Fig. 24.1
The PCL receives direct arterial branches from the medial superior genicular artery which is a branch of middle genicular artery
With respect to collateral ligament blood supply, there are anatomic features that may potentially impact the healing potential, when compared to the inherent healing abilities of cruciate ligaments. The medial collateral ligament (MCL) has multiple branching blood vessels throughout the epiligament, with some of these vessels penetrating into the substance of the ligament itself [10, 11]. In contrast, the blood supply of the ACL is limited to one or two branches of the middle genicular artery, which branches along the surface of the ligament, beneath the synovial covering [11, 12].
24.2.2 Intra-articular and Extra-articular Ligament Healing
The ability for ligamentous injuries about the knee to heal is variable, depending on the specific ligament involved, and the severity of injury. There are a number of proposed reasons as to why the healing of intra-articular ligament injuries of the knee is less reliable than healing of those ligaments composed of fibers located outside of the articular space [13]. Examining the healing response of intra-articular ligaments compared to extra-articular ligaments, there has been research demonstrating no significant difference in the biological processes of cellular proliferation, cellular migration, and collagen production. The process of platelet fibrin clot formation, however, has been shown to be deficient in cases of intra-articular ligament injury [14]. Without this clot, the torn ligamentous fibers remain separated after injury, and subsequent cellular migration and tissue repair/remodeling is impaired. Furthermore, the presence of circulating plasmin in the joint space, which is capable of breaking down fibrin clot, has been postulated to be a reason for suboptimal or nonexistent clot formation [15]. Moreover, synovial fluid has been shown to inhibit ACL/PCL fibroblast proliferation and migration [16], thereby retarding tissue healing (Fig. 24.2).


Fig. 24.2
Stages of intra-articular ligament healing. (a) Migration of leucocytes and RBCs. (b) Lack of platelet fibrin clot formation, impairing ligament resynovialization of an injured ACL. (c) Histologic demonstration of longitudinal fiber derangement
It is well established that healing of injured medial and lateral collateral ligaments, without surgical intervention, is typically superior to that of the ACL and PCL. Variation in the healing potential of ACL and MCL has been studied in experimental animal models that have demonstrated a positive association of vascular supply and healing potential of these ligaments [17]. The body of the ACL has very sparse or no blood vessels, and the relative hypovascularity of the ACL, and the associated blunted physiological response to injury, may be a major factor in the diminished healing potential of this ligament.
In young, athletically active individuals, up to 90% of all knee injuries involve the MCL, ACL, or some combination of both [18, 19]. In the event of MCL injury where there is laxity exceeding 5 mm on valgus stress testing, there is increased likelihood of less-organized collagen alignment and increased residual laxity, which may lead to reduced valgus knee loading resistance post-healing. Biomechanical deficits that may present after ACL reconstruction in cases of associated MCL injury often necessitate a delay in patient rehabilitation advancement with respect to activities that require sudden running directional changes and single leg jump landings [20]. This reinforces the importance of restoring an effective MCL checkrein function, particularly where a patient has increased ligamentous laxity about the knee, or decreased dynamic neuromuscular stiffness.
Valgus instability after healing of the MCL has been attributed to histologic defects that include smaller than normal collagen fibril size and abnormal collagen cross-linking [21, 22]. Biologic therapies that have the potential to impact collagen reformation and orientation after such injuries have, therefore, stimulated great clinical interest. The application of growth factors may stimulate development of mechanically stronger MCL tissue that is better able to withstand valgus knee stress, compared to the tissue expected to arise from natural healing processes. Others have proposed the use of trephination to treat lesions of the MCL in order to increase the concentration of growth factors that accumulate about the injured ligament [23, 24]. Cross-fiber massage is a further treatment method that has been proposed, and this refers to the manual application of forces transverse to the direction of underlying collagen fibers, with the goal of inducing physiological and/or structural tissue changes [24].
Differing healing environments in cases of MCL or LCL injury may significantly affect knee joint kinematics under valgus and varus tibial torques [25]. Migration and proliferation of ligament fibroblasts are essential for the healing of ligament injuries. Various cytokines have been demonstrated to act in a dose-dependent manner with respect to cellular migration and proliferation in cases of ligament fibroblasts and the subsequent production of repair tissue [26]. Optimizing the healing conditions using biologic supplementation, including the application of growth factors, may lead to the formation of repair tissue of superior quality, although evidence-based research to conclusively support such treatments remains preliminary.
24.3 Surgical Repair of Ligament Injury
Repair of the ACL or PCL, whether partially or completely torn, has been attempted previously and is well described in the literature [27, 28]. Unfortunately, failure rates of this surgical treatment have been reported to be as high as 90% [28, 29]. As knowledge has progressed, there is now greater insight into processes of ligament healing, and solutions have been put forward to address obstacles such as clot deficiency after intra-articular ligament rupture. These therapies can be broadly categorized into three groups: cellular, structural, and composite solutions. These therapeutic applications may be used in isolation or to augment a surgical repair procedure.
24.4 Biologic Augmentation of Ligament Healing and Repair
Cellular therapies have the potential to address deficiencies in the healing of injured ligament by treating damaged tissue at a molecular level, thereby providing an enhanced biologic environment for repair processes to proceed. Steadman’s “healing response therapy” was one of the earliest treatments to highlight the role of pluripotent stromal cells (PSCs) derived from bone marrow in aiding the healing of ruptured ligaments in human subjects [30, 31]. Clinical results of this therapy were encouraging, and have been attributed to the pluripotent nature of bone marrow cells. Pluripotent stromal cells were initially isolated from bone marrow, and have since been isolated from a number of other tissues such as fat, muscle, skin, connective tissue, skin, and bone. The phenotypic plasticity of these cells has generated a considerable enthusiasm to use them in repairing or regenerating connective tissue with ex vivo tissue engineering or in situ techniques. Recent findings of similarities between ligament outgrowth cells and PSCs suggest the possibility to modulate these cells to enhance healing of repaired ligaments [32].
The technique of primary ACL/PCL repair with bone marrow stimulation has been used at our institution for the past 15 years [33, 34], and we have demonstrated that marrow stimulation, using microfracture technique, in conjunction with repair of a partially torn ACL or PCL, reliably leads to restoration of ligamentous stability and enables return to sporting activities in young adults. In our prospective case series involving 50 patients (mean age 28.3 years), it was demonstrated that ACL primary repair combined with bone marrow stimulation and platelet-rich plasma (PRP) injection is an effective technique to restore knee stability and function in young athletes with acute partial ACL tears, after 5 years of follow-up [35].
24.4.1 Preferred Technique
The patient is placed supine and given either general or spinal anesthesia. After sterile draping and preparation, a complete knee examination is performed. Examination tests of knee stability are performed, followed by Rolimeter testing (Don Joy). Routine arthroscopic examination of the knee is performed, carefully noting cruciate ligament integrity. Partial tearing of the ACL or PCL is confirmed by probing the individual bundles at varying flexion angles, and with the knee in a figure-of-4 position. Lachman and jerk tests are performed under direct visualization of the ligaments to supplement the examination of ligamentous injury. After identification of the injured ligament fibers, polydioxanone suture no. 1 (Ethicon, Piscataway, New Jersey) is then passed through and around the residual distal and proximal fibers using a standard clever hook, and tied using a Duncan loop to reappose the torn ligament (Fig. 24.3a). The role of these sutures is to reapproximate the torn fibers in order to minimize gapping between the residual tissues, thereby recreating continuity of the ligament. Subsequent healing is promoted by adjacent marrow stimulation within the notch to facilitate the release of PSCs (Fig. 24.3b). Microfracture perforations, 1.5 mm in diameter, 3–4 mm apart, and 3 mm deep, are made around the anatomic femoral insertion of the injured intra-articular ligament. Great care is taken to avoid damaging the preserved ligamentous insertion. Release of marrow elements from the microfracture site is confirmed under direct visualization. In cases where the ACL fibers appear stretched but not interrupted, we perform marrow stimulation without ligament suturing.


Fig. 24.3
(a) Suturing of partially torn ACL to remaining fibers using No.1 Polydioxanone suture. (b) Microfracture awl used to create subchondral perforations at the femoral attachment site, leading to the release of marrow elements
Platelet-rich plasma is then prepared using a commercially available system. The PRP is subsequently activated with batroxobin enzyme (Plateltex® act-S.R.O., Bratislava, SK) to produce a sticky PRP gel, which is then injected at the repaired site to biologically augment ligamentous healing. Recently, biologic augmentation of ligament repair has extended to the use of bone marrow aspirate concentrate (BMAC), which provides cellular augmentation, in addition to platelet-derived growth factors. As with PRP, the BMAC is activated using batroxobin to create an activated clot, which is then applied to the site of ligament repair under dry arthroscopy.
In our study period from 2004 to 2009 [33], there were 26 athletes included in the analysis with incomplete tears of the ACL who underwent primary repair, followed by bone marrow stimulation about the ACL femoral attachment site and biologic augmentation. All patients participated in a specific rehabilitation program and were evaluated pre- and postoperatively by Marx, Noyes, Tegner, Single Assessment Numeric Evaluation, Lysholm, and International Knee Documentation Committee scores. Anterior tibial translation was measured using a Rolimeter under anesthesia, and at final follow-up. After a mean follow-up period of 25.3 months, all patients had excellent functional outcomes and had returned to sporting activities. Second look arthroscopy in six patients revealed that the repaired ACL bundles had excellent vascularity, minimal fibrous tissue, and good stability on probing. Partial PCL tears have also been treated with this technique at our institution, and patient clinical outcomes are currently undergoing analysis. In cases of combined ligamentous injury where there is low- to moderate-grade injury to the medial or lateral collateral ligament, the injured collateral ligament is typically treated with a biologic injection consisting of PRP or BMAC (Fig. 24.4). Isolated MCL and LCL tears that do not require surgical intervention are often treated with PRP injection in and around the injured ligament at our outpatient clinics (Figs. 24.5 and 24.6).




Fig. 24.4
Injection of bone marrow aspirate concentrate to treat a grade II lateral collateral ligament injury after arthroscopic ACL repair with biologic augmentation

Fig. 24.5
Graph demonstrating significant improvement of side to side difference in knee laxity as measured by a Rolimeter device 3 years following ACL repair surgery with biologic augmentation

Fig. 24.6
Tegner and Marx scores demonstrating significant (p < 0.05) improvements in clinical outcomes 3 years following ACL repair with biologic augmentation
24.4.2 Postoperative Rehabilitation
The operative knee is positioned into a brace, and partial weight bearing is allowed with crutches for 3 weeks. Continuous passive motion (CPM) is used initially at 20–60° of knee flexion, and is increased gradually until 90° is achieved by 2 weeks postoperatively, after which CPM is discontinued. At least 120° of flexion is expected by 6 weeks. Proprioceptive and strength training progresses systematically, with running activities commencing at 3 months. Return to high contact sports is delayed for a minimum of 4–5 months postoperatively.
24.5 Future Perspectives
24.5.1 Growth Factors and PRP
Growth factors have been shown to enhance cellular proliferation and migration, as well as increase collagen production, according to a number of in vitro studies. Various bioactive molecules are involved in orchestrating the cellular repair response after tendon repair [36]. Numerous growth factors are markedly upregulated following tendon injury and are active at multiple stages of the healing process, including insulin-like growth factor-1 (IGF-1), transforming growth factor beta (TGF-β), basic fibroblast growth factor (bFGF), platelet-derived growth factor (PDGF), vascular endothelial growth factor (VEGF), bone morphogenic protein (BMP), and connective tissue growth factor (CTGF) [37–40]. IGF-1 is an important mediator during the inflammatory and proliferative phase of tendon healing, while PDGF, FGF, BMP, and TGF-β have also been shown to play important roles in ligamentous healing.
Kobayashi et al. have reported improved healing and vascularity following instillation of FGF after canine ACL injury [41]. Aspenberg et al. demonstrated improved healing in cases of Achilles tendon injury treated with GDF 5 (BMP 14) [42]. These growth factors have been used in conjunction with synthetic scaffolds with the goal of enhancing ligament repair. Chen et al. examined the use of BMP 2 with hydrogel and periosteum to stimulate tendon-bone healing in a model of ACL reconstruction, and reported that PPC-BMP-hydrogel composite is an effective inducer of healing, being capable of acting as a matrix for encapsulation of cell and growth factors [43].
PRP has been defined as a volume of plasma fraction isolated from autologous blood with a platelet concentration above the baseline level (200,000 platelets/μL). Platelets contain many important bioactive proteins and growth factors. These factors regulate key processes in tissue repair, including cellular chemotaxis, migration, proliferation, differentiation, and extracellular matrix synthesis. The rationale for PRP use is to stimulate and augment the natural healing cascade by providing a “supra-physiologic” release of platelet-derived factors directly at the site of injury.
Autologous PRP can be obtained from simple venous blood extraction with a commercially available kit. After collection, the blood undergoes a centrifugation process to isolate PRP. For PRP gel preparations, platelets are typically activated by thrombin (autologous or animal derived), calcium chloride, or a procoagulant enzyme, such as batroxobin, which works as a fibrinogen-cleaving enzyme that induces rapid fibrin clot formation. When PRP isolates are injected into a site of tissue injury, platelets are activated by endogenous thrombin and/or intra-articular collagen. Depending on the preparation method, PRP can be categorized as leucocyte-rich PRP (L-PRP), leucocyte-poor PRP (LP-PRP), or platelet-poor plasma (PPP) [44]. The constituents of PRP preparations may vary, depending on which system is used to process the autologous blood. Our institution prefers to use PRP preparations rich in the growth factors PDGF, TGF-β, and IGF-1, which have been shown in vitro to be particularly effective in encouraging biologic healing processes (Figs. 24.7 and 24.8).



Fig. 24.7
The role of PRP and associated growth factors in tissue healing

Fig. 24.8
Autologous blood collected by venipuncture and centrifuged in a commercially available system to prepare PRP
Some researchers have questioned the beneficial effects of isolated PRP use in tissue healing. Murray et al. contested the beneficial role of PRP in ACL healing following the outcomes demonstrated in skeletally immature pigs that were treated with ACL repair in conjunction with PRP injection [45]. The addition of PRP to the sutured ACL repairs did not improve AP knee laxity, maximum tensile load, or linear stiffness of the ACL after 14 weeks in vivo, compared to a sample that underwent ACL repair without the addition of PRP. In an alternate porcine model of ACL repair, however, Murray et al. reported that the use of collagen-platelet composites did have beneficial effects on sutured ACL repair [46].
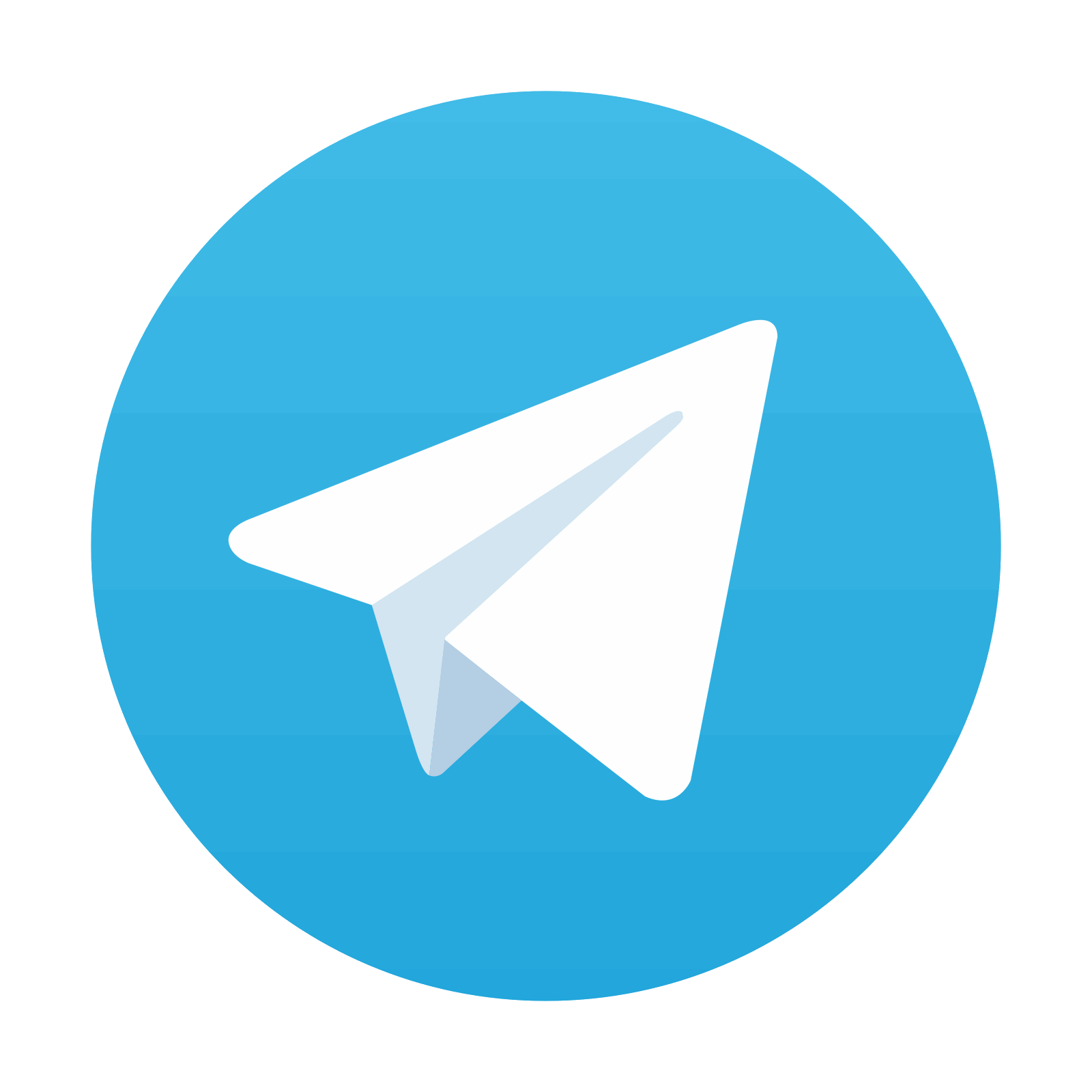
Stay updated, free articles. Join our Telegram channel

Full access? Get Clinical Tree
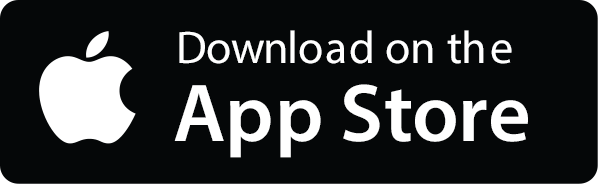
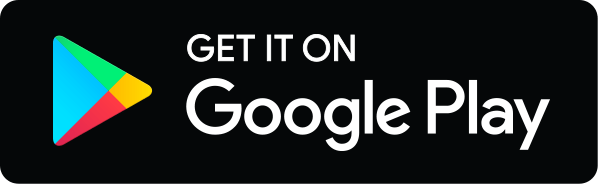