CHAPTER 28 Cervical, Thoracic, and Lumbar Spinal Trauma of the Immature Spine
Epidemiology
Spine injuries involve 1% to 5% of children admitted to trauma centers, making these injuries relatively uncommon in pediatric patients.1,2 The true incidence may be higher given the challenges of obtaining a reliable examination and necessary images in children compared with adults.1–3 A high index of suspicion is warranted in children with polytrauma and children who have sustained a head injury; 40% to 50% of children with a head injury also have a cervical spine injury.3,4
The level of spine involvement and mechanism vary with age. The cervical spine is most commonly involved in all pediatric patients; children younger than 8 years of age are more susceptible to upper cervical spine injuries, and children older than 8 more commonly sustain subaxial cervical spine injuries.5 Thoracic and lumbar spine injuries also increase in incidence as children age and the spine takes on the biomechanical characteristics of an adult.5
Traffic-related incidents account for nearly one third of all pediatric spine injuries; however, other mechanisms of injury are unique to particular age groups.5 In neonates, birth trauma is the most common cause of spine trauma with an incidence of 1 in 60,000 live births.6 Excessive distraction or hyperextension of the cervical spine is thought to be the mechanism, occurring in breach deliveries and large neonates.7 Spinal cord injury should always be suspected in neonates with hypotonia or cardiopulmonary instability. Injuries in infants and young children should raise suspicion for nonaccidental trauma. Imaging studies show avulsion fractures of the spinous processes or multilevel compression fractures in conjunction with rib fractures, long bone fractures, cutaneous lesions, and other characteristic injuries of abuse.8,9 In older children and adolescents, sports-related injuries and diving accidents occur more frequently.5 Recognizing these unique mechanisms of injury helps to raise the suspicion of a spine injury for the treating clinician.
Developmental Anatomy and Biomechanics
Understanding the developmental anatomy allows identification of normal radiographic variants and synchondroses versus true pathology. The atlas and axis undergo unique development, whereas the subaxial cervical spine, thoracic spine, and lumbar spine follow a similar pattern of maturation. The atlas develops from three primary centers of ossification: the anterior arch and two neural arches.10 The two neural arches are visible at birth and develop into the lateral masses. The anterior arch is radiographically visible at birth in only 20% of infants, and in the remaining infants it ossifies over the subsequent year. Measuring the atlanto-dens interval (ADI) is unreliable in infants younger than 1 year old. The synchondrosis between the two neural arches located posteriorly closes by age 3. Ossification between the anterior arch and the two neural arches occurs by age 7.11
The axis (C2) is formed by five primary centers of ossification. The odontoid process is formed by two parallel ossification centers that fuse in utero during the 7th fetal month. A secondary ossification center occurs at the tip of the odontoid, termed the os terminale, arising between ages 3 and 6 years and fusing by age 12 years. The remaining primary centers of ossification are the body and two neural arches. The body typically fuses with the odontoid by age 6 years, but this synchondrosis can persist until age 11. The neural arches fuse anterior to the body by age 6 and posterior by age 3, similar to the atlas. Fractures can occur through the synchondrosis of the odontoid peg, recognized by soft tissue swelling and asymmetrical gapping of this synchondrosis with associated tilting of the dens.12
The subaxial cervical spine (C3-7), thoracic spine, and lumbar spine all develop in a similar fashion. There are three primary ossification centers: the two neural arches and the body. The neural arches fuse to the body anteriorly by age 6 and posteriorly by age 3. Secondary centers of ossification can exist at the tips of the transverse processes, spinous process, and superior and inferior aspects of the vertebral body. These areas ossify in early adulthood and can be mistaken for fracture.10,11 The vertebral bodies of the subaxial cervical spine first ossify posteriorly with progression anteriorly as the child ages. This process replaces a cartilage scaffold with bone, and the vertebral body takes on its characteristic rectangular shape by age 7. Until age 7, it is acceptable to have anterior wedging of the subaxial cervical vertebrae, and this should not be confused with anterior compression fractures. This normal wedging can be profound at C3.
Children with a spine injury are typically divided by age: younger than or older than 8 years old. The biomechanics and injury patterns seen in children younger than 8 years differ from the patterns seen in children older than 8. The spine typically assumes adult characteristics and size by age 8 to 10 years. From birth until age 8, children are usually more susceptible to upper cervical spine injuries. In addition, it is more common for children in this younger age group to have a neurologic injury and subluxation or complete dislocation rather than a fracture.2,13–16 Proposed reasons for the increased incidence of upper cervical spine injuries in this younger age group are listed in Table 28–1. These unique characteristics are important to keep in mind when transporting and evaluating a young child.
TABLE 28–1 Risk Factors in Children Younger than 8 Years Old for Developing Upper Cervical Spine Injuries
Transport and Evaluation
Herzenberg and colleagues17 were the first to note that the transport of young children (<8 years old) on a standard adult spine board tended to cause excessive flexion of the cervical spine. It was noted in all cases that the cervical spine was forced into relative kyphosis because of the disproportionately large head relative to the chest. This flexed position could potentially jeopardize the cervical cord if the mechanism of injury was a flexion force, which is often the case in motor vehicle accidents. To obtain a neutral position, Herzenberg and colleagues17 recommended pediatric spine boards with a cutout for the occiput. Alternatively, a standard spine board can be used with a towel roll placed under the shoulders allowing the head to drop into mild extension, as shown in Figure 28–1.
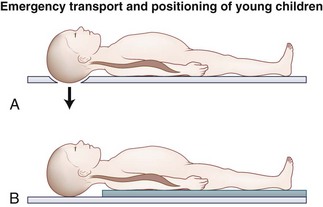
FIGURE 28–1 Emergency transport and positioning of young children.
(From Herzenberg JE, Hensinger RN, Dedrick DK, et al: Emergency transport and position of young children who have an injury of the cervical spine: The standard backboard may be hazardous. J Bone Joint Surg Am 71:15-22, 1989.)
In a subsequent study, Curran and colleagues18 prospectively evaluated these modified transportation methods for achieving neutral alignment of the cervical spine. They obtained supine lateral radiographs of the cervical spine in 118 pediatric trauma patients and measured the sagittal alignment using the Cobb method. They determined that only 60% of the patients were within 5 degrees of neutral alignment using this method. This study highlights the point that very young children may need even more relative chest elevation and as the child approaches age 8 this decreases. This point was confirmed by Nypaver and Treloar,19 who evaluated the height of back elevation needed in children younger than 4 years versus children older than 4 to achieve neutral cervical spine alignment. Children younger than four required 5 mm additional elevation.
Although it is unknown how alignment during transport affects outcome, the authors follow the recommendations of Herzenberg and colleagues17 regarding spine board immobilization, keeping in mind that very young children may need additional elevation to achieve neutral alignment. A clinical guideline is to evaluate the child from the side after immobilization, aligning the external auditory meatus with the shoulder. A well-fitting cervical collar should be placed, transport should occur on a spine board with a cutout for the occiput or alternatively elevating the shoulders, sand bags should be placed on either side of the head to prevent rotation, and the head should be taped to the spine board.
Clinical evaluation of a child thought to have an injury to the spine is often hampered by an inability to obtain an accurate history and a thorough physical examination. The clinician should have a heightened suspicion of spine injury in nonverbal children, owing to a 23-fold increased likelihood of missing a cervical spine injury.20 The mechanism of injury should be taken into account when considering the likelihood of an occult spine injury. Additionally, the presence of associated injuries, including facial trauma, head injuries, thoracic wall injuries, and abdominal injuries, increases the likelihood of spine trauma. Abdominal injuries, particularly injuries of the small bowel, are associated with flexion distraction injuries of the thoracolumbar spine.21 Spinal injuries can occur at multiple levels with noncontiguous fractures occurring in 16%.22
Plain Radiography of Cervical Spine
Imaging of the spine should be done if a child meets any of the following criteria: The child is nonverbal secondary to age or altered mental status, intoxication is present, a neurologic deficit exists or there is a history of a transient deficit, neck pain is present, there is a history of a high-risk mechanism of injury, physical signs of neck trauma or lap belt trauma are present, or painful distracting injuries are present.23 Additionally, unexplained cardiorespiratory instability can be an indication of a high cervical spine injury and should be evaluated appropriately.
The three-view cervical spine series may not be as applicable in pediatric patients with polytrauma, but likely still has a role in patients without polytrauma. The supine lateral cervical radiograph has a reported sensitivity of 79% to 85% in pediatric patients.24 This sensitivity is dependent on seeing all seven cervical vertebrae, including the occipitocervical and cervicothoracic junction. Lally and colleagues25 found that all seven cervical vertebrae were seen in only 57% of children on the initial cervical spine series. The addition of the anteroposterior and open-mouth odontoid views increases the sensitivity to approximately 94% if ideal images can be obtained, which can be extremely challenging in an uncooperative child.26 Buhs and colleagues27 showed that the open-mouth odontoid view did not provide additional information in children younger than 9 that was not already appreciated on the anteroposterior and lateral views. In its place, these authors recommended use of computed tomography (CT) from the occiput to C2.
The use of flexion and extension radiographs during the initial evaluation has come into question. Ralston and colleagues28 disputed the need for these dynamic studies in the acute setting. These investigators had blinded radiologists retrospectively review static and flexion-extension radiographs on 129 children. They found that if the static x-rays were normal, the flexion-extension views would reveal no abnormality. Dwek and Chung29 confirmed these findings, retrospectively evaluating 247 children in whom static and flexion-extension radiographs had been obtained in the trauma setting. They found the flexion-extension view helpful in ruling out an injury in only four patients who were originally thought to have abnormal static films. These dynamic studies are helpful in evaluating for instability after an appropriate time of closed treatment. In the delayed setting, flexion-extension radiographs can confirm either healing and resultant stability or the need for operative treatment.
Interpretation of the cervical spine series requires an understanding of the normal anatomy and the anatomic variants of the immature spine that can mimic trauma. Special attention should be given to the upper cervical spine including the atlantoaxial and atlanto-occipital region given the propensity of injuries to this area in children and the subtle radiographic findings.30 Several methods for evaluating the craniocervical junction using the lateral x-ray have been described. The “rule of 12’s” is thought to be the most sensitive method, whereby the distance between the basion and tip of the odontoid process should be 12 mm or less, and a line drawn parallel along the posterior aspect of the body of C2 including the odontoid should come within 12 mm of the basion. The distance from the tip of the odontoid to the basion is unreliable in children younger than 13 because of incomplete ossification of the odontoid; however, the line drawn parallel to the back of the C2 body and odontoid peg should be less than 12 mm from the basion.22 A gap of more than 5 mm from the occipital condyle to the C1 facet also represents a disruption of the occipitocervical junction.31
The atlantoaxial articulation is best evaluated with the ADI. In children, the normal ADI can be up to 5 mm. If the ADI exceeds 5 mm on lateral flexion and 4 mm on lateral extension, the transverse atlantal ligament is likely disrupted.32 When the ADI exceeds 10 to 12 mm, the alar and apical ligaments have failed with a high risk of cord compression.33 The extent of cord compression at the atlantoaxial joint can be determined using Steel’s rule of thirds,34 which is based on dividing the inner diameter of the C1 ring into thirds. One third of the space should be occupied by the odontoid; one third, by the spinal cord; and one third, by the space available for the cord. When the dens migrates posteriorly by greater than one third of this space, the transverse ligament has been disrupted, and the spinal cord is likely being compressed.
Swischuk35 described the posterior cervical line (spinolaminar line) to help diagnose pathologic angulation and translation in the upper cervical spine. The posterior cervical line is formed by drawing a line from the anterior aspect of the spinous process of C1 to the anterior aspect of the spinous process of C3. The line should pass within 1.5 mm of the anterior aspect of the spinous process of C2 on flexion-extension radiographs; otherwise, a true injury should be suspected.
Many normal anatomic variants should be recognized so as to not be confused with a “true” traumatic injury. A normal finding on extension radiographs in 20% of children is overriding of the anterior arch of the atlas on the odontoid. This finding is incomplete ossification of the dens.36 Another common variant noted on cervical spine x-rays in an immature spine is pseudosubluxation of C2 on C3 and less commonly C3 on C4. Cattell and Filtzer36 were the first to appreciate this normal finding in a study involving 160 pediatric patients with no history of cervical spine trauma. On dynamic radiographs, 46% of children younger than 8 years showed 3 mm of anteroposterior motion of C2 on C3; 14% of children had radiographic pseudosubluxation of C3 on C4.36,37 Based on this study and others, 4 mm of translation is considered normal.6,28,36,38
The absence of cervical lordosis on static lateral x-rays can be a normal finding in 14% of children up to age 16.22 This normal variant can be differentiated from a more ominous sign of posterior ligamentous injury by assessing the posterior intraspinous distance. Each intraspinous distance should not be more than 1.5 times greater than the intraspinous distance directly above and below a given level. The only exception to this rule is the C1-2 intraspinous distance, which can be greater than 1.5 times the distance of the level below. This normal increased mobility is thought to be secondary to the stout posterior ligaments linking C1 to the occiput.39,40
Another challenge unique to the developing spine is differentiating a synchondrosis that has not yet ossified from a true fracture. A synchondrosis typically shows well-corticated sclerotic margins. Another helpful aid in differentiating a subtle fracture from a synchondrosis is evaluation of the prevertebral soft tissues. The retropharyngeal space (at C2) should be less than 7 mm and the retrotracheal space (at C6) should be less than 14 mm in children. In simplistic terms, the retropharyngeal space should be one half the anteroposterior distance of a cervical vertebral body, and the retrotracheal space can be up to a full cervical vertebral body. The retropharyngeal soft tissue can be falsely increased with expiration, such as in a crying child.41 The lateral cervical spine x-ray should be repeated, attempting to obtain the radiograph during inspiration, to determine if a true abnormality exists.
Plain Radiography of Thoracic Spine
Burst fractures are best seen on a CT scan; subtle findings on conventional radiography include interpedicular widening on the anteroposterior projection and small cortical defects at the posterosuperior corner of the vertebral body on the lateral projection. Improper radiographs are the leading cause of missed injury and subsequent neurologic deterioration in large series of trauma patients, supporting the argument for increased use of CT.42,43
Computed Tomography and Magnetic Resonance Imaging of Spine
The use of helical CT has replaced conventional radiography as the screening tool of choice for the adult cervical spine in the setting of blunt trauma.44,45 One concern in children is the exposure to radiation and potential risk of developing thyroid cancer. Some estimates have been four times the radiation dose in the helical CT group compared with conventional radiographs.46,47 CT scan with reconstructions should be used for the correct indications.
There is growing evidence that CT is superior to plain radiography for evaluating the thoracolumbar spine in adults. This evidence has relevance in patients with polytrauma who are being evaluated with a traumagram. These studies, originally targeted for evaluation of the thoracic and abdominal contents, have been shown to be very sensitive at screening for thoracolumbar spine injury. Multiple prospective studies have shown a higher sensitivity for CT (93% to 100%) compared with plain radiography (33% to 74%) with improved interobserver variability.37,48–50 This information can likely be extrapolated to pediatric patients, but further studies need to be performed before CT scans with sagittal and coronal reconstructions replace conventional radiography for thoracolumbar spine clearance.
Magnetic resonance imaging (MRI) is useful for evaluating a neurologic deficit and soft tissue involvement, to help with preoperative planning, and to assist with cervical spine clearance in an obtunded patient. MRI in this age group can be difficult because these young patients often need sedation to prevent movement during data acquisition. MRI should be obtained in patients with evidence of a neurologic deficit, and some investigators would argue that it should be obtained in patients with a transient deficit. A transient deficit can be an indication of a more serious ligamentous and neurologic injury and subsequent need for immobilization.51
In a study of 74 children suspected to have a cervical spine injury based on plain radiography, MRI confirmed the plain radiography diagnosis in 66% and altered the diagnosis in 34%.52 Keiper and colleagues53 obtained MRI in children who had clinical symptoms consistent with a cervical spine injury despite having negative plain x-rays and CT studies. There were 16 abnormal results in 52 children, with posterior soft tissue injury being the most common injury. Four of these patients eventually underwent surgical stabilization, and MRI assisted with preoperative planning. MRI can be especially helpful in clearing the cervical spine in obtunded patients. Frank and colleagues54 showed the effectiveness of MRI at decreasing time to cervical spine clearance, length of time in the pediatric intensive care unit, and length of time in the hospital.
Spine Clearance
Early cervical spine clearance has multiple benefits and helps avoid complications. Cervical collars have known complications, including skin breakdown around the neck, dysphagia, pulmonary complications, increased intracranial pressure, and decubitus ulcers.38,55,56 After a cervical collar has been placed on a child, formal clearance must be obtained before it can be removed. Clinical clearance of the cervical spine has been evaluated using the National Emergency X-Radiography Utilization Study (NEXUS), which was originally developed for adults. NEXUS has been used as a decision-making instrument to determine the need for radiography. The criteria in adults for clinical clearance are absence of neck pain, neurologic symptoms, distracting injuries, or altered mental status (owing to injury, age, or intoxication). If any one of these four criteria is present, the patient is considered to be high risk and must be radiographically cleared. If none of these criteria are present, the collar can be cleared without further imaging.
Application of NEXUS criteria in pediatric patients was studied in a prospective multicenter study. All 30 cervical spine injuries were placed correctly into the high-risk group, and, more importantly, no cervical spine injuries were noted in the low-risk group. Additionally, use of NEXUS criteria decreased pediatric cervical spine imaging by 20%.57 These rules cannot be safely applied in children too young to cooperate with an examination, in children in the presence of a high-energy mechanism, or in children with associated injuries that heighten the suspicion of a spine injury. The Canadian C-Spine Rule has gained popularity in the adult literature, showing a higher sensitivity than NEXUS (99.4% vs. 90.7%) and a higher specificity (45.1% vs. 36.8%), but to the authors’ knowledge it has not been studied in children.58
It has been shown that an efficient, multidisciplinary approach can facilitate rapid clearance of the cervical spine, decreasing average time to 7.5 hours in nonintubated patients and 19.4 hours in intubated patients.23 This rapid clearance is dependent on a system that is safe and user-friendly enough to allow the primary team to perform the clearance. Anderson and colleagues59 evaluated the percent of cervical spines cleared by spine specialists before versus after initiation of a clearance protocol, noting a 60% increase in spines cleared by nonspine physicians without any late injuries detected.
Children with no evidence of injury on plain radiography or CT scan who have persistent pain should remain in the cervical collar with later clearance in the clinic with dynamic radiographs. In the presence of a neurologic deficit or a history of a transient deficit before arrival, MRI of the entire spine should be obtained. If spinal cord injury without radiographic abnormality (SCIWORA) is suspected, the cervical collar should remain in place, spine precautions should be continued, and the patient should be admitted for observation because there can be delayed deterioration.60,61
Clearing the cervical spine in an unconscious patient can be facilitated by a standard protocol. If the patient returns to a normal mental status, the protocol for a conscious patient can be used. There have been reports of delayed neurologic deterioration in patients with altered mental status and unrecognized ligamentous injury that subluxated on mobilization.62 The two modalities available for evaluating ligamentous injury in an obtunded patient include fluoroscopic flexion-extension and MRI. Fluoroscopic flexion-extension examination can be labor-intensive, and in an adult series, it was difficult to visualize the cervicothoracic junction.63 Because of these problems, many investigators believe that MRI is superior for evaluating ligamentous injury.
MRI has been found to be most sensitive for detecting a ligamentous injury at postinjury day 2 or 3.64 Stassen and colleagues65 used MRI within their protocol at a Level I adult trauma center for assisting in cervical spine clearance. All obtunded patients with a negative CT scan of the cervical spine underwent MRI on postinjury day 3 if they could not be clinically cleared. MRI allowed clearance of the cervical collar in 60% of subjects, and there was no delayed neurologic sequela. The remaining patients were treated in a cervical collar for 6 weeks without complication.
The best available evidence indicates that MRI is the most sensitive study for detecting posterior ligamentous disruption and should be the primary study in obtunded patients.66 Equivocal MRI findings can be evaluated further with flexion-extension radiographs, or the physician can forgo further study and treat the patient with a collar for 6 weeks. At the termination of the 6-week period, the patient should undergo a flexion-extension series to ensure adequate healing. Expedient clearance of the thoracolumbar spine in a child with polytrauma is also very important to prevent skin breakdown, respiratory complications, and ileus. At the authors’ institution, the CT traumagram, with sagittal and coronal reconstructions, is used to augment the clinical examination and clear the thoracolumbar spine.
Spinal Cord Injury in Children
The mechanism of spinal cord injury differs depending on age.22 Children younger than 8 years old more often sustain spinal cord insult involving the upper cervical spine, secondary to ligamentous injury often with no discernible bony changes. Children older than 8 have spinal cord injuries with associated fractures owing to biomechanics of the spine that more closely mirror the biomechanics of the adult spine. A thorough neurologic examination should be obtained to determine the level involved and whether the lesion is complete or incomplete. It is important to note whether there is sacral sparing as indicated by sensation at the anal mucocutaneous junction (S4-5 dermatome) on the left and the right side, ability to contract the anal sphincter voluntarily, and deep anal sensation. This sacral sparing indicates continuity of long tracts with improved likelihood for return of neurologic function.67 The Frankel classification and American Spinal Injury Association (ASIA) Impairment Scale have been used in pediatric patients to provide a standardized description of injury.
Damage to the spinal cord has been categorized into primary and secondary injury. Primary injury occurs at the time of the accident leading to structural damage of the neural elements and supporting blood supply. Secondary injury occurs within minutes of the trauma setting off a cascade of events that result in ischemia, increased cell membrane permeability, pathologic electrolyte shift, edema, and production of free radicals.68 This process continues to evolve over the subsequent days, and medical and surgical strategies are directed at mitigating secondary injury. Current treatments of interest include support of spinal cord perfusion, steroids, and early versus delayed decompression.
During transport and initial evaluation, it is crucial to prevent hypotension and to keep oxygen saturations high in patients suspected to have a spinal cord injury so as to help protect the spinal cord blood perfusion.69 Animal and adult studies have shown improved outcome in subjects aggressively managed to prevent spinal cord ischemia.70–72 This practice has been accepted by many pediatric trauma centers and includes admitting patients to the intensive care unit and accurately monitoring volume status and blood pressure. The mean arterial pressure should be maintained at greater than 80 mm Hg for 7 days.
Steroids are stabilizing agents; they act as a powerful anti-inflammatory decreasing edema and scavenging oxygen free radicals. High-dose methylprednisolone is administered to children based on data collected in adults. The recommendations on timing and dose are based on studies performed in patients as reported in the National Acute Spinal Cord Injury Study III.73,74 Methylprednisolone is administered intravenously at a loading dose of 30 mg/kg over 15 minutes followed by 5.4 mg/kg/hr continuous infusion. If administration of the steroid is initiated within 3 hours of injury, the infusion should be continued for 23 hours. If steroid administration is started at 3 to 8 hours after injury, it should be continued for the subsequent 48 hours.
Steroids should not be given if a child presents after 8 hours or if the spinal cord injury is secondary to penetrating trauma. In this setting, there are significant risks with little to no benefit.75 The National Acute Spinal Cord Injury Study III73,74 excluded all children younger than 13 years of age. There are no equivalent data in young children, and in this age group the use of steroids is not evidence based.
The indications for early operative intervention for pediatric patients with spinal cord injury include radiographic confirmation of spinal cord compression in the setting of an incomplete injury or progressive deficit, an open spinal injury, and a grossly unstable spine in patients who are neurologically intact. To the authors’ knowledge, there have been no well-designed prospective studies in pediatric patients evaluating the effect and timing of decompression.76
Functional outcomes depend on the level of injury and whether it is complete or incomplete. Patients with injuries above C4 may be dependent on a respirator, and phrenic nerve pacemakers can be implanted. Patients with C3 lesions can shrug their shoulders and often have neck motion, permitting operation of equipment with sip/puff controls, voice activation, eyebrow or eye blink, and head or chin controls. Patients with C6 lesions are often able to propel a manual wheelchair with the assistance of fusions and tendon transfers. At least partial recovery after a complete spinal cord injury has been reported in 10% to 25% of patients.22,69,75,77 In a series reported by Wang and colleagues,78 64% of patients showed at least partial recovery after spinal cord injury. Of patients with complete injuries, 25% eventually became ambulatory. Recovery was seen up to 1 year after injury.
Children are also better able to adapt to an injury. Anderson and colleagues79 interviewed 161 adults who had sustained a spinal cord injury as a child and found that 64% lived independently, and approximately 50% of patients reported being satisfied with their quality of life. Concerning outcomes from operative versus nonoperative treatment of spine fractures associated with spinal cord injuries, one study showed that patients in the operative group were able to use their wheelchair 5.2 weeks earlier.80 Early activity is crucial in paralyzed patients to avoid pulmonary complications, disuse osteopenia, and complications from insensate skin.67
Complications unique to pediatric patients with a spinal cord injury are susceptibility to development of post-traumatic deformity and growth arrest.81 Causes can be divided into intrinsic, extrinsic, and iatrogenic factors. Intrinsic factors include injury to the vertebral apophyses resulting in abnormal growth and change in biomechanics from altered shape of the vertebral body and loss of posterior ligamentous support. This change in biomechanics can alter the forces on the vertebral apophyses, exacerbating the deformity further.82 Extrinsic factors include weak trunk muscles in the setting of gravity and spasticity with a contracture. Iatrogenic factors include improperly instrumented segments and use of a laminectomy without fusion. The risk of developing a deformity, in the setting of a laminectomy without fusion, is approximately 50%, with a much higher incidence in the cervical and thoracic spine.83 Nonoperative treatment with a brace can help slow the progression of deformity, temporizing the situation and allowing the child maximum time to grow before performing a fusion.84 Performing surgical correction and fusion needs to take into account remaining growth. An estimate of remaining growth can be determined by multiplying 0.7 mm times the number of segments fused times the number of years of remaining growth.85
Posterior fusion in young children has the added drawback of crankshaft phenomenon. This phenomenon occurs with continued growth of the anterior column in the setting of a fused posterior column. With continued anterior growth, the apical vertebral body rotates producing a scoliotic curvature.86 Delaying surgery must be balanced with addressing curves while they are still supple. The goals of surgery include halting progression, obtaining correction, and balancing the spine and pelvis to equalize sitting skin pressure. Indications include a scoliotic curve of greater than 40 degrees and kyphotic curves of greater than 60 degrees. Anterior release may be needed if the deformity is rigid as shown on bending films. Including the sacrum in the fusion construct is recommended if pelvic obliquity exists.
Spinal Cord Injury Without Radiographic Abnormality
The biomechanical differences in the spine of children younger than 8 years place them at risk of SCIWORA.22 This entity was first described by Pang and Wilberger87 in 1982 before the use of MRI. The term SCIWORA was developed to describe spinal cord injuries without overt vertebral column disruption as displayed by conventional x-rays, CT scans, myelograms, and dynamic flexion-extension radiographs. SCIWORA excludes injuries secondary to penetrating trauma, secondary to electrical shock, secondary to obstetric complication, and in association with congenital anomalies. The incidence of SCIWORA in patients with spinal cord injury for ages birth to 17 was reported to be 35% in earlier literature.61 As MRI has become more widely used, the incidence in children with spinal cord injuries is reported to be 3%.5 This change likely reflects an improvement in imaging quality and the ability to detect subtle injuries previously missed, rather than a change in actual injury type. More recently, Bosch and colleagues88 found that recurrent SCIWORA was an uncommon entity, which occurred only in low-energy sports-related injuries, resulting in transient neurologic symptoms, with full recovery in all cases.
MRI is the study of choice for evaluation of patients suspected to have SCIWORA. There are extraneural soft tissue changes and intraneural changes. The changes seen on MRI are secondary to edema and methemoglobin, a processed form of hemoglobin. Edema is seen as isointense on T1 and hyperintense on T2; extracellular methemoglobin is seen as hyperintense on T1 and hyperintense on T2. MRI changes in the extraneural tissues can be detected within hours of injury because the blood is quickly metabolized into a form easily seen on MRI. Intraneural changes can take days to be detectable because of the delayed metabolism of a hemorrhage into a form visible on MRI.89,90 It is recommended that MRI be obtained at the time of presentation to rule out a compressive lesion that needs to be surgically addressed and at 6 to 9 days after injury to improve detection of intraneural injuries related to SCIWORA.61
The recommended duration of immobilization in a brace has been controversial, with the concern for delayed neurologic deterioration or reinjury motivating the recommendation for a brace. Bosch and colleagues88 found that immobilization did not prevent recurrent SCIWORA, and it did not improve the outcomes of primary SCIWORA after instability was properly ruled out. A meta-analysis by Launay and colleagues60 showed that patients immobilized for 8 weeks had a 17% chance of developing recurrent SCIWORA, whereas no patients immobilized for 12 weeks had recurrent SCIWORA develop. Based on this information, it is recommended that children be immobilized for 12 weeks with flexion and extension views of the injured region at 12 weeks to detect instability.
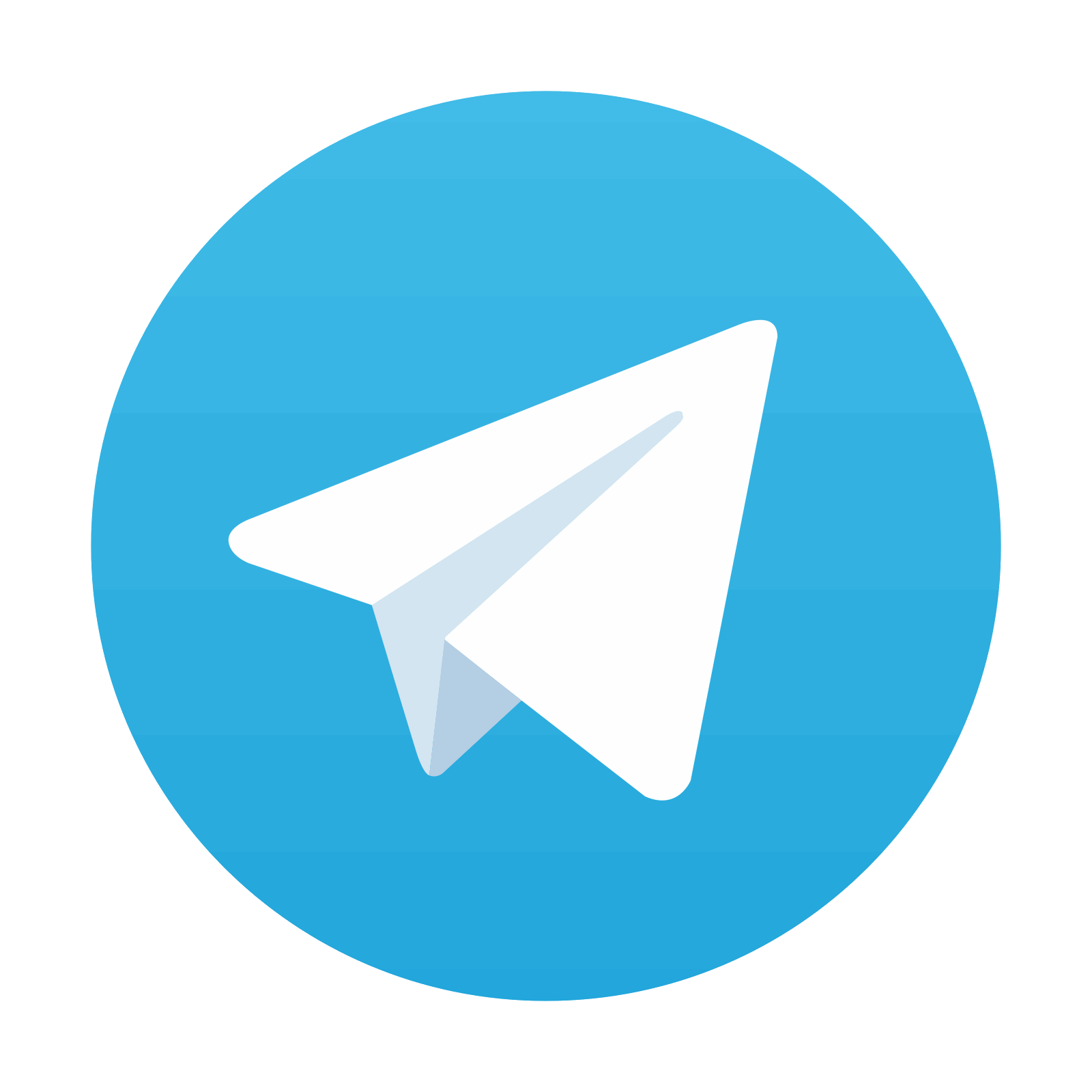
Stay updated, free articles. Join our Telegram channel

Full access? Get Clinical Tree
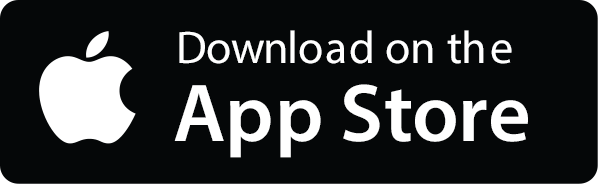
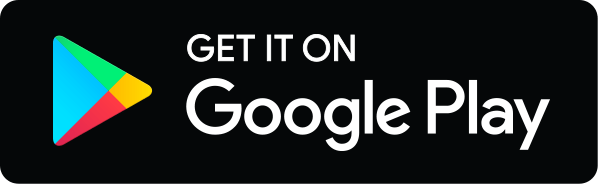