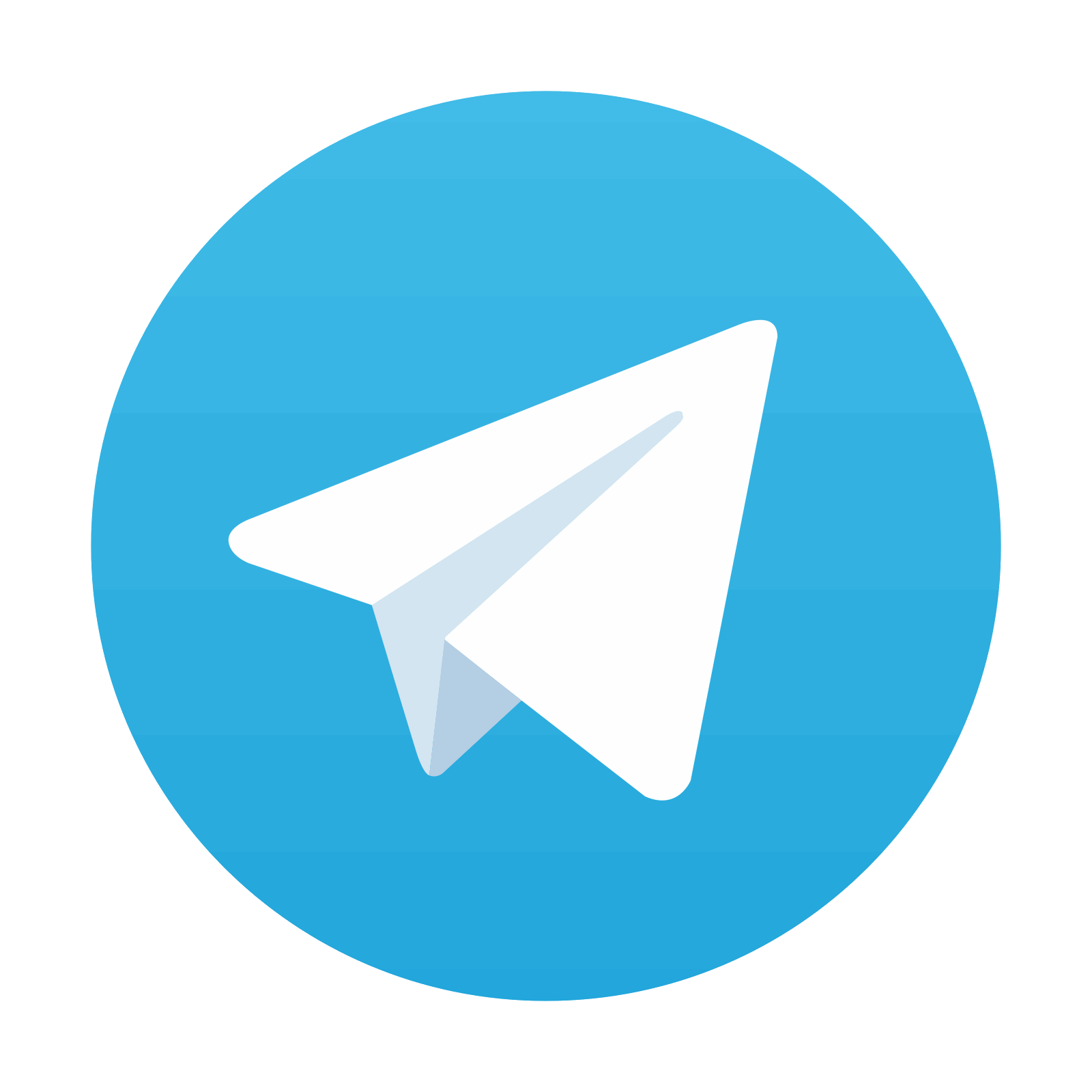
Stay updated, free articles. Join our Telegram channel

Full access? Get Clinical Tree
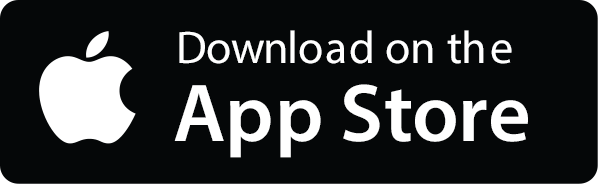
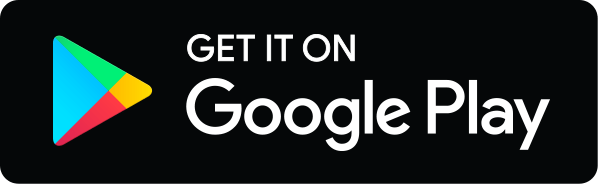