Fig. 1
Near-infrared motion capture camera (Vicon Motion Systems Ltd., Oxford, UK)
Kinetic analysis at a basic level can involve drawing a free body diagram to estimate two-dimensional forces during activity. These diagrams require information of the body’s anthropometrics in order to accurately estimate the forces acting on the segment. However, the calculations of these forces are limited to a static state of equilibrium and cannot be used for dynamic force estimates. A force transducer can be used to directly quantify a force and include devices such as a handheld dynamometer, which is commonly used in the clinical setting to measure muscle strength (Fig. 2). In the laboratory setting, a force plate is often used to measure three-dimensional forces during activity. The force plate data can be used for three-dimensional biomechanical analysis using an inverse dynamics approach by integrating the kinematic and kinetic data to calculate joint moments, powers, and energy in all three planes of motion.
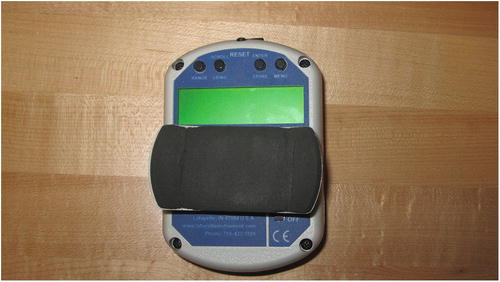
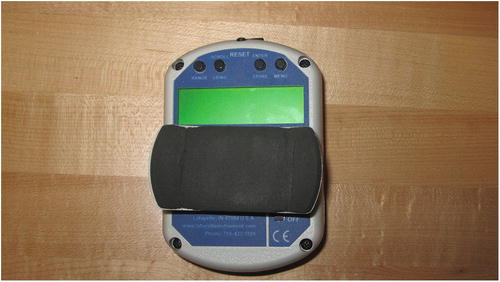
Fig. 2
Handheld dynamometer (Lafayette Instrument Company, Lafayette, IN)
Electromyography (EMG) and pressure sensors are also commonly used instruments to obtain information on muscle activation and pressure distribution patterns. EMG is a technique for recording and measuring electrical activity in the muscle, and the signal is a sum of all the recorded muscle fiber action potentials in the area of the recording electrode. Surface EMG involves the use of electrodes (Fig. 3) that are placed on the skin overlying the muscle of interest. Fine wire or intramuscular EMG involves the insertion of a small needle electrode or wires directly into the muscle fibers. Surface EMG measures a large sample of motor unit action potentials from the area under the electrodes, whereas fine wire EMG only records from a small number of motor units that are in close proximity to the electrode. There are many factors that can influence the signal recorded by EMG such as skin impedance, muscle fiber depth, adipose tissue, movement artifact, and muscle length; therefore, caution should be taken when interpreting the results. It also should be acknowledged that EMG signal is only directly proportional to muscle force output during an isometric contraction when muscle length does not change. Dynamic activities such as jumping or running cause a change in the number of motor units that are positioned under the electrode; therefore, a consistent measure from the same motor units is not being recorded throughout the movement which can influence the signal output. EMG data can also be used with advanced muscle measurement techniques such as musculoskeletal modeling which can provide a more accurate estimate of muscle forces during activity, although the use of such techniques requires a greater computational background and an understanding of programming to create a valid model.
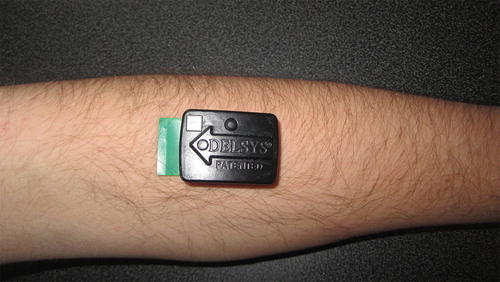
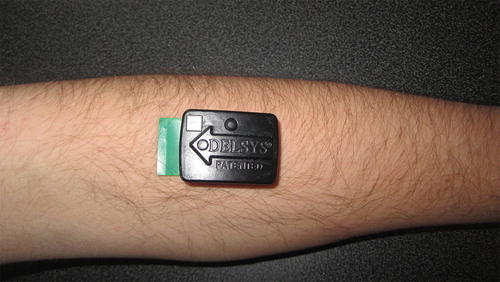
Fig. 3
Wireless EMG sensor (Delsys Inc., Natick, MA)
Biomechanical Analysis of the Hip Joint
Early biomechanical investigations of the hip joint performed by Rydell described the complex structural anatomy of the femur as it pertains to accommodating load demand during weight bearing [5]. Crowninshield [6] performed a kinematic and kinetic analysis of the hip during the activities of walking, stair negotiation, and moving from sitting to standing. These seminal investigations of hip biomechanics provide the foundation for further investigation of hip biomechanics during functional activity in both the healthy and pathologic populations. Kinematic investigation in patients with hip osteoarthritis revealed an overall loss of hip range of motion during gait. Additionally patients with the greatest amount of motion loss and pain exhibited a kinematic alteration characterized by a “reversal” of sagittal plane motion as the hip was moved into extension [7]. Similarly this sagittal plane kinematic “reversal” was observed in patients with symptomatic femoroacetabular impingement (FAI) during the stance phase of gait [8]. Kennedy et al. [9] found that patients with symptomatic FAI demonstrate less hip abduction and pelvic frontal plane motion compared to controls during the swing phase of walking. Patients with FAI also demonstrate a reduced squat depth compared to controls during a maximal squat task [10]. These findings support the use of a maximal squat test during the evaluation of patients with symptomatic FAI [11].
Hip pathology has also been shown to cause kinetic alterations at the hip joint. Often structural abnormality associated with developmental deformity or degenerative osteoarthritis can have profound effect on force production and transmission at the hip joint. These structural abnormalities can lead to alterations of the hip joint axis of rotation which has been shown to have profound impact on the hip muscles moment arm length, muscle force production, and muscle moments produced at the hip joint [12]. An instrumented femoral prosthesis following a total hip replacement has been used to quantify in vivo contact forces during daily activities such as walking and stair climbing [13]. The hip joint contact forces during level walking were found to be approximately 1.5 times body weight and 2.5 times body weight for stair climbing [13]. Hip joint forces during common open chain hip exercises were also quantified using musculoskeletal modeling based on kinematic, kinetic, and EMG data from healthy individuals. This model simulated conditions of weakness in particular muscle groups and showed that a significant increase in force in the anterior hip occurs in the presence of hip extensor weakness [14]. These findings have implications for rehabilitation specialists when prescribing exercises for patients with anterior hip injury such as an acetabular labral tear. FAI has also been shown to alter kinetics at the hip joint during walking with a reduction of external moments for hip flexion and external rotation in symptomatic patients [15].
Muscle activation patterns may influence the kinetics at the hip joint, and changes in these patterns have been observed in patients with hip pathology such as osteoarthritis and FAI [16, 17]. A recent systematic review of hip muscle weakness in patients with OA revealed consistent evidence of reductions in muscle force output and fiber atrophy [16]. Similar findings were observed in patients with FAI where isometric muscle torque was reduced for hip adduction, flexion, external rotation, and abduction compared to healthy controls [17]. In addition to pathology, externally imparted forces change the muscle activation patterns at the hip. Neumann et al. [18, 19] found that changes in carrying load position result in changes in muscle activity of the gluteus medius muscle. These findings have practical significance for patient education and treatment interventions. Carrying a load at the side results in a greater amount of EMG activity of the ipsilateral gluteus medius muscle during walking [18]. Conversely, individuals who used an assistive device on the contralateral side demonstrated a reduction in gluteus medius activity compared to walking without an assistive device [20]. The basic biomechanical findings provide the rationale for physicians and rehabilitation specialists in instructing patients with hip pathology to use an assistive device such as a cane on the side contralateral the injury [18].
Hip pathomorphology is an abnormality in the three-dimensional geometry of the hip, whereas hip pathomechanics involve the understanding of how motion conflicts produce chondrolabral injury. Ganz and colleagues published the landmark paper describing the concept of femoroacetabular impingement (FAI) as a possible cause of end-stage hip osteoarthritis [21]. FAI consists of morphological pathology involving abutment of the femoral neck and the acetabular rim at the extremes of range of motion. Deformities can be primarily related to the acetabulum (pincer), femoral neck (CAM), or most commonly a combination of the two. FAI and other pathomorphologic hip deformities (i.e., dysplasia) can lead to labral injury, osseous change, and eventually osteoarthritis. Surgical correction of the deformities on the acetabulum, proximal femur, or both was originally described using an open surgical hip dislocation or osteotomies. More recently, hip arthroscopy has been widely utilized as a technique to correct the pathomorphology associated with FAI (Fig. 4).
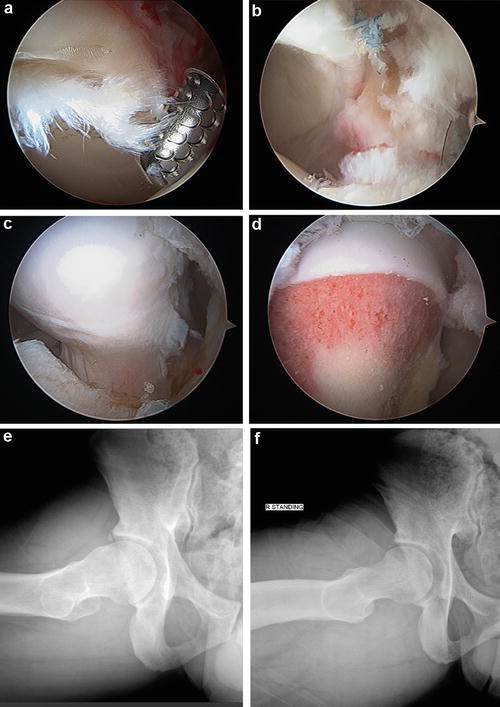
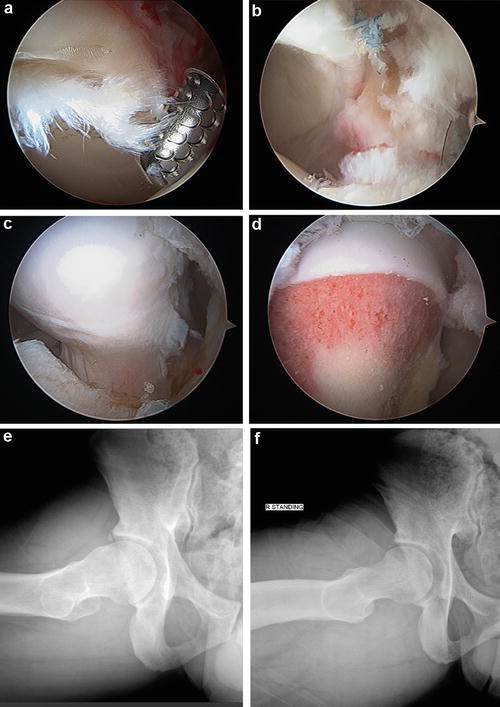
Fig. 4
Hip arthroscopy to treat chondrolabral injury and FAI. (a) Injury at the chondrolabral junction seen with CAM deformity. (b) Arthroscopic appearance after labral refixation and chondral debridement. (c) CAM deformity at the proximal femoral head-neck junction. (d) View of the proximal femoral head-neck junction after osteochondroplasty. (e) Plain radiograph using the Dunn lateral view of the CAM deformity prior to surgery. (f) Plain radiograph using the Dunn lateral view of the proximal femur after osteochondroplasty
There has been recent interest in the characterization of hip pathomorphology using three-dimensional imaging to assist identification of deformities and guide surgical treatment. Computer tomography (CT) scans allow for the precise evaluation of the three-dimensional morphology of the hip joint. Kang and colleagues have described a novel, automated method to describe aberrancies in the topography of the proximal femur and acetabulum [22]. The quantification of CAM and pincer deformities is critical for a more precise understanding of hip motion conflicts (Figs. 5 and 6). In addition, three-dimensional modeling is another technique to create a virtual hip model to identify areas of impingement with hip movement. More advanced three-dimensional imaging and dynamic imaging may provide surgeons information about the exact amount of osseous resection of the acetabulum and proximal femur to normalize the anatomy and eliminate motion conflict.
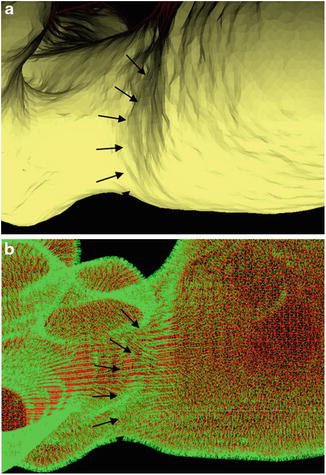
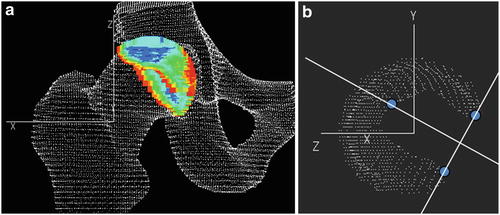
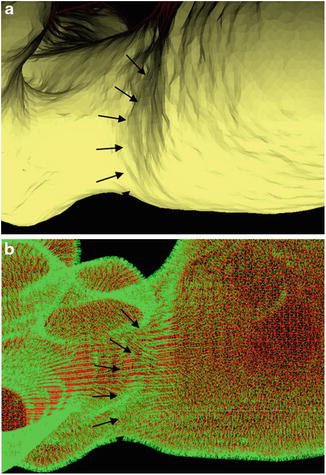
Fig. 5
Quantification of CAM deformity. (a) CAM lesion in the femoral neck appeared on the 3D polygon model (arrows). (b) Normal vectors of the polygons (green lines). The CAM lesion is shown by arrows
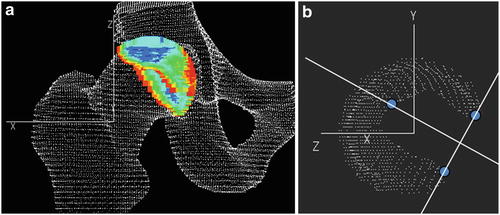
Fig. 6
In vivo zoning system based on the acetabulum. (a) Automatic segmentation of the acetabulum point clouds using the least distances in the hip joint. (b) Determination of anatomical landmarks to define acetabulum reference lines
Controlled laboratory biomechanical investigation has established our foundational understanding of the biomechanics of the hip in the healthy and pathologic populations; however, the methods applied in these studies are often beyond the capability of the clinical setting. Therefore, it is essential that orthopedic clinicians have a manner in which to evaluate the biomechanics of a patient in order to assist in the clinical decision making process. Visual observation of movement patterns during common activities can provide practical information on the biomechanical implications of hip pathology . Evaluation of hip osteokinematics, arthrokinematics, and muscle activation can provide information about the movement and alteration in loading patterns that are associated with a certain injury. Additionally, this information provides a baseline comparison to assess improvement following a treatment intervention. Gait analysis is commonly performed in orthopedic practice as this activity is one of the most basic activities of daily living. Understanding the biomechanics of the gait cycle can assist clinicians in diagnosis and treatment of hip injury through the observation of deviations that are often associated with a particular pathology. The following sections of this chapter will discuss the clinical biomechanical analysis of the hip joint through the discussion of normal hip osteokinematics, arthrokinematics, and muscle actions in each of the planes of motion. Additionally, biomechanical analysis of the hip joint during normal gait will be discussed, and gait and deviations associated with hip pathology will be presented.
Osteokinematics, Arthrokinematics, and Muscle Actions of the Hip Joint
The ball and socket structure of the hip joint allows for six degrees of freedom around three axes of motion at the hip joint center. Although the hip is a relatively mobile joint, it is also incredibly stable because of its osseous architecture, joint articulation, and extensive surrounding soft tissue structures. Open chain activity of the hip joint is characterized by femoral-on-pelvic motion, whereas closed chain function often results in pelvic-on-femoral motion [23]. Femoral-on-pelvic movement will be referred to as open chain and pelvic-on-femoral movement as closed chain for the remainder of this discussion.
The movements and axis of rotation of the hip joint in each motion plane are listed in Table 1. Osteokinematics describe the motion of a body segment and in the case of the hip joint refers to motion of the femur or pelvis. Arthrokinematics refers to the movement that occurs between the two joint surfaces and is the motion occurring between the femoral head and acetabulum in the case of the hip joint. The arthrokinematics of the hip joint abide by the principles of the convex on concave and concave on convex rules [23]. These principles state that when a convex surface (i.e., femoral head) moves on a concave surface (i.e., acetabulum), the motion between the joint surfaces occurs in the opposite directions. Conversely, when the concave surface of the acetabulum moves on a convex surface of the femoral head, the motions at the joint will occur in the same direction (i.e., closed chain hip motion).
Table 1
Planes of motion, anatomical direction, axis of rotation, and muscle actions of the hip joint
Plane of motion | Movement | Axis of rotation | Muscles |
---|---|---|---|
Sagittal | Flexion and extension | Mediolateral | Flexors (iliopsoas, rectus femoris, sartorius, adductor longus) |
Extensors (gluteus maximus, adductor magnus; hamstrings) | |||
Frontal | Abduction and adduction | Anterior-posterior | Adductors (adductor longus, brevis, and magnus, obturator externus, pectineus) |
Abductors (tensor fascia lata, gluteus medius and minimus, sartorius) | |||
Transverse | Internal and external rotation | Longitudinal axis | External rotators (piriformis, gluteus maximus, gemelli superior and inferior, obturator internus, quadratus femoris) |
The hip consists of 25 muscles that cross the joint; therefore, the influence of these muscle actions on joint mechanics is profound [12]. Additionally, a number of the muscles span two joints and, therefore, influence multiple joints during activation. The muscle actions at the hip will be discussed in the context of the direction of force produced to create movement. Although these muscle actions involve angular and linear force production, the discussion on the quantification of these forces involves advanced biomechanical analysis and is beyond the scope of this chapter. The following sections will describe the osteokinematics, arthrokinematics, and muscle actions for the hip in each of the three planes of motion.
Sagittal Plane Osteokinematics
The normal osteokinematics of the hip joint in the sagittal plane for open chain flexion is approximately 120–125° with the knee in a flexed position but reduces to between 70° and 80° when the knee is in an extended position [23, 24]. During closed chain function, hip flexion corresponds to an anterior tilt of the pelvis which results in approximation of the anterior pelvis and the anterior aspect of the femoral neck and shaft. Motion in this plane has profound implication in hip pathology as hip flexion loss is associated with conditions such as osteoarthritis and femoroacetabular impingement [21, 25]. The normal value for open chain hip extension is between 10° and 15° [24]. A posterior pelvic tilt in the closed chain creates extension motion at the hip joint and impact gait in the presence of hip flexion contracture or intraarticular injury [7]. Closed chain motion at the hip joint has a direct consequence on the movement of the lumbar spine because of the strong attachment of the sacrum to the pelvis through the posterior pelvic ligaments and trunk extensor muscles [23]. The link that the hip joint provides between the trunk and lower extremities has profound implications for the diagnosis and treatment of low back and lower extremity kinetic chain injury.
Sagittal Plane Arthrokinematics
The bony structure and soft tissue support of the hip joint minimize translational motion of the femoral head on the acetabulum [23]. The motion between the femur and acetabulum during the motions of hip flexion and extension occurs as a pure rotation around a medial lateral axis that passes through the center of the femoral head. Open chain hip flexion causes a spin of the femoral head in the posterior, while an anterior pelvic tilt will cause an anteriorly directed rotation of both the femoral head and acetabulum. These motions occur in the opposite direction for open chain hip extension and a posterior pelvic tilt.
Sagittal Plane Muscle Actions
In order for a muscle to create motion at the hip in the sagittal plane, the line of pull from the muscle must lie either anterior or posterior to the joint axis of rotation [12]. Muscles that create action on the anterior side of the axis of rotation will result in the motion of flexion at the hip, while muscles that lie posterior to this axis cause hip extension motion. This information is important to clinicians because the understanding of the line of pull of a muscle in relation to the joints axis of rotation can provide a general idea of the direction of force at the joint during motion. This information can also be used to assist with muscle strengthening by changing joint positions to influence moment arm lengths and muscle force production [12].
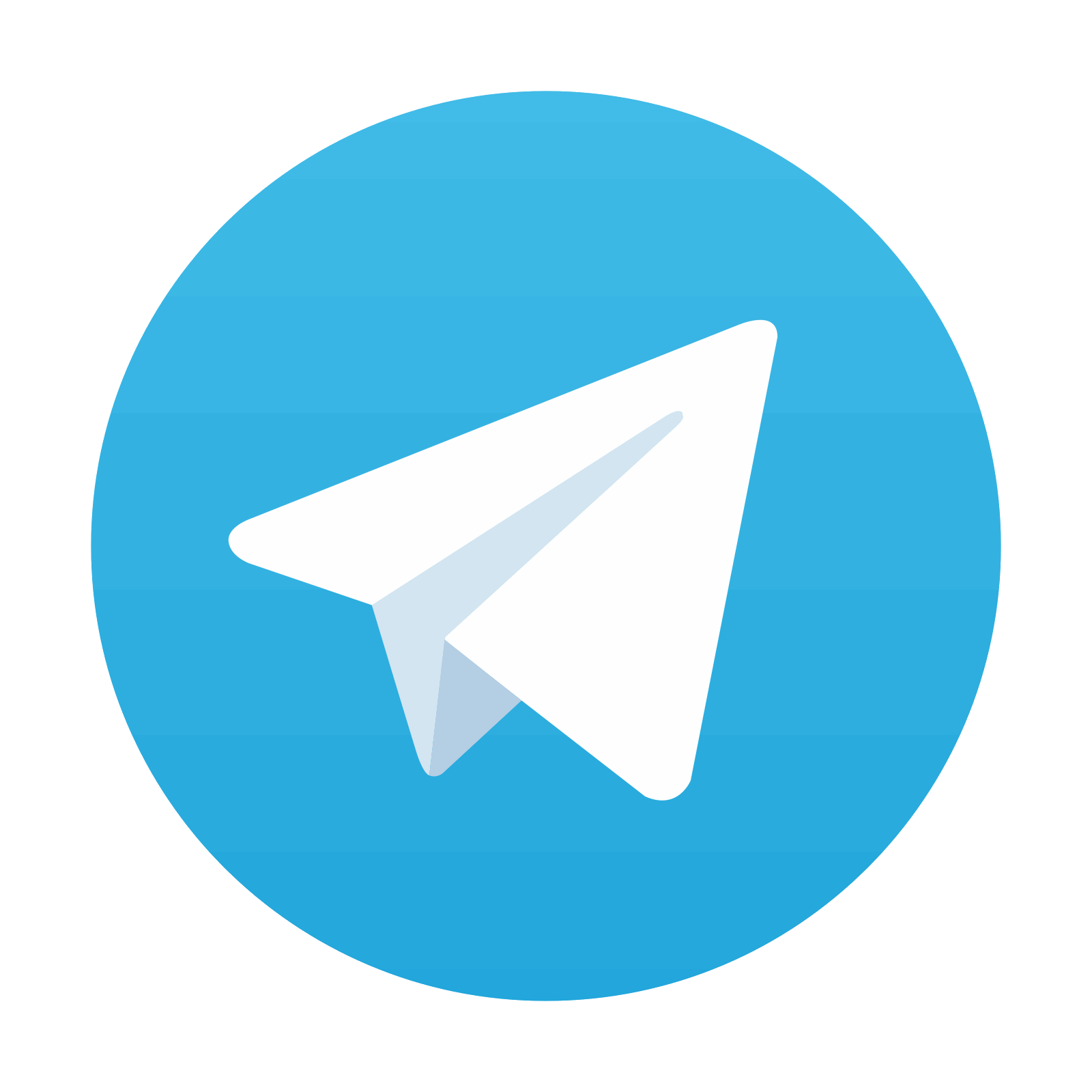
Stay updated, free articles. Join our Telegram channel

Full access? Get Clinical Tree
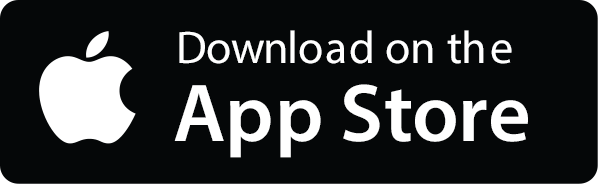
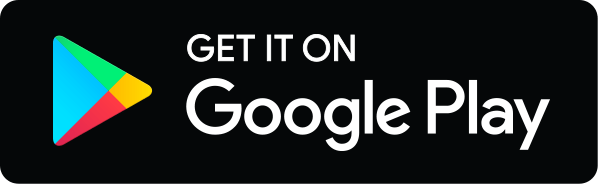