Ceramic-on-Ceramic Bearings
Aaron Carter and Peter F. Sharkey
Key Points
Introduction
The most frequently used bearing surface in total hip arthroplasty (THA) is the metal head articulating with an ultra-high-molecular-weight polyethylene socket. It is considered the standard bearing surface for THA in the United States. As the indications for THA are extending to a younger and more active population, the focus has been moving toward increasing the longevity of these implants. Wear debris from polyethylene leading to periprosthetic osteolysis is considered the major long-term complication resulting in the need for revision.1–3 The extent of osteolysis is of major importance not only in terms of the likelihood of failure, but also in terms of the complexities of obtaining fixation of new components during revision surgery and the long-term success of the revision surgery itself.1 The need for improved bearing surfaces has led to cross-linking of polyethylene via a variety of methods. These advancements have greatly improved the wear characteristics of polyethylene.4,5 Studies have shown that cross-linking polyethylene in an articulation with a metal or a ceramic head can reduce wear by more than 50%.6–8 However, cross-linking polyethylene may weaken the polyethylene, causing catastrophic failure with cracking of the liner.9–11
Ceramics were originally introduced as a solution to the problems of friction and wear seen in metal-on-polyethylene and metal-on-metal configurations. The intended goal of the ceramic-on-ceramic bearing is to reduce biologically active wear debris, thereby minimizing the occurrence of osteolysis and aseptic loosening.12 The standard ceramic-on-ceramic articulation is the alumina-on-alumina bearing. Both in vitro and clinical retrieval studies have demonstrated a significant reduction in wear and particle production when compared with metal on polyethylene articulations.13–17 Osteolysis in these bearings appears to be minimal or nonexistent.18,19 Although osteolysis has only rarely been identified with the ceramic-on-ceramic articulation, potential disadvantages have been identified. Ceramics are hard, brittle materials that lack fracture toughness. Improvements in processing and machining of ceramics have reduced fracture risk; however, this complication has not yet been eliminated.15,20-24 Other disadvantages of ceramic-on-ceramic articulations include stripe wear, motion-related noise, impingement, and limited head and liner options.
This chapter will review the basic science of ceramic-on-ceramic bearings, including mechanical properties, advantages, and disadvantages of this articulation. Clinical studies related to ceramic-on-ceramic bearings will be summarized, and future directions will be discussed.
Basic Science
History
The ceramic-on-ceramic articulation was first introduced by Pierre Boutin and associates in 1970 as an alternative to the conventional metal-on-polyethylene total hip.25 Early results for this first prosthesis showed promise; however, ceramic fracture was a matter of concern.26 High fracture rates were attributable to the large grain sizes, low density, and impurity of the alumina.27 Prior fixation methods involved gluing the head to the stem with a resin or screwing it in place.26 As the production of surgical-grade dense alumina ceramic evolved, strong fixation of the ceramic head to the metal stem was achieved with the introduction of the Morse taper in 1977, significantly reducing the risk of fracture to 2%.26,28 Current alumina production techniques have brought the fracture rate to 0.004%.29 With the reduction in the risk of fracture, acetabular component fixation leading to loosening and subsequent revision became the major long-term problem for these devices. Various methods of fixation were explored, finally leading to the use of porous-coated titanium shells with modular acetabular inserts press-fit into bone.26 Despite their use in Europe, alumina femoral heads only became available in the early 1980s in the United States, with ceramic-on-ceramic alumina bearing surfaces available by the early 2000s.30 Alumina has been a standardized material since 1984 (International Standard Organization [ISO] 6474).26
General Statements About Ceramics
The two ceramic materials currently in clinical use as bearing surfaces are aluminum oxide (alumina) and zirconium oxide (zirconia). The ceramic-on-ceramic bearing surface is an alumina-on-alumina bearing surface because zirconia produces high wear when articulating against itself.31 These materials exist in their highest oxidation state, allowing excellent biocompatibility, thermodynamic stability, chemical inertness, and resistance to corrosion.31 Ceramics are water insoluble and have excellent compression strength but poor bending strength.28,31 Because of their mechanical properties, ceramics are considered hard and brittle in nature.
Use of proprietary ceramic processing methods by each manufacturer reflects the fact that all ceramics are not alike and subtle differences exist between ceramics manufactured by each company.
Manufacturing Process
Alumina ceramics are manufactured under a complex process involving multiple steps under intense and optimal quality control. The mechanical properties of the final product rely heavily on proper performance of these manufacturing steps.26
The current third generation of ceramic processing consists of mixing aluminum oxide powder with organic bonding agents, water, and lubricants.27 The mixture is isostatically pressed into a mold that will give it its final shape. The formed piece is then dried, while the water is evaporated, and a thermal process removes the organic binder. The product is then sintered at a very high temperature (between 1600° C and 1800° C) under high pressure. The quality and purity of the initial powder and control of precision over the thermal process applied affect the final microstructure of the ceramic.26,28 Mechanical strength and tribological characteristics are determined by the purity, porosity, and grain size through the ceramic. When ceramics were first produced, longer sintering times were necessary to achieve full or nearly full density; however, larger grain size resulted, thus reducing overall strength and contributing to early failures.27,31 The addition of materials such as CaO or MgO prevented grain growth, allowing manufacturers to achieve smaller grain sizes and thus higher strength and reliability.31 These additions in the late 1980s and early 1990s would be considered second-generation ceramics, which would later give rise to the third-generation ceramics in use today.27 The third-generation ceramics were developed in 1994 and constitute ceramics that employ hot isostatic pressing, further resulting in smaller grain size, minimal grain boundaries and inclusions, and increased burst strength and wear resistance.29 Currently, four major companies in the world meet the technologically demanding and complex process requirements needed to produce medical grade ceramics, including Ceraver Osteal (Roissy, France), Ceramtec AG (Stuttgart, Germany), Morgan Advanced Ceramics (Rugby, UK), and Kyocera (Kyoto, Japan).26 Since 2003, several companies have released ceramics for use in the United States (Wright Medical Technology, Arlington, Tenn; Stryker-Howmedica-Osteonics, Allendale, NJ; Encore Medical, Austin, Tex; Smith & Nephew, Memphis, Tenn; Biomet, Warsaw, Ind; DePuy Orthopaedics, Warsaw, Ind; Zimmer, Warsaw, Ind; Stelkast, McMurray, Pa; and Exactech, Gainesville, Fla).26,32
Flaws in the manufacturing process can lead to catastrophic ceramic fracture. Crack propagation and subsequent fracture can result from flaws as small as the size of a few alumina grains.31 Improvements in the manufacturing process such as diminishing the size of grains used for fabricating components have reduced the risk of fracture. When ceramics were first introduced, the average grain size was 50 µm. The alumina used today has an average grain size of 2 µm, contributing to the substantial decrease in risk of catastrophic fracture.33
Alumina Ceramics Mechanical Properties
Alumina ceramic is a highly oxidized, hard, stable monophasic polycrystalline form of industrial sapphire.34 Because of its high oxidation state, alumina exists in a low state of energy and exhibits a high state of thermodynamic stability. It has a high thermal conductivity coefficient and exhibits excellent resistance to corrosion. Alumina is also biologically inert and is resistant to further oxidation because of its fully oxidized state.31 The ionic structure of alumina creates a hydrophilic state, resulting in fluid film lubrication and leading to higher wettability compared with orthopedic polymers and metals.26,35 This increases lubrication of the joint. The intrinsic hardness of alumina makes it highly abrasion and wear resistant.26,36 The hardness of alumina increases resistance to scratching, making it much less likely to scratch than titanium or cobalt chrome alloys. The only material that is capable of scratching alumina is diamond. Scratch resistance makes alumina resistant to third-body wear.37
Alumina has strong compression strength despite poor bending characteristics. Alumina also lacks the ability to deform under high stress owing to its stiffness. This has limited ceramic use to only the femoral head and the cup liner. Polyethylene has the capability to mold around the femoral head if any inconsistency is present between articular surfaces. Ceramics lack this capability and are subject to high wear rates if any articulation mismatch occurs.13,38 Clearance between the ceramic femoral head and the socket must be over 50 µm to prevent grain detachment and third-body wear.39 Lack of ceramic deformation makes the points of contact between articulation surfaces smaller than with metal-on-polyethylene articulations. Advances in manufacturing techniques since 1993 have made it unnecessary to factory-match components.
Mechanical and biological factors have been linked to cement fragmentation and eventual loosening.35 The stiffness of alumina ceramics is roughly 300 times higher than that of cancellous bone and is 190 times higher than that of polymethylmethacrylate (PMMA).26 This modulus mismatch alters the stress distribution across the ceramic-cement-bone interface. This in combination with stress shielding makes microfracture of cement likely.35 In addition, the low damping capacity of alumina may increase the risk of cement or bone microfracture due to excess transmission of impact loading.35 These properties prevent the use of ceramics in the manufacture of stem or socket components.
Alumina ceramics are brittle because of excellent compression strength and low bending strength. Ceramics exhibit a linear elastic behavior and low fracture toughness, causing them to break without warning.26 The risk of ceramic fracture is determined by the initial flaws of the ceramic. The incidence of fracture has substantially decreased over time owing to smaller grain size, fewer impurities, laser etching, and proof testing. The burst strength of alumina has improved over time from 38 kilonewtons (1977) to 98 kilonewtons (1998), far exceeding the U.S. Food and Drug Administration (FDA) limit of 46 kilonewtons.26
Tribological Properties
Tribology is the science of the mechanisms of friction, lubrication, and wear of interacting surfaces that are in relative motion. In vitro studies and retrieval analysis have provided useful information on the function and behavior of ceramic-on-ceramic prostheses.
Numerous in vitro wear studies have documented the low friction and wear of alumina on alumina.40,41 Low surface roughness secondary to small grain size, hardness contributing to scratch resistance, and enhanced wettability and fluid film lubrication result in the excellent tribological properties of the alumina articulation. In vitro testing has demonstrated that two phases of wear are present.42 The first million cycles constitute the “run-in” phase, and wear rates for alumina on alumina range between 0.1 and 0.3 mm3 per million cycles.42,43 The second phase is termed the steady-state phase, and volumetric wear rates decrease to less than 0.01 mm3.42,43 This wear is considerably less when compared with the traditional metal-on-polyethylene bearing, and in some studies is up to 5000 times less.26,37,42,44
Accelerated wear can occur in alumina-on-alumina couples in certain clinical situations. A unique phenomenon of stripe wear occurs from microseparation during the swing phase of gait, or when the ball is levered out of the socket by impingement, resulting in edge loading and accelerated wear over a discreet area. Conditions associated with ceramic wear include vertical cup position, femoral neck impingement, and femoral head separation.45 In vitro testing that simulates microseparation has been able to reproduce wear rates and patterns comparable with those seen clinically.21 Wear as high as 1.24 mm3 per million cycles can occur with separation and stripe wear. Particles produced from wear also exhibit a bimodal distribution that is speculated to be generated by two separate mechanisms in vivo. Nanometer-size particles (5 to 90 nm) are produced from polishing under normal articulating conditions. Micrometer-size particles (0.05 to 3.2 µm) are produced by stripe wear and transgranular fracture of the ceramic.21,46
Numerous retrieval studies have been performed to analyze the performance of ceramic-on-ceramic bearings. One study examined alumina component retrieval associated with aseptic loosening of the socket at a mean of 11 years after implantation.13 Components were classified into three groups: (1) low wear with no visible sign of wear; (2) stripe wear with a visible oblong worn area on the femoral heads and penetration less than 10 µm/yr; and (3) severe wear with visible loss of material on both components, and maximum penetrations greater than 150 µm. Examination of the 11 components revealed massive wear on two devices, and the remaining nine components demonstrated linear wear rates less than 15 µm/yr. The authors concluded that one type of wear has a negligible effect on the long-term life of the implant, and a second type of wear leads to catastrophic destruction of the bearing surface. Clinical wear rates of the bearing surface vary, ranging from 0.3 µm/yr to 5.0 mm/yr.37,44,47 Improvement in alumina quality, in addition to factors responsible for a load increase (weight, young age, and male gender) or for impairment in the load distribution over the component surfaces (large grain size, nonoptimal initial cup inclination, and cup migration and/or tilting), can account for these variations.13 It is important to note, however, that most catastrophic wear has been reported in products produced before 1990.13,27,29 Recent wear rates have been consistently below 15 µm/yr.13,47
Wear Debris and Tissue Response
In vitro and in vivo studies have shown that alumina debris is biologically inert and well tolerated. The small size and the low volume of alumina wear particles generated result in a low level of bioactivity.12,18,48-50 In contrast with polyethylene or metallic particles, giant cells have not been observed in contact with alumina wear particles. One study compared 12 periprosthetic membranes collected during revision surgery for aseptic component loosening in an alumina-on-alumina articulation, and compared them with membranes collected from metal-on-polyethylene bearings.35 In the alumina-on-alumina group, the mild cellular reaction was in fact due to the use of zirconia ceramic particles in the cement as an opacifying agent. The zirconia ceramic was present in large enough amounts to illicit a small macrophage response, which subsequently contributed to component loosening. No cellular reaction to the alumina particles was noted, which contrasted with the polyethylene debris generated from the metal-on-polyethylene group.
Osteolysis associated with alumina-on-alumina bearings has been reported rarely. One study demonstrated a high revision rate (27%) due to aseptic loosening in a cohort of 69 hips when the bearing was made with ceramics consisting of large grain size and high porosity.38 These factors resulted in a large production of debris and osteolysis. Another observation found periprosthetic tissue from alumina-on-alumina couples retrieved at revision surgery to have lower prostaglandin E2 levels than tissue obtained from hips with metal on polyethylene.38
A separate study demonstrated that alumina and polyethylene debris stimulate release of tumor necrosis factor (TNF).51 Cell mortality and the amount of TNF released increase with the size and concentration of alumina particles. This same study also showed that polyethylene particles cause greater release of TNF, stimulating up to 8 to 10 times the quantity of ceramic particles.51 Alumina particles have been shown to induce macrophage apoptosis, which provides a mechanism to explain the lower levels of TNF. This could also explain the differences seen in osteolysis patterns of ceramic-on-ceramic when compared with metal-on-polyethylene articulations.50
Ceramic Advantages
The primary advantage of using a ceramic-on-ceramic articulation is decreased wear and osteolysis.7,18,26,41,43 Osteolysis is considered the major phenomenon preventing development of a longer-lasting hip replacement. As the indications for total hip arthroplasty have been extended to younger, healthier, and more active patients, the need to eliminate wear and osteolysis has become more apparent.
The tribological properties of alumina make ceramics ideal for the articular surfaces of total hip arthroplasty. The surface roughness of alumina has been greatly reduced with reduced grain size and improved polishing technology resulting from advances in material processing. The inherent hard characteristics of alumina make the material resistant to scratches, minimizing third-body wear from bone, cement, or metal debris. Alumina ceramic is also considered to have high compression strength and low bioreactivity. One advantage of ceramics over metal-on-metal bearings is that they do not increase serum or cellular ion content.52,53 The ionic properties of alumina in combination with body fluids make the ceramic surface wettable, creating a fluid film that decreases friction. Low wear rates in combination with minimal bioreactivity decrease the likelihood of osteolysis. With current implant design and advanced ceramic processing, osteolysis has not been reported in follow-up as long as 18.5 years.19
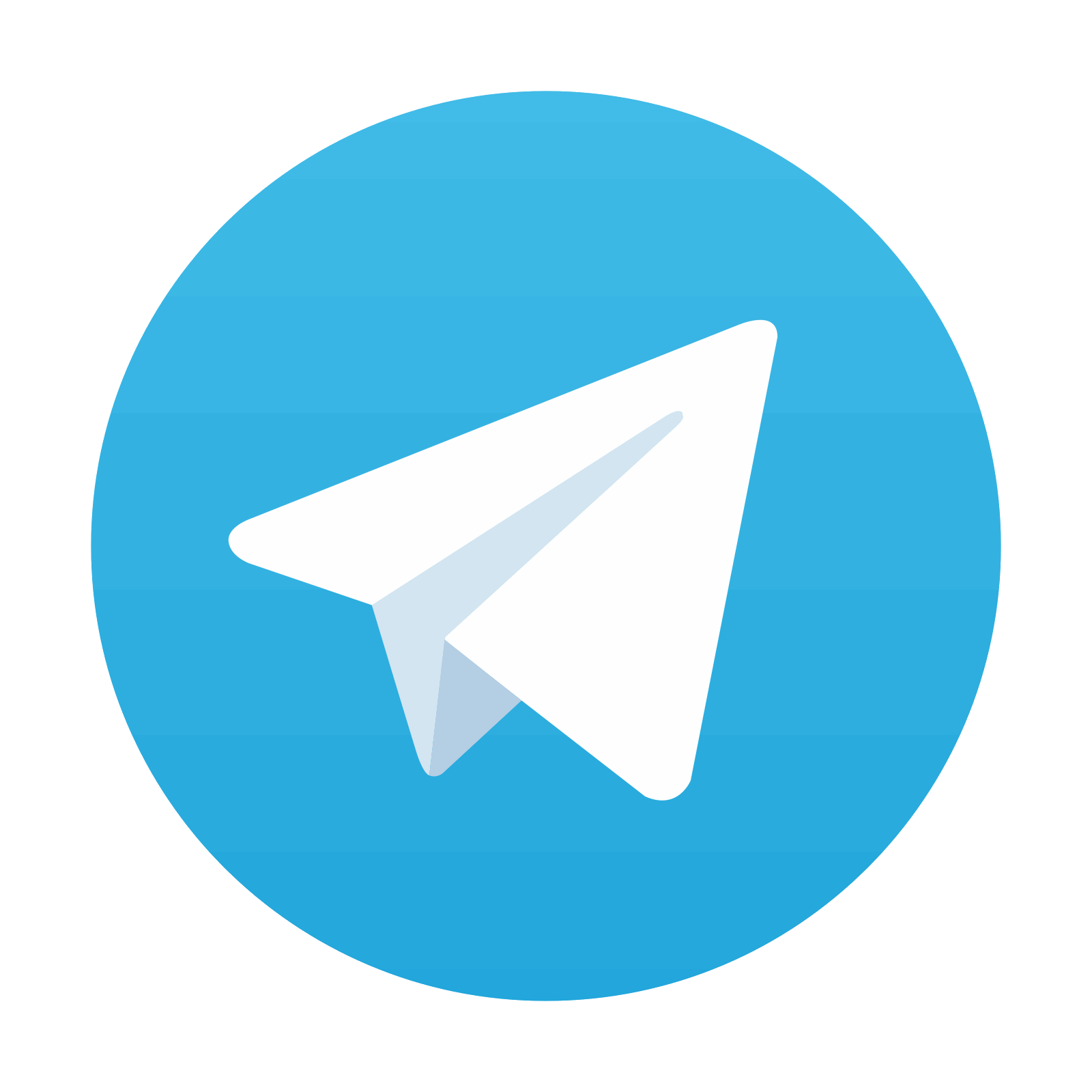
Stay updated, free articles. Join our Telegram channel

Full access? Get Clinical Tree
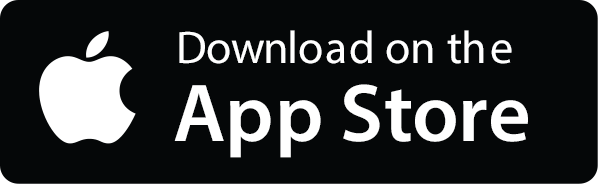
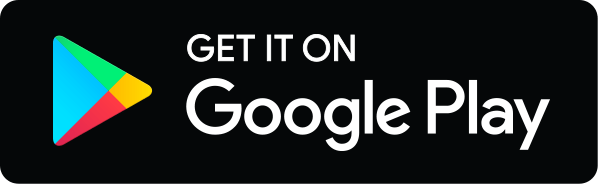