CHAPTER 3 Congenital Anomalies and Variational Anatomy of the Shoulder
VARIATIONAL ANATOMY OF THE SHOULDER
The anatomy of the shoulder has a wide range of normal arrangements. Some can play a role in the development of shoulder pathology, but most go completely unnoticed.
Clavicle
The clavicle serves as a strut between the scapula and the thorax. It possesses a unique double curve that accommodates the two very different motions of the sternal and acromial ends. The sternal end is essentially fixed but does rotate on its axis. The acromial end must match scapular motion by elevating 60 degrees with the arm fully abducted. The matched motion of the acromion and clavicle provides bony congruency and stability and thereby prevents internal rotation of the scapula throughout humeral elevation. Only 5 to 8 degrees of rotation occurs at the acromioclavicular joint with the arm moving through a full range of motion.1,2 In addition, the clavicle is the origin of one of the heads of the pectoralis major and the anterior head of the deltoid, which are essential in elevation of the arm.
The work of DePalma in 1957, in which variations in 150 clavicles were examined, found no two clavicles to have all the same characteristics.3 He described a definite relationship between the length of the clavicle and the amount of curvature. Within each curve of the clavicle, a circle can be drawn that uses the arc as part of the circle’s circumference. DePalma divided the length of the clavicle in inches by the summed radii of the circles in inches (Fig. 3-1) to produce a unitless index that he named the clavicle curve index. The range of values fell between 0.40 and 1.29. He divided the range into groups of 0.10, which resulted in nine groups (e.g., 0.40-0.49 for group 1 and 1.20-1.29 for group 9). Contrary to the work of Fich,4 who reported more severe curves in the dominant arm’s clavicle, the two groups showed similar curve characteristics.
Of more interest was the variation in anterior torsion of the lateral aspect of the clavicle. DePalma observed 66 specimens with their sternoclavicular and acromioclavicular joints intact and the sternum in a vertical position.3 The clavicles fell into three categories: type I, type II, and type III (Fig. 3-2).
From this study, it was observed that as the curve of the lateral part of the clavicle decreased, the distal end of the clavicle became thicker, with a more circular acromial end. Of the 66 specimens studied, 27 (41%) were type I, 32 (48%) were type II, and 7 (11%) were type III. Type I distal clavicles have the highest rate of degenerative changes.3 Either the decrease in surface area of the articulation or the vertical alignment producing higher shearing forces can lead to increased degeneration.
The acromioclavicular joint is in a plane that allows motion in all directions, including rotation. It is formed by the lateral edge of the clavicle and the acromion and contains a fibrocartilage disk. The ends of the bones are enveloped by a loose articular capsule that is reinforced by an inferior acromioclavicular ligament and a stronger superior acromioclavicular ligament (Fig. 3-3).
Acromioclavicular Joint
The acromioclavicular joint is supported by the acromioclavicular ligaments in the anteroposterior plane and the superoinferior plane. The undersurface of the lateral aspect of the clavicle serves as the attachment point for the strong coracoclavicular ligaments. These ligaments can be thought to serve two distinct functions. The first is to prevent superior migration of the clavicle as seen in acromioclavicular separations. The second is to suspend the scapula from the distal end of the clavicle as the shoulder elevates.
Although the coracoclavicular ligament complex functions as a single ligament, it is composed of two distinct ligaments. Posterior and medial is the conoid ligament. Its nearly vertical short, stout fibers form an inverted cone whose base is on the clavicle, extending posteriorly to the conoid tubercle of the clavicle. Its tapered apex is on the posteromedial aspect of the coracoid process. In a cadaveric study, Harris and colleagues reported three anatomic variants of the conoid ligament with differing characteristics at its coracoid insertion.5 The anterior and lateral segment of the coracoclavicular ligament, the trapezoid ligament, assumes a trapezoid shape. The fibers originate on the superior aspect of the coracoid process, just anterior to the fibers of the conoid ligament. These fibers course anterolaterally and insert on the inferior surface of the clavicle. The trapezoid ligament inserts at the mid arc of the lateral curve of the clavicle, with its most lateral attachment on the trapezoid ridge of the clavicle, averaging 15.3 mm from the distal end of the clavicle.5
The coracoacromial ligament originates on the anterior margin of the acromion and inserts on the posterior-lateral aspect of the coracoid. Pieper and colleagues reported variations in the coracoacromial ligament in 124 shoulders.6 Their findings were two distinct ligaments in 59.7% and one ligament in 25.8% of shoulders. They were also able to identify a third band located more posterior and medial than the conoid in 14.5% of shoulders. Very little variation was found in the dominant and nondominant shoulders of the same cadaver with regard to number of bands.
Over all specimens, the different types of coracoacromial ligament morphology had no side predilection. In a smaller cadaveric study, Holt and Allibone identified four anatomic variants of the coracoacromial ligament.7,8 A quadrangular variant was seen in 48% of shoulders and a broad band variant in 8%. A Y-shaped variant seen in 42% of shoulders was composed of two distinct ligaments. A multiple-banded variant was seen in 2% of shoulders containing a third band.8 Fealy and colleagues further described the two-banded ligament as being composed of an anterolateral and posteromedial band.9 In their study, three distinct bands were seen in 3% of shoulders, two distinct bands in 75%, and only one in 20%. The coracoacromial ligament was completely absent in 2% of shoulders. A lateral extension of the anterolateral band, termed the falx, is continuous with fibers of the conjoined tendon on its lateral aspect.
The presumed function of the coracoacromial arch has undergone much change in the past 60 years. Codman described it as a fulcrum that guided the head during abduction.10 It was subsequently found that the coracoacromial ligament could be sacrificed in the face of normal shoulder musculature without compromising shoulder function.3 However, in patients with massive rotator cuff tears, the presence of the coracoacromial arch limits superior migration of the humeral head. Thus, the coracoacromial ligament is not to be sacrificed without consideration.
Sternoclavicular Joint
The sternal end of the clavicle is roughly prismatic in shape. It is concave anteroposteriorly and convex vertically, creating a diarthrodial joint. It is larger than the posterolateral facet of the manubrium sterni and the cartilage of the first rib, and less than half of the medial clavicle articulates with the sternum.11,12 The clavicle therefore protrudes superiorly from its medial articulation. In addition to the size mismatch, a great deal of incongruence exists between the two surfaces. Nature has compensated by interposing a fibrocartilage disk to buffer the stress and strains of the joint (Fig. 3-4).
The intra-articular disk is slightly thicker at its periphery than at the center. It is also thicker superiorly than at its inferior pole. The disk takes its origin from a circular area on the posterior-superior portion of the medial part of the clavicle. It then inserts inferiorly into the junction of the sternum and the cartilage of the first rib. This orientation makes the disk a strong stabilizer of the medial part of the clavicle to elevation. The disk also divides the sternoclavicular joint into two compartments: the smaller diskosternal (inferomedial) and the larger diskoclavicular (superolateral).13 Perforation of the disk and resultant communication between the compartments occurs in 2.6% of shoulders.3 There also appears to be a degenerative pattern of increasing thinning of the inferior pole as one ages.
The joint is enclosed in a synovial capsule that also has attachment to the disk periphery. The surface of the capsule is reinforced by strong oblique fibrous bands, the anterior and the posterior sternoclavicular ligaments. These capsular ligaments are the most important structures in preventing superior displacement of the medial clavicle.14 The interclavicular ligament and the costoclavicular ligaments act to further stabilize the medial part of the clavicle. Along the superior aspect of the manubrium sterni courses the intersternal ligament, which links the clavicles. The costoclavicular or rhomboid ligaments are extracapsular and consist of an anterior and a posterior fasciculus. The anterior and posterior components of this ligament cross and run from the inferior medial portion of the clavicle to the cartilage of the first rib.
Structures lying immediately behind the sternoclavicular joint require familiarity for safe surgery in this area. Important vital structures include the dome of the parietal lung pleura, the esophagus, and the trachea. The sternohyoid and sternothyroid muscles lie directly behind the sternoclavicular joint. These muscles are much thicker and thus a more effective protective layer on the right. A sheath of fascia encompassing the omohyoid is continuous with the clavipectoral fascia, which encloses the subclavius and pectoralis minor muscles. This myofascial layer is anterior to the vessels as they travel from the base of the neck to the axilla (Fig. 3-5).
Coracoid Process and Adjacent Structures
The coracoid process is readily palpable in the infraclavicular region just under the anterior head of the deltoid. The coracoid is a landmark for surgeons and a reminder of the important structures in its vicinity. The process is a short, fat, crooked projection from the anterior neck of the scapula. It is directed anteriorly, laterally, and inferiorly from its origin. The process serves as an origin for the medial and proximal coracoclavicular ligaments and the anterior and lateral coracoacromial ligaments. The short head of the biceps, the coracobrachialis, and the pectoralis minor muscles all attach to the coracoid process.
The clavipectoral fascia, which is an offshoot of the axillary fascia, first envelops the pectoralis minor and then continues superiorly to surround the subclavius muscle and clavicle (Fig. 3-6). The pectoralis minor muscle runs in an inferior-to-medial direction to insert on the second through fifth ribs. The brachiocephalic veins exit the thorax behind the sternoclavicular joint and immediately divide into the internal jugular and subclavian veins. The internal jugular continues cranially within the carotid sheath. The subclavian vein curves laterally and inferiorly atop the anterior scalene muscle, then it ducks under the clavicle and subclavius muscle and over the first rib to become the axillary vein.
The arterial structures are the brachiocephalic trunk on the right and the left common carotid and left subclavian arteries (Fig. 3-7). These arteries are the first three major vessels off the aorta. The course of the arteries is similar to that of the veins. On the right, the brachiocephalic trunk divides posterior to the sternoclavicular joint and becomes the common carotid and subclavian arteries. The subclavian arteries also exit between the clavicle and first rib but course posterior to the anterior scalene muscle. The subclavian vessels become the axillary vessels as they emerge beneath the clavicle. The axillary vessels travel together with the brachial plexus anterior to the chest wall and posterior to the clavipectoral fascia.
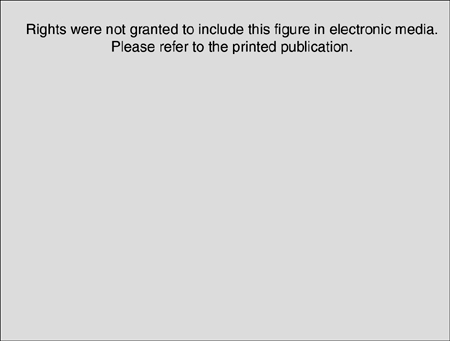
FIGURE 3-7 The subclavian artery and brachial plexus enter the axilla by crossing the first rib together.
(From DePalma AF: Surgery of the Shoulder, 3rd ed. Philadelphia: JB Lippincott, 1983, p 41.)
The interscalene triangle is bordered anteriorly by the anterior scalene muscle, posteriorly by the middle scalene muscle and inferiorly by the medial surface of the first rib. The costoclavicular triangle is bordered anteriorly by the middle third of the clavicle, posteriorly by the first rib, medially by the costoclavicular ligament, and laterally by the edge of the middle scalene muscle. The tight confines of these spaces enclose the contents of the major nervous and vascular supply to the upper extremity.
Any anomalous muscle or band predisposes the patient to the development of thoracic outlet syndrome. Several anomalies have been identified (Figs. 3-8 to 3-16).15
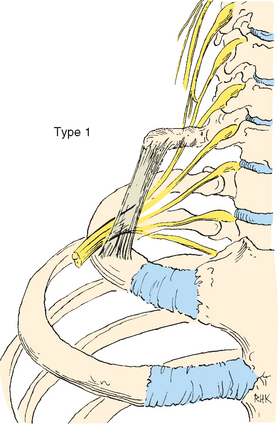
FIGURE 3-8 Type 1 fibrous band.
(Modified from Wood VE, Twito RS, Verska JM: Thoracic outlet syndrome: The results of first rib resection in 100 patients. Orthop Clin North Am 19:131-146, 1988.)
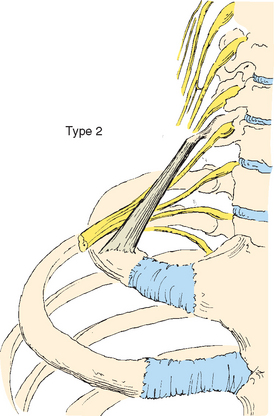
FIGURE 3-9 Type 2 fibrous band.
(Modified from Wood VE, Twito RS, Verska JM: Thoracic outlet syndrome: The results of first rib resection in 100 patients. Orthop Clin North Am 19:131-146, 1988.)
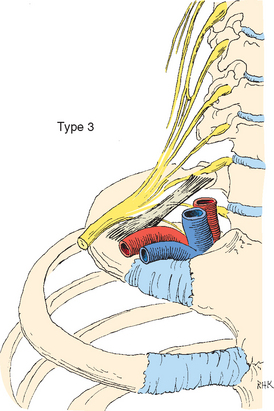
FIGURE 3-10 A type 3 fibrous band is the most common band in thoracic outlet syndrome.
(Modified from Wood VE, Twito RS, Verska JM: Thoracic outlet syndrome: The results of first rib resection in 100 patients. Orthop Clin North Am 19:131-146, 1988.)
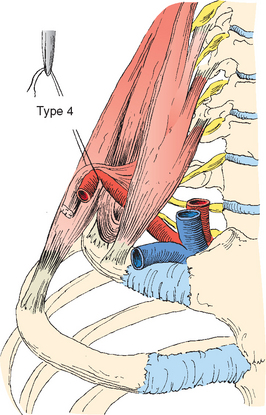
FIGURE 3-11 A type 4 fibrous band forms a sling.
(Modified from Wood VE, Twito RS, Verska JM: Thoracic outlet syndrome: The results of first rib resection in 100 patients. Orthop Clin North Am 19:131-146, 1988.)
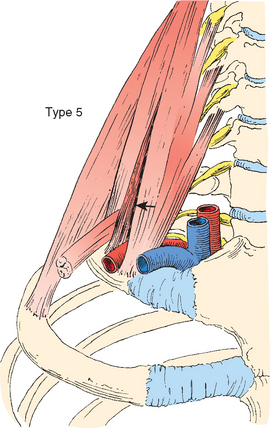
FIGURE 3-12 A type 5 band (arrow) is an abnormal scalenus minimus.
(Modified from Wood VE, Twito RS, Verska JM: Thoracic outlet syndrome: The results of first rib resection in 100 patients. Orthop Clin North Am 19:131-146, 1988.)
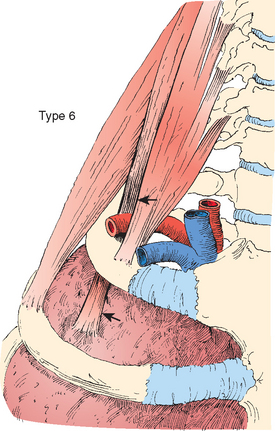
FIGURE 3-13 A type 6 band (arrows) inserts on Sibson’s fascia.
(Modified from Wood VE, Twito RS, Verska JM: Thoracic outlet syndrome: The results of first rib resection in 100 patients. Orthop Clin North Am 19:131-146, 1988.)
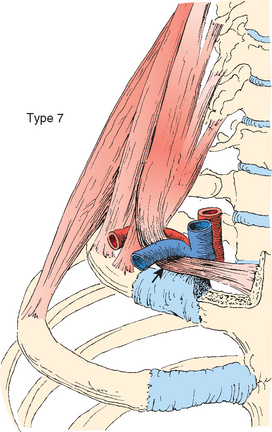
FIGURE 3-14 Type 7 fascial band (arrow).
(Modified from Wood VE, Twito RS, Verska JM: Thoracic outlet syndrome: The results of first rib resection in 100 patients. Orthop Clin North Am 19:131-146, 1988.)
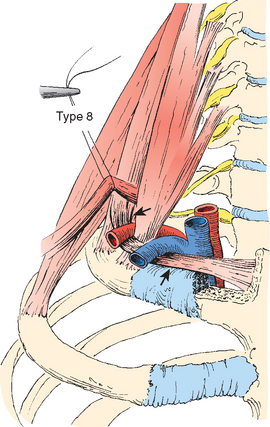
FIGURE 3-15 Type 8 fascial band (arrows).
(Modified from Wood VE, Twito RS, Verska JM: Thoracic outlet syndrome: The results of first rib resection in 100 patients. Orthop Clin North Am 19:131-146, 1988.)
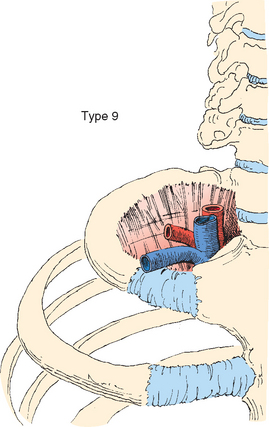
FIGURE 3-16 A type 9 fascial band entirely fills the inside of the first rib.
(Modified from Wood VE, Twito RS, Verska JM: Thoracic outlet syndrome: The results of first rib resection in 100 patients. Orthop Clin North Am 19:131-146, 1988.)
One variation consists of the brachial plexus exiting through the fibers of the anterior scalene. Roos16–18 identified nine types of anomalous fibrous or muscular band arrangements associated with thoracic outlet syndrome. Each anomaly is associated with a different type of compressive pathology.
In addition to the previous nine types identified, Roos also identified five patterns of bands associated with high cervical and median nerve neuropathy (Figs. 3-17 to 3-21).18 We make this distinction because the presence or absence of upper brachial plexus involvement can aid in the operative approach if the patient requires surgery for brachial plexus entrapment.18–20
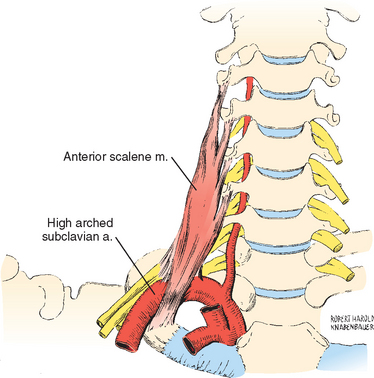
FIGURE 3-17 A type I upper brachial plexus anomaly has the anterior scalene fibers fused to the perineurium.
(Modified and reprinted with permission from the Society of Thoracic Surgeons: Wood VE, Ellison DW: Results of upper plexus thoracic outlet syndrome operation. Ann Thorac Surg 58:458-461, 1994.)
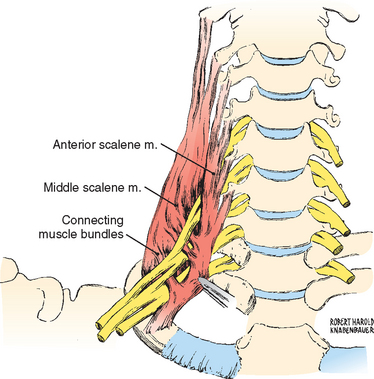
FIGURE 3-18 A type II upper plexus anomaly has connecting muscle bellies of the anterior and middle scalene.
(Modified and reprinted with permission from the Society of Thoracic Surgeons: Wood VE, Ellison DW: Results of upper plexus thoracic outlet syndrome operation. Ann Thorac Surg 58:458-461, 1994.)
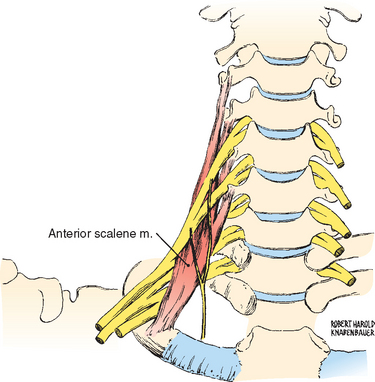
FIGURE 3-19 In a type III upper plexus anomaly, the anterior scalene muscle traverses the brachial plexus.
(Modified and reprinted with permission from the Society of Thoracic Surgeons: Wood VE, Ellison DW: Results of upper plexus thoracic outlet syndrome operation. Ann Thorac Surg 58:458-461, 1994.)
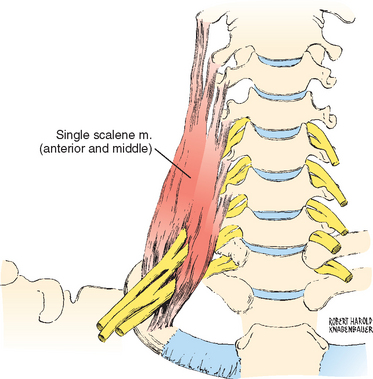
FIGURE 3-20 In a type IV upper plexus anomaly, the brachial plexus traverses the anterior scalene muscle.
(Modified and reprinted with permission from the Society of Thoracic Surgeons: Wood VE, Ellison DW: Results of upper plexus thoracic outlet syndrome operation. Ann Thorac Surg 58:458-461, 1994.)
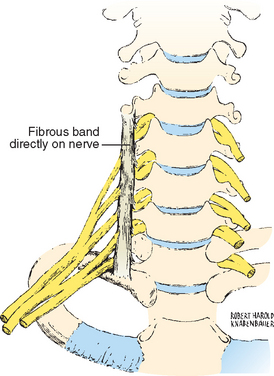
FIGURE 3-21 A type V upper plexus anomaly has a vertical fibrous band posterior to the anterior scalene muscle.
(Modified and reprinted with permission from the Society of Thoracic Surgeons: Wood VE, Ellison DW: Results of upper plexus thoracic outlet syndrome operation. Ann Thorac Surg 58:458-461, 1994.)
Posterior Deltoid Region
The infraspinatus muscle and the posterior head of the deltoid take their origin from the spine of the scapula and are covered by the trapezius muscle (Fig. 3-22). In the superior lateral region, the suprascapular nerve and artery enter into the infraspinous fossa through the spinoglenoid notch. Here, the suprascapular nerve supplies innervation to the infraspinatus muscle and the fibers of the shoulder joint capsule.
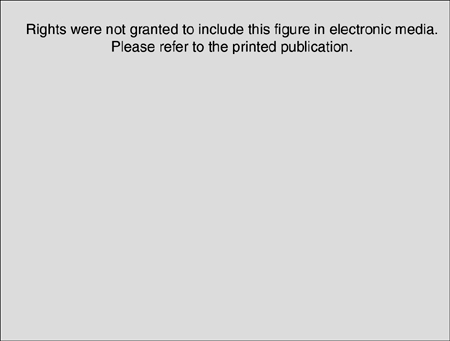
FIGURE 3-22 The posterior deltoid has several potential spaces containing neurovascular structures.
(Modified from DePalma AF: Surgery of the Shoulder, 3rd ed. Philadelphia: JB Lippincott, 1983, p 48.)
The quadrangular space is another important area of this region. It is formed by the humerus laterally, the long head of the triceps medially, the teres minor superiorly, and the teres major inferiorly. This space is traversed by the axillary nerve and the posterior circumflex humeral artery. Soon after it leaves the quadrangular space, the axillary nerve divides into anterior and posterior branches. The posterior branch innervates the teres minor and posterior deltoid muscles. The posterior branch continues laterally to become the lateral brachial cutaneous nerve, which innervates the lower posterior and lateral portion of the deltoid.
The anterior branch of the axillary nerve continues with the posterior circumflex humeral artery as they wind around the surgical neck of the humerus to reach the anterior aspect of the shoulder. The nerve diminishes in size as it progresses anteriorly and gives off numerous branches that travel vertically to enter the substance of the deltoid. Through the entire course, the axillary nerve runs 5 cm from the lateral edge of the acromion, thus providing a safe zone for the surgeon to operate. Burkhead and associates demonstrated considerable variation in this safe zone. They found the axillary nerve as close as 3.5 cm from the lateral acromion.21 Cetik and colleagues defined a safe area for surgical dissection as a quadrangular space with differing anterior and posterior lengths depending on arm length.22 The average distance of the axillary nerve from the anterior acromion was 6.08 cm (range, 5.2-6.9 cm), and the posterior acromion was 4.87 cm (range, 4.3-5.5 cm). Women and persons with small arms tend to have a smaller safe zone and need special consideration when planning for surgery.
Variations of the Suprascapular Notch and Suprascapular Ligament
The morphology of the suprascapular notch is a result of congenital and developmental changes in the area.23–25 Rengachary and colleagues described six types (Fig. 3-23)23–25:
Congenital duplication of the suprascapular ligament, which is typically manifested as bilateral suprascapular nerve palsy, has also been reported (Fig. 3-24).26 In a more recent cadaveric study of 79 shoulders by Ticker and colleagues, one bifid and one trifid suprascapular ligament was identified.27 Partial ossification of the suprascapular ligament has been described in 6% to 18% of cadaveric specimens.24,27,28 Complete ossification has been reported in 3.7 to 5% of specimens.24,27–29 A familial association to suprascapular ligament calcification has also been reported.30
Subacromial and Anterolateral Subdeltoid Region
Deltoid Muscle
The lateral head of the deltoid is formed from obliquely arranged fibers that arise in pennate fashion from either side of five or six tendinous bands whose proximal fibers are attached to the lateral acromion. In addition, three or four similar tendinous bands whose origins are from the deltoid tendon serve as the insertion point of the muscle fibers. This complex arrangement provides a powerful contraction that does not require a significant change in length, as would be needed with fibers arranged in parallel.
Acromion Process
In a cadaveric study, Bigliani and coworkers identified three types of acromial morphology, with types I, II, and III corresponding to flat, curved, or hooked, respectively, as viewed in sagittal cross section.31 They found an association between type III acromion morphology and rotator cuff tears (Fig. 3-25).
Nicholson and colleagues examined the morphology of the acromion in 402 shoulder specimens. They found a consistent distribution of type I (27%-37%), type II (33%-52%), and type III (17%-31%) acromion processes across age, gender, and race.32 However, a significant increase in spur formation was noted at the insertion site of the coracoacromial ligaments when specimens from cadavers younger than 50 years (7%) were compared with specimens older than 50 years (30%). Other authors have also noted this phenomenon.33–36
Glenohumeral Ligaments and Recesses
The fibrous capsule is a large redundant structure with twice the surface area of the humeral head. Inferiorly and posteriorly, the capsule is continuous with the labrum and adjacent bone (Fig. 3-26). Anteriorly, the capsule varies in relation to the labrum, the glenohumeral ligaments, and the synovial recesses.37 Three capsular thickenings are present in the anterior portion of the capsule and are named the inferior, middle, and superior glenohumeral ligaments. These structures act to reinforce the anterior capsule and serve as a static checkrein to external rotation of the humeral head. From the humeral head, they converge toward the anterior border of the labrum. The superior ligament blends with the superior portion of the labrum and the biceps tendon, whereas the middle and inferior ligaments blend with the labrum inferior to the superior ligament. In the region of the glenohumeral ligaments are the synovial recesses of the capsule.
DePalma’s study of 96 shoulders in 1950 revealed six variations in the relationship of the glenohumeral ligaments and the synovial recesses3:
Steinbeck and associates reported similar results in the dissection of 104 shoulders in 1998 (Fig. 3-27)38: type 1, 38.5%; type 2, 0%, type 3: 46.2%, type 4, 0.8%; type 5, 0%; type 6, 9.6%.
DePalma observed that the glenohumeral ligaments have a great amount of variation as well. The middle glenohumeral ligament was found to be a well-defined structure in 68% of specimens; it was poorly defined in 16% and absent in 12%.3 When present, it arises from the anterior portion of the labrum immediately below the superior ligament. In 4% to 5% of specimens it appears as a double structure. Furthermore, its width, length, and thickness vary considerably.
Steinbeck and colleagues also examined arrangements of the glenohumeral ligament complex in 104 shoulders.38 The superior glenohumeral ligament was again found to be the most consistent ligament in that it was present in 98.2% of specimens, although it was less than 2 mm thick in 28.8%. The middle glenohumeral ligament was present in 84.6% of specimens and, when present, consistently crossed the tendon of subscapularis. The inferior glenohumeral ligament was the most variable in existence and arrangement. It was a discrete structure in 72.1% of specimens and present only as a thickening of the capsule in 21.1%.
An anterosuperior foramen has also been identified between the glenoid and the labrum during arthroscopy.39,40 Williams reported a similar variant with an absent anterior superior labrum and the addition of a cord-like middle glenohumeral ligament that attaches to the superior labrum. Along with his coauthors, he named this arrangement the Buford complex (Fig. 3-28). In a review of 200 shoulder arthroscopy tapes, 1.5% had a Buford complex and 12% had a sublabral foramen.41 Of the patients with sublabral foramina, 75% had cord-like middle glenohumeral ligaments and 25% had normal glenohumeral ligaments. The authors warned against treatment of this lesion by attachment to the glenoid because of the possibility that postoperative range of motion would be restricted.
Glenoid Fossa and Labrum
Das and coworkers reported the anatomic finding of glenoid version in 50 shoulders. Their values ranged from 12 degrees of retroversion to 10 degrees of anteversion. The average version for the entire sample was 1.1 degrees of retroversion.42
Churchill and colleagues attempted the largest study to identify variations in size, inclination, and version of the glenoid by examining 344 human scapular bones.43 The size of the glenoid varied between sexes but not among races, with a mean female glenoid height of 32.6 ± 1.8 mm (range, 29.4-37.0 mm) and width of 23.0 ± 1.5 mm (range, 19.7-26.3 mm). Male glenoid size was reported as a mean height of 37.5 ± 2.2 mm (range, 30.4-42.6 mm) and width of 27.8 ± 1.6 mm (range, 24.3-32.5 mm). Iannotti and associates examined 140 fresh shoulders and recorded the average height of the glenoid to be 39.5 ± 3.5 mm (range, 30-48 mm) and the width to be 29 ± 3.2 mm (range, 21-35 mm).44 The average height of the donors in the study of Iannotti and colleagues was 181 cm, and 60% to 67% were male (cadaveric and living, respectively). The average height in the study of Churchill and associates was 173.0 cm for male patients and 161.3 cm for female patients. These differences might account for the variation in findings.
Churchill and coauthors reported version to be considerably different among races but not between genders. Black female subjects and black male subjects were found to have a transverse axis retroversion of 0.30 degrees (−6 to +6 degrees) and 0.11 degrees (−8.8 to +10.3 degrees), respectively. Glenoid retroversion in white female subjects averaged 2.16 degrees (−2.8 to +10.5 degrees), and in white male subjects the average was 2.87 degrees (range, −9.5 to +10.5 degrees). Churchill and associates also studied glenoid inclination and reported a wide variation in results (−7 to +15.8 degrees). Despite the variability in inclination, the majority of shoulders fell between 0 and 9.8 degrees of inclination.
Proximal Humerus
The massive humeral head lies beneath the coracoacromial arch and articulates with the glenoid of the scapula. The humeral head has 134 to 160 degrees of internal torsion as reported by DePalma, which corresponds to 44 to 70 degrees of retroversion.3,45–49 Edelson extensively studied the remains of fetal, child, and adult humeri in an attempt to elucidate the amount and development of humeral head retroversion. He defined retroversion as the angle subtended by the intercondylar axis of the elbow and a line bisecting the humeral head.50 Fetal retroversion averaged 78 degrees.51 The adult specimens averaged significantly less, with a range of −8 to +74 degrees; most, however, fell into the range of 25 to 35 degrees of retroversion. Edelson found that the change from fetal to adult retroversion of the humerus can be completed as early as 4.5 years and almost certainly by 11 years. In addition, he hypothesized that further torsion takes place after 11 years of age but occurs in the distal two thirds of the humerus.
Biceps Tendon
The biceps tendon has a partially intracapsular position. However, developmental anomalies sometimes occur. The tendon can be attached to a mesentery, can be entirely intracapsular, or can be absent.3 The tendon can also exist as a double structure.
The synovial lining of the glenohumeral capsule continues distally between the greater and lesser tuberosities. The synovial lining then reflects onto the tendon itself to form an important gliding mechanism within the bicipital groove. The motion of the tendon is minimal, but the amount of contact between the humerus and the tendon changes with arm position and thus does the function of the gliding mechanism.52–54 Contraction of the biceps under local anesthesia in the operating room produces no movement of the tendon, nor does movement of the arm through a full range of motion.
The bicipital groove has a great deal of anatomic variation. A supratubercle ridge can sometimes extend proximally from the superior aspect of the lesser tuberosity on the medial aspect of the groove (Fig. 3-29). DePalma’s study in 1950 found this structure to be well developed in 23.9% of specimens, moderately developed in 31.5%, and absent in 43.4%. Hitchcock and Bechtol observed this feature in 59 of 100 humeri.55 In Meyer’s series of 200 shoulders, the tubercle was present in 17.5% of specimens.56 It is postulated that a well-developed supratubercle ridge predisposes the biceps tendon to instability by potentially levering it out of the groove.56 A well-developed ridge can also increase the contact force between the biceps tendon and the transverse humeral ligament and thereby predispose to tendinitis.
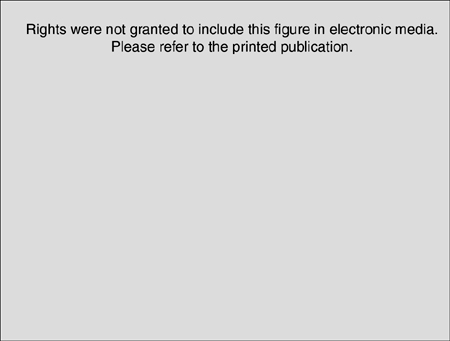
FIGURE 3-29 Schematic drawing of a supratubercular ridge that can facilitate displacement of the biceps tendon.
(From DePalma AF: Surgery of the Shoulder, 3rd ed. Philadelphia: JB Lippincott, 1983, p 54.)
The medial wall of the groove varies greatly in height, and such variation defines the obliquity of the groove. When a supratubercle ridge is present, the depth of the groove diminishes further. Hitchcock and Bechtol defined six variations of the angle of the medial wall of the bicipital groove (Fig. 3-30)55:
COMMON MALFORMATIONS OF THE SHOULDER
Cleidocranial Dysostosis
Cleidocranial dysostosis is a hereditary disorder that affects bones formed by intramembranous ossification.57–66 The skull, clavicles, ribs, teeth, and pubic symphysis are most commonly affected. Normal intelligence, an enlarged head, and forward-sloping shoulders are the typical clinical features (Fig. 3-31). If other malformations are associated with these findings, the condition is known as mutational dysostosis.59,65,67
The range of shoulder function in this condition is vast.68 Patients with this syndrome have been reported to work as heavy laborers without shoulder complaints, whereas others report glenohumeral instability.69 The outer third of the clavicle is the most commonly affected portion, and the medial aspect is usually normal.70,71 The middle part of the clavicle can also be absent, with spared medial and lateral portions.70 In either scenario, the missing portion of clavicle is replaced by fibrous tissue. Bilateral shoulder involvement is found in 82% to 90% of patients.71,72 Although uncommon, normal clavicles do not exclude this diagnosis.73 Two separate reports of families with this condition included members with normal clavicles.73,74
Associated Findings
Craniofacial
Frontal bossing and an enlarged forehead is common. The sutures of the skull remain unfused and are said to be metopic.75 An arched palate with multiple dental abnormalities is common, and consultation with a dentist is necessary. Eustachian tube dysfunction is common as well as both conductive and sensorineural hearing loss.76,77 Periodic audiologic testing is therefore recommended.
Pelvis, Hip, and Spine
Patients with cleidocranial dysostosis can have the pelvic manifestations of a widened symphysis pubis or sacroiliac joint.78 These abnormalities are not symptomatic, and their prevalence is therefore uncertain and their clinical relevance limited. Congenital coxa vara is reported to occur in 50% of patients.79 Neural tube defects can also be associated with this condition. Sacral agenesis, scoliosis, meningomyelocele, and vertebral body defects have all been reported.
Hands and Feet
The metacarpals and metatarsals can have both proximal and distal epiphyseal elongation. A resultant overgrowth of one or several metacarpals is common. Although the other bones of the hands and feet can be involved, the distal phalanx of the thumb and hallux are most greatly affected. The tarsal, carpal, and phalangeal bones may be small and irregular.
Inheritance
Approximately half of cases occur de novo, whereas the other 50% of patients have a family history. An autosomal dominant pattern with variable penetration is thought to be the responsible mechanism of transmission; thus, sexes are affected without predilection.4,65
Congenital Pseudarthrosis of the Clavicle
Easily mistaken for a fracture of the clavicle, this anomaly does not show evidence of callus formation (Fig. 3-32).72,80–83 The entities that need to be ruled out are cleidocranial dysostosis, neurofibromatosis, and trauma.84 No or minimal resulting loss of function is thought to occur.85 Overwhelmingly, the abnormality occurs unilaterally on the right side; however, left-sided and bilateral pseudarthrosis have been reported.86–95 A bump in the midcla-vicular region is palpable or grossly seen, and smooth ends are apparent on radiographs.96 Thoracic outlet syndrome has been reported to be secondary to the pseudarthrosis.97–101
Inheritance
A familial association has been reported, but a true pattern of genetic transmission has not been documented.102–105
Associated Abnormalities
Dextrocardia and the presence of cervical ribs have been associated with a left-sided pseudarthrosis.89,102 No other abnormalities are associated with this condition. If some are found, either cleidocranial dysostosis or neurofibromatosis needs to be investigated, and perhaps the patient should be referred to a geneticist.65
Treatment
Benign neglect has generally been accepted as treatment of this entity.106,107 Because of reports of thoracic outlet syndrome, some authors have proposed early excision and bone grafting in younger patients.80,90,91,99,108,109 Evidence that the risks of surgery outweigh the perceived benefits of intervention is sparse.110
Sprengel’s Deformity
The scapula is formed near the cervical spine in the developing fetus.40,111–113 Failure of the scapula to descend from its origin causes the scapula to appear small and high riding.114–120 The resulting deformity causes a wide range of disfigurement and dysfunction of the shoulder (Fig. 3-33). A fibrous connection between the cervical spine and the superior angle of the scapula is often present.121 When ossified, the connection is known as the omovertebral bone and is present in 20% to 40% of cases.40,122–124 The cause of this malformation is not known (Fig. 3-34).120,125–128
Cavendish extensively studied the appearance and dysfunction of 100 affected shoulders. He proposed a grading system to divide shoulders into groups by their appearance122:
In addition to elevation of the scapula, the scapula is misshapen and rotated. The glenoid is directed inferiorly, and the height of the scapula is reduced in comparison to the unaffected shoulder (Fig. 3-35). This phenomenon can make evaluation of the amount of elevation difficult. The inferior angle of the scapula is easily identified on radiographs, but because of the differences in the shape of the scapulae, elevation in comparison to the contralateral scapula is exaggerated. Likewise, if measurement is carried out to compare the height of the glenoids, the amount of elevation will be underestimated because of the rotatory deformity. Despite the theoretical disadvantage of measuring glenoid height, it seems to be the most accurate and reproducible method of evaluation.
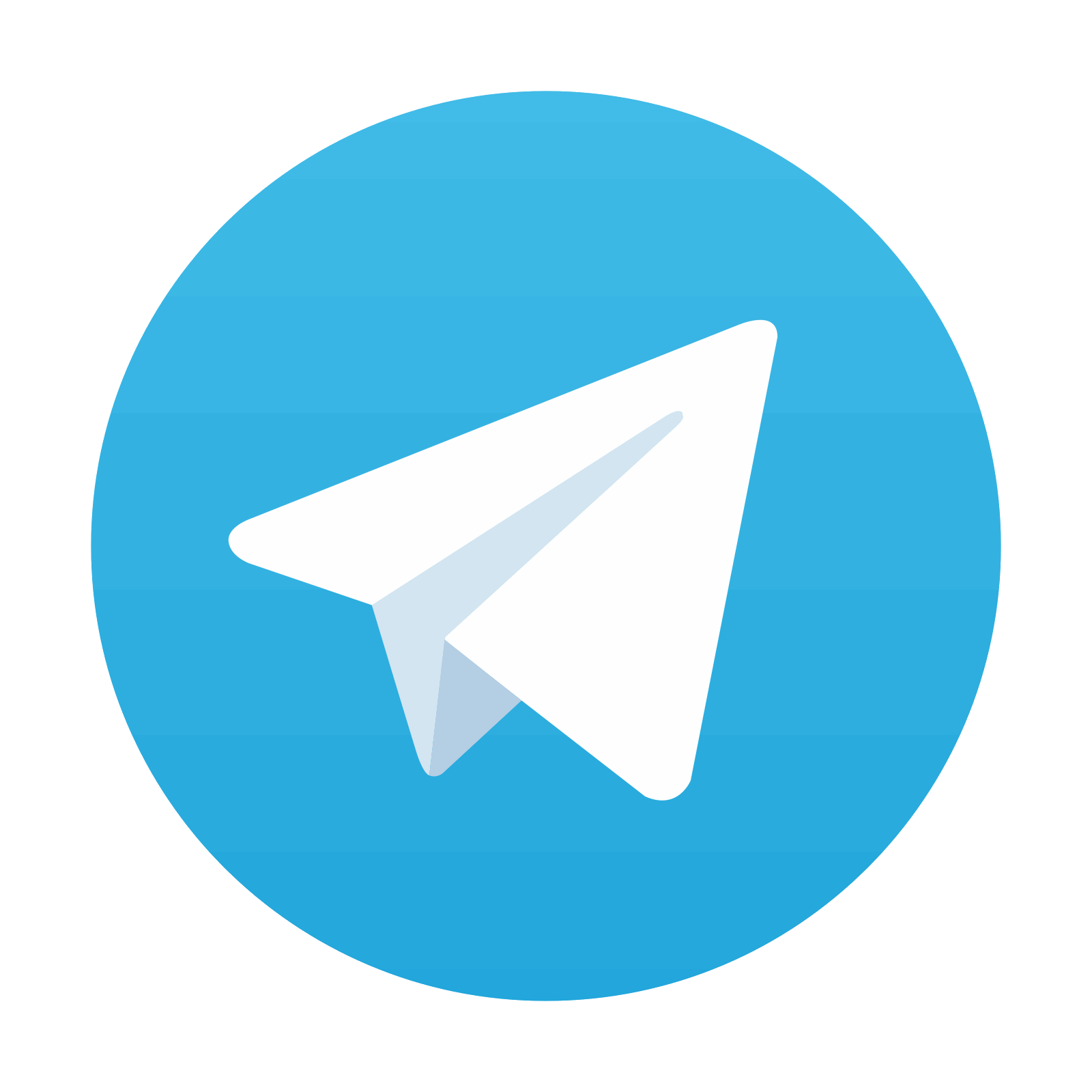
Stay updated, free articles. Join our Telegram channel

Full access? Get Clinical Tree
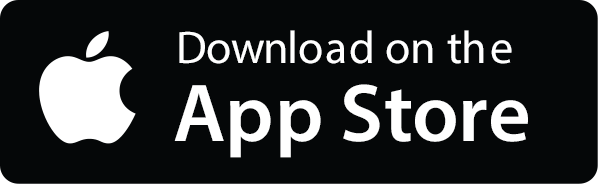
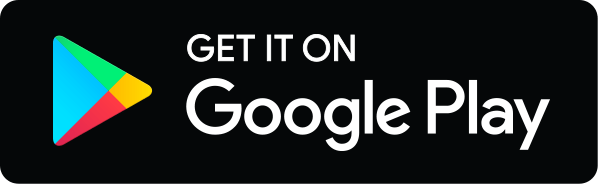