CHAPTER 7 Biomechanics of the Spinal Motion Segment
From a biomechanical standpoint, the spine seems to accomplish three major functions.1 First, the spine provides a structure by which loads can be transmitted through the body. Second, the spine permits motion in multidimensional space. Third, the spine provides a structure to protect the spinal cord. To appreciate the ability of the spine to accomplish these functions, we need to understand the natural movements of the spine and the ability of the spine to withstand forces or loads that are transmitted through the structure.
Physical Characteristics of Spine Structures
Several physiologic curves are also characteristic of the upright spine (Fig. 7–1A). The curves within the cervical and lumbar regions of the spine are referred to as cervical lordosis and lumbar lordosis, whereas the thoracic and sacral curves are referred to as thoracic kyphosis and sacral kyphosis because these curves bow in the opposite direction of the lordotic curves. These curves work collectively to accommodate pelvic orientation under different conditions. When sitting, the pelvis rotates backward and the lumbar curve flattens. When the pelvis is rotated forward, the lumbar curve is accentuated. Collectively, the spinal curves balance each other and form a stable system that maintains the center of gravity in a balanced state.
The “building blocks” of the spine are the spinal motion segments (Fig. 7–1B), also known as the functional spinal unit. This unit consists of two vertebrae and the disc in between them. This unit represents the central focus of biomechanical functioning and clinical assessment. This chapter explores the spinal motion segment from a biomechanical perspective with the intent of understanding the significance of features that may influence status.
Support Structures
The spine is constructed of a series of vertebral bones that are stacked on one another to form the spinal column that runs from the pelvis to the head. A vertebral bone, or vertebra, is shown in Figure 7–2. The large round portion of the bone is the vertebral body and represents the major load-bearing structure of the spinal column. The outer portion of this bone is composed of a thin yet very strong layer of cortical bone. Cortical bone, also known as compact bone, forms a protective outer shell, has a high resistance to bending and torsion, and provides strength in situations where bending would be undesirable. The inner portion of the bone consists of a spongy matrix of cancellous bone. This type of bone is less dense and more elastic than cortical bone. Cancellous bone forms the interior scaffolding of the structure and helps the bone to maintain its shape despite compressive forces. This structure is composed of bundles of short and parallel strands of bone fused together.
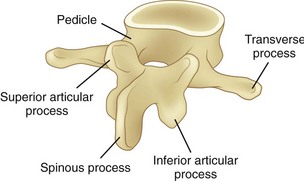
FIGURE 7–2 Lumbar vertebra and its posterior elements.
(Adapted from Marras WS: The Working Back: A Systems View. Hoboken, John Wiley & Sons, 2008.)
Posterior of the vertebral body are bony structures that constitute the posterior elements and form a protective channel or tunnel for the spinal cord (see Fig. 7–1B). The biomechanical role of the posterior elements is to control the position of the vertebral bodies. These elements provide attachment points for muscles to control the position of the vertebra and supply lever arms to provide the system with mechanical advantage. In addition, these structures control motion and provide mechanical “stops” to prevent excessive movement of the vertebral body. A significant portion of the mechanical load is borne by the posterior elements, relieving the disc of excessive loading.
As shown in Figure 7–2, toward the top of the posterior surface of each vertebra are pedicles. The pedicles provide a robust support structure (a type of pillar) to transmit force between the posterior elements and the vertebral body. Projecting out from each pedicle are the lamina structures that come together at the midline of the body and form a neural arch. This arch is a strong structure that provides protection to the spinal cord in the form of a channel (vertebral foramen).
Disc
The disc consists of two distinct portions, each of which is associated with a distinct mechanical function. The outer portion of the disc, called the anulus fibrosus, consists of alternating layers of fibers that are oriented at a 60- to 65-degree angle relative to the vertical. The anulus fibrosus consists of about 10 to 20 concentric, circumferential sheets of collagen called lamellae that are nestled together around the periphery of the disc (Fig. 7–3). The lamellae are stiff and can withstand significant compression loading. Given the collagenous nature of these lamellae, they are pliable and can also permit bending of the spinal column. If the structure were to buckle, however, it would lose its stiffness and would be unable to support compression. The second portion of the disc (nucleus pulposus) is designed to overcome this potential problem.
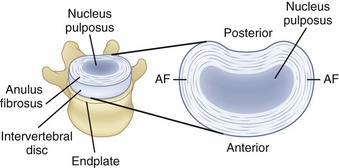
FIGURE 7–3 A, Disc, vertebral endplate, and vertebral body. B, Construction of intervertebral disc.
(Adapted from Marras WS: The Working Back: A Systems View. Hoboken, John Wiley & Sons, 2008; Bogduk N: Clinical Anatomy of the Lumbar Spine and Sacrum, 4th ed. Edinburgh, Churchill Livingstone, 2005.)
Within the anulus fibrosus is a gelatinous core referred to as the nucleus pulposus (see Fig. 7–3). When compressed, this core expands radially and places the anulus fibrosus in tension, providing stiffness. The integrity of the system changes throughout the day. The disc absorbs water while one is recumbent, which makes the system stiffer than when one is upright. Conversely, when one is upright, water is squeezed out of the disc, and the structure becomes more lax.
Spinal Ligaments
The spinal ligaments are shown in Figure 7–4. The arrangement of these structures provides support for the spine in different dimensions of loading. Because support is offered in the different directions of motion, these structures provide stability when the spinal system is intact.
Coordinate System and Force and Movement Definitions
A biomechanical assessment of the spine is concerned with the assessment of movements and forces developing within the spine as it is exposed to activities of daily living and other work or environmental conditions. Movements or motions are compared with the natural limits of movement, and forces imposed on a tissue (also called tissue loading) are compared with the tissue tolerances (magnitude of load at which damage occurs). To describe movement and force transmission through tissue accurately, it is necessary to describe precisely direction of movement and direction and magnitude of the force application on the tissue. Direction is defined relative to a coordinate system or reference frame. The central (global) coordinate system of the body is shown in Figure 7–5. The origin or center of this coordinate system is located at the base of the spine. Figure 7–5 describes the coordinate system (used in this chapter) as a traditional three-dimensional cartesian coordinate system with three mutually perpendicular axes oriented with a vertical Z-axis. Some references have adopted the ISB coordinate convention, where the Y-axis is defined as the vertical axis.
Within the spinal motion segment or functional spinal unit, a local coordinate system can also be defined. The convention that defines this local coordinate system is shown in Figure 7–6. Movement of the spinal motion segments is defined relative to the subjacent vertebrae. Movements of the motion segment can be either translations (indicating straight line movements in any direction) or rotations (indicating movement around a point as when bending).
Figure 7–6 indicates that forces and moments (torques) can develop along each dimension of the reference frame. Forces along the Z dimension are either compression or tension depending on whether they compress the spinal motions segment or pull on the tissues. These are typically the forces one is concerned about when lifting an object in the sagittal plane. Two types of shear forces are also of concern when evaluating the biomechanics of the spine. Anteroposterior shear force describes the forward or backward force in the Y-axis that can result from pushing or pulling activities. The lateral shear forces refer to the sideways forces acting along the X-axis and represent the forces that develop in the spinal motion segment when one pushes an object to the side of the body.
Compression of the disc causes pressure within the nucleus pulposus in all directions, and this pressure places the anulus fibrosus under tension. As shown in Figure 7–7, the nucleus pressure can lead to deformation near the center of the endplate with this form of loading.
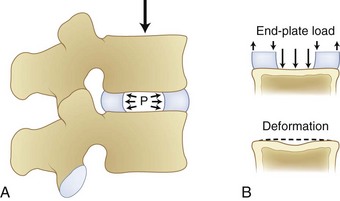
FIGURE 7–7 Compression of disc leading to increased pressure in disc nucleus and deformation of endplate.
(From White AA III, Panjabi MM: Clinical Biomechanics of the Spine, 2nd ed. Philadelphia, JB Lippincott, 1990.)
Figure 7–8 illustrates how shear, torsion, and tension influence the fibers of the anulus. Shear forces tense the fibers in the direction of movement and relax the fibers in the opposite direction. Similarly, torsion or twisting tenses the fibers that are lengthened by the movement and relaxes the remaining fibers. This differential of force among the fibers is believed to result in tissue damage. Finally, lengthening of the spine places the fibers under tension. This action increases the force on all the fibers regardless of their orientation.
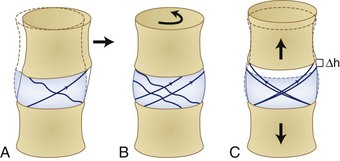
FIGURE 7–8 The effects of shear (A), torsion (B), and tension (C) on the fibers of the anulus fibrosus.
(From Adams MA, Bogduk N, Burton AK, et al: The Biomechanics of Back Pain, 2nd ed. Edinburgh, Churchill Livingstone, 2006.)
Bending moments refer to forces acting around an axis in Figure 7–6. The curved arrows in this figure show the direction in which moments act around a spinal segment. A bending moment can be defined around the X-axis resulting in a movement in the sagittal plane (forward bending moment), or it can be defined around the Y-axis indicating a sideways or lateral bend. In either of these situations, the moment or toque around the central axis defines the loading of the segment. Twisting of the spine can result when forces are applied around the Z-axis of the spine. This situation results in what is typically referred to as torsional moment.
The amount of displacement between the neutral position of the vertebra and the point at which resistance to physiologic motion is experienced is referred to as a neutral zone.2 Neutral zones can be defined for translational and rotational movements. The neutral zone can be described for each of 6 degrees of freedom.
Tissue Load Characteristics
The forces represented in Figure 7–6 define the direction of load application and the magnitude of the force. The nature and temporal characteristics of the loading situation also define the probability that the load application will result in tissue damage. It is believed that tissue damage can result from several different “types” of trauma to the tissue. Each type of trauma is believed to be associated with very different tolerance levels. First, acute trauma is the most familiar type of loading. Acute trauma refers to a single application of force that exceeds the tolerance level of the tissue. This would be the case if a large load were imposed on the spinal motion segment and a rupture of the disc occurred. In this case, the magnitude of the force applied in a particular direction would far exceed the tissue strength of the disc resulting in a rupture.
More recently, a third type of biomechanical trauma (instability) has received much attention in the literature.3–8 Stability is the ability of a system to respond to a perturbation and reestablish a state of equilibrium.2 Instability of the spine refers to the abnormal displacement of spine under physiologic loading. The abnormal displacement can occur in translation or rotation, but most likely would be some combination of these two types of motions. These abnormal motions are often small in magnitude, but the displacement may be enough to stimulate pain in sensitive tissue. Stability is significant because it is often the initiator of tissue damage when the system is out of alignment or when the musculoskeletal system overcompensates for a perturbation.2 When the supporting musculature cannot offer adequate stability to a joint (owing to improper muscle recruitment, fatigue, structure laxity, or weakness), the structure may move abnormally and result in sudden and unexpected force applications on a tissue. This type of trauma is similar to the acute trauma pathway, but is initiated by a miscalculation of the muscle recruitment pattern.
Mechanical Degeneration—Tissues at Risk
Clinicians are beginning to understand that low back disorders can occur before tissue damage. Biochemical studies have shown that these types of tissue insults can result in an upregulation of proinflammatory cytokines. This upregulation may result in tissue inflammation at much lower levels of load than would occur under normal conditions. This inflammation makes nociceptive tissues more sensitive to pain and may initiate back pain.9
When endplate loading exceeds its tolerance limit, microfractures can occur in the structure. Microfracture of the endplate itself usually does not initiate pain because few pain receptors reside within the disc and endplate. Repeated microfracture of this vertebral endplate can lead to the formation of scar tissue and calcification that can interfere with nutrient flow to the disc fibers. Because scar tissue is thicker and denser than endplate tissue, the scar tissue interferes with nutrient delivery to the disc. This reduced nutrient flow can lead to atrophy and weakening of the disc fibers and disc degeneration. Because the disc has relatively few nociceptors except at the outer layers, this degenerative process is usually not noticed by the individual until the disc is weakened to the point where bulging or rupture occurs, and surrounding tissues that are rich in nociceptors are stimulated. Figure 7–9 describes this sequence of events that are believed to lead to disc degeneration.9
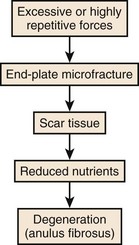
FIGURE 7–9 Sequence of events associated with cumulative or repeated trauma leading to disc degeneration.
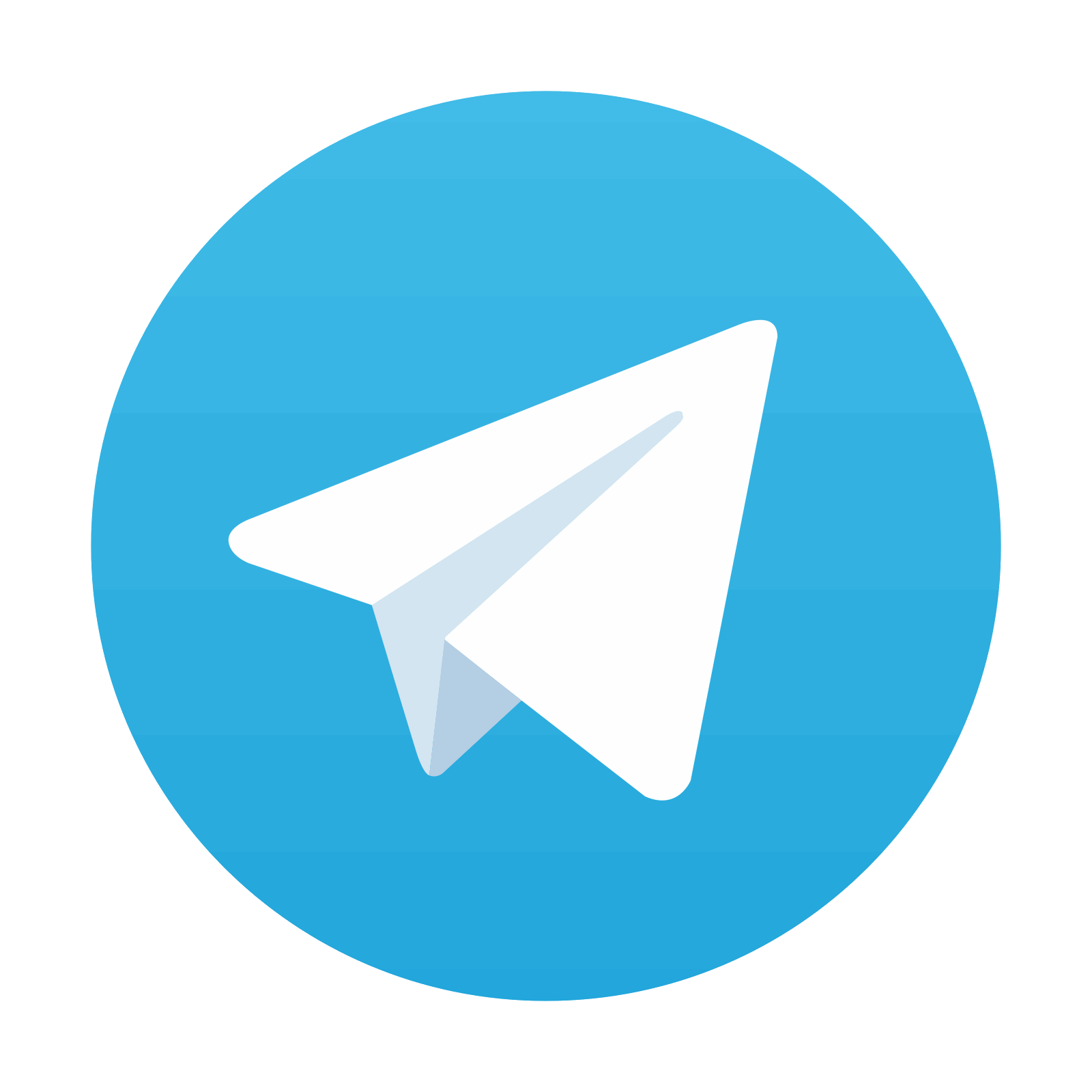
Stay updated, free articles. Join our Telegram channel

Full access? Get Clinical Tree
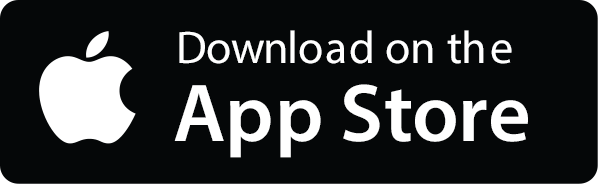
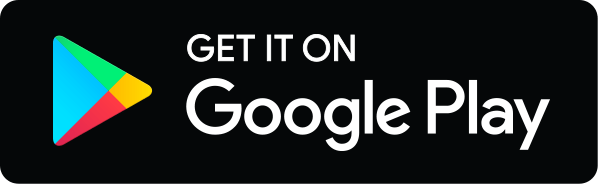