CHAPTER 2 Applied Anatomy of the Spine
Pars Interarticularis
The pars interarticularis defines the parts of the arch that lie between the superior and inferior articular facets of all subatlantal movable vertebral elements (Fig. 2–1). The term pars interarticularis arose to designate that area of the arch that is most stressed by translational movement between adjacent segments, particularly in the second cervical and fifth lumbar vertebrae, which are susceptible to traumatic and stress fractures in this region (i.e., hangman’s fracture of C21 and isthmic spondylolysis of L5). In sequential alternation with the intervertebral facet joints, it roofs the lateral recesses of the spinal canal and contributes to the dorsal margins of the intervertebral foramina. In the subcervical vertebrae, it also provides the dorsal part of the base of the transverse process.
In the case of the second cervical vertebra (axis) there is a unique anterior relationship of its superior articular facets with the more posteriorly positioned inferior processes that elongates the C2 pars interarticularis. As this offset area receives the greatest leverage between the “cervicocranium” and the lower cervical spine, the indicated line in the illustration in Figure 2–2 shows the common site of mechanical failure in hyperextension injuries to the upper cervical spine.
In the case of the lumbar vertebrae, the pars interarticularis has been subdivided further. McCulloch and Transfelt2 referred to the “lateral buttress,” which they believed offered particular structural support to the intervening structures. They described it as the bony bridge that connects the superolateral edge of the inferior facet to the junction of the transverse process and the pedicle. In a follow-up anatomic study, Weiner and colleagues3 measured the surface area of the lateral buttress in human cadaveric lumbar spines. They found the greatest areas (about 80 mm2) from L1 to L3, whereas area averaged 50 mm2 at L4 and only 15 mm2 at L5. These investigators thought that the broadness of the buttress in the upper lumbar spine can obscure or confuse landmarks for placement of pedicle screws, and its relative thinness (or nonexistence) in the lower lumbar spine can be a predisposing factor to stress fractures or iatrogenic injury to the pars interarticularis.
Regional Characteristics
Although the 24 vertebrae of the presacral spine are divided into three distinct groups (Fig. 2–3), in which the individual members may be recognized by one or two uniquely regional features, there is a gradual craniocaudal progression of morphologic changes. The vertebrae found above and below the point of regional demarcation are transitional and bear some of the characteristics of both areas.
Cervical Vertebrae
Of the seven cervical vertebrae, the first two (Fig. 2–4A to D) and the last require special notation, but the third to the sixth are fairly uniform, and a common description suffices (Fig. 2–4E and F). Because the cervical vertebrae bear the least weight, their bodies are relatively small and thin with respect to the size of the vertebral arch and vertebral foramen. In addition, their diameter is greater transversely than in the anteroposterior direction. The lateral edges of the superior surface of each body are sharply turned upward to form the uncinate processes that are characteristic of the cervical region. The most obvious diagnostic feature of the cervical vertebrae is the transverse foramina that perforate the transverse processes and transmit the vertebral arteries. The anterior part of the transverse processes represents fused costal elements that arise from the sides of the body. The lateral extremities of the transverse processes bear two projections, the anterior and posterior tubercles. The former serve as origins of anterior cervical muscles; the latter provide origins and insertions for posterior cervical muscles. A deep groove between the upper aspects of the tubercles holds the cervical spinal nerves.
The cervical pedicles connect the posterior vertebral arch to the vertebral body. Anatomic studies have shown that the cervical pedicle height ranges from 5.1 to 9.5 mm, and width ranges from 3 to 7.5 mm.4,5 The pedicle is angled medially between 90 and 110 degrees.5
The superior and inferior articular processes appear as obliquely sectioned surfaces of short cylinders of bone that, when united with the adjacent vertebrae, form two osseous shafts posterolateral to the stacked vertebral bodies. The cervical vertebrae present a tripod of flexible columns for the support of the head. As in the upper cervical spine, the combination of the articular processes and the intervening bone is often referred to as the lateral mass in the subaxial region. It is a common site for screw insertion during internal fixation of the cervical spine.6
The laminae are narrow and have a thinner superior edge. At their mid-dorsal junction, they bear a bifid spinous process that receives the insertions of the semispinalis cervicis muscles. The height of the lamina of C4 is 10 to 11 mm, whereas the lamina thickness at C5 is about 2 mm.7 The lamina is thickest at T2, where it measures an average of 5 mm.
Thoracic Vertebrae
All 12 thoracic vertebrae support ribs and have facets for the diarthrodial articulations of these structures. The first and last four have specific peculiarities in the manner of costal articulations, but the second to the eighth are similar (Fig. 2–4G and H).
Because the pedicles arise more superiorly on the dorsum of the body than they do in the cervical region, the inferior vertebral notch forms an even greater contribution to the intervertebral foramen. The pedicle height increases from T1 to T12, but the transverse pedicle width (which is more critical for transpedicular screw containment) does not follow this same craniocaudal pattern.8 Cinotti and colleagues9 found that the pedicles in the T4 to T8 region had the smallest transverse diameter. Scoles and colleagues10 documented similar findings in 50 cadaveric human spines, with the smallest diameters measured at T3 to T6. On average, the transverse pedicle diameter at T3 is 3.4 mm in women and 3.9 mm in men. At T6, it averages 3 mm in women and 3.5 mm in men. At T1, however, the mean diameter is 6.4 mm in women and 7.3 mm in men.
Lumbar Vertebrae
The lumbar vertebrae are the lowest five vertebrae of the presacral column (see Fig. 2–4I and J). All their features are expressed in more massive proportions. They are easily distinguished from other regional elements by their lack of a transverse foramen or costal articular facets. The body is large, having a width greater than its anteroposterior diameter, and is slightly thicker anteriorly than posteriorly. All structures associated with the vertebral arch are blunt and stout. The thick pedicles are widely placed on the dorsolaterosuperior aspects of the body, and with their laminae they enclose a triangular vertebral foramen. Although the inferior vertebral notch is deeper than the superior, both make substantial contributions to the intervertebral foramen. The transverse processes are flat and winglike in the upper three lumbar segments, but in the fifth segment they are thick, rounded stumps. The fourth transverse process is usually the smallest.
Sacral Vertebrae
The sacrum consists of five fused vertebrae that form a single triangular complex of bone that supports the spine and forms the posterior part of the pelvis (Figs. 2–5 and 2–6). It is markedly curved and tilted backward, so that its first element articulates with the fifth lumbar vertebra at a pronounced angle (the sacrovertebral angle).
Arthrology of the Spine
The articulations of the spine include the three major types of joints: synarthroses, diarthroses, and amphiarthroses (Figs. 2-7 to 2-9). The synarthroses are found during development and the first decade of life. The best examples are the neurocentral joints of the immature spine, which are the two unions between the centers of ossification for the two halves of the vertebral arch and that of the centrum. Until they are obliterated during the 2nd decade, they possess a thin plate cartilage between the two apposed bony surfaces. Another example is the early union between the articular processes of the sacral vertebrae, known as ephemeral synchondroses.
Articulations of the Vertebral Arches
This shinglelike arrangement conceals the true length of the ligaments because of the overlapping of the superior lamina. Their morphology is best appreciated from the ventral aspect as in Figure 2–9B. The yellow elastic fibers that give the ligamenta flava their name maintain their elasticity even in embalmed specimens. It has been stated in some texts that the elasticity of the ligamenta flava serves to assist in the maintenance of the erect posture. A more probable reason for this property is simply to keep the ligament taut during extension, where any laxity would permit redundancy and infolding toward the ventrally related nervous structures, as occurs in degenerative lumbar spinal stenosis.
There are two separable layers of the ligamentum flavum, one superficial and one deep, that have distinct attachments to the inferior lamina.11 The superficial component inserts at the classically described location along the posterosuperior aspect of the lamina. The deep component inserts along the anterosuperior surface of the lamina.11 This attachment can have significance during surgical removal of the ligamentum flavum for exposure of the neural elements.
The interspinous ligaments (see Fig. 2–9A) are membranous sets of fibers that connect adjoining spinous processes. They are situated medial to the thin pairs of interspinal muscles that bridge the apices of the spine. The fibers of the ligaments are arranged obliquely as they connect the base of the superior spine with the superior ridge and apex of the next most inferior spinous process. These midline ligaments are found in pairs with a distinct dissectible cleft between them.
The supraspinous ligament (see Fig. 2–9A) is a continuous fibrous cord that runs along the apices of the spinous processes from the seventh cervical to the end of the sacral spinous crest. Similar to the longitudinal ligaments of the vertebra, the more superficial fibers of the ligament extend over several spinal segments, whereas the deeper, shorter fibers bridge only two or three segments. In the cervical region the supraspinous ligament assumes a distinctive character and a specific name, the ligamentum nuchae. This structure is bowstrung across the cervical lordosis from the external occipital protuberance to the spine of the seventh cervical vertebra. Its anterior border forms a sagittal fibrous sheet that divides the posterior nuchal muscles and attaches to the spinous processes of all cervical vertebrae. The ligamentum nuchae contains an abundance of elastic fibers. In quadrupeds, it forms a strong truss that supports the cantilevered position of the head.
Special Articulations
The median atlantoaxial articulation is a pivot (trochoid) joint (Figs. 2–10 and 2–11). The essential features of the articulation are the odontoid process (dens) of the axis and the internal surface of the anterior arch of the atlas. The opposition of the two bones is maintained by the thick, straplike transverse atlantal ligament. The ligament and the arch of the atlas have true synovial cavities intervening between them and the odontoid process. Alar expansions of the transverse ligament attach to tubercles on the lateral rims of the anterior foramen magnum, and a single, unpaired cord, the apical odontoid ligament, attaches the apex of the process to the basion. The entire joint is covered posteriorly by a cranial extension of the posterior longitudinal ligament, which is named tectorial membrane in this region. Because the atlas freely glides over the superior articulating facets of C2, the atlantoaxial pivot is essential for preventing horizontal displacements between C1 and C2. Fracture of the odontoid or, less likely, rupture of the transverse ligament produces a very unstable articulation.
Articulations of the Vertebral Bodies
Intervertebral Disc
In view of the semiliquid nature of the nucleus pulposus and the vacuities that may be shown in the nucleus of aging specimens, von Luschka12 attempted to classify the intervertebral disc as a diarthrosis, in which the vertebral chondral plates were the articular cartilages, the anulus provided the articular capsule, and the fluid and ephemeral spaces within the nucleus corresponded to the synovia and the joint cavity. Although the intervertebral disc forms a joint that should be classified in its own exclusive category because its development, structure, and function are generally different from those of any other joint, it most closely conforms to an amphiarthrosis of the symphysis type.
Anulus Fibrosus
The anulus is a concentric series of fibrous lamellae that encase the nucleus and strongly unite the vertebral bodies (Fig. 2–12). The essential function of the nucleus is to resist and redistribute compressive forces within the spine, whereas one of the major functions of the anulus is to withstand tension, whether the tensile forces be from the horizontal extensions of the compressed nucleus, from the torsional stress of the column, or from the separation of the vertebral bodies on the convex side of a spinal flexure. Without optical aid, simple dissection and discernment reveals how well the anulus is constructed for the performance of this function.
On horizontal section, it is noted that an individual lamella encircling the disc is composed of glistening fibers that run an oblique or spiral course in relation to the axis of the vertebral column. Because the disc presents a kidney-shaped or heart-shaped horizontal section, and the nucleus is displaced posteriorly, these lamellae are thinner and more closely packed between the nucleus and the dorsal aspect of the disc. The bands are stoutest and individually more distinct in the anterior third of the disc, and here when transected they may give the impression that they are of varying composition because every other ring presents a difference in color and elevation with reference to the plane of section. Teasing and inspection at an oblique angle shows in the freed lamellae, however, that this difference is due to an abrupt change in the direction of the fibers of adjacent rings. Previous descriptions of the anulus have claimed that the alternating appearance of the banding is the result of the interposition of a chondrous layer between each fibrous ring.13 In reality, the alternations of glistening white lamellae with translucent rings result from differences in the incidence of light with regard to the direction of the fiber bundles. This repeated reversal of fiber arrangement within the anulus has implications in the biomechanics of the disc, which are discussed later.
The disposition of the lamellae on sagittal section is not consistently vertical. In the regions of the anulus approximating the nucleus pulposus, the first distinct bands curve inward, with their convexity facing the nuclear substance. As one follows the successive layers outward, a true vertical profile is assumed, but as the external laminae of the disc are approached, they may again become bowed, with their convexity facing the periphery of the disc.14,15
The attachment of the anulus to its respective vertebral bodies warrants particular mention. This attachment is best understood when a dried preparation of a thoracic or lumbar vertebra is examined first. In the adult, the articular surface of the body presents two aspects: a concave central depression that is quite porous and an elevated ring of compact bone that appears to be rolled over the edge of the vertebral body. Often a demarcating fissure falsely suggests that the ring is a true epiphysis of the body, but postnatal studies of ossification have indicated that it is a traction apophysis for the attachment of the anulus and associated longitudinal ligaments.16
In life, the depth of the central concavity is filled to the level of the marginal ring by the presence of a cribriform cartilaginous plate. In contrast to other articular surfaces, there is no closing plate of compact osseous material intervening between this cartilage and the cancellous medullary part of the bone. The trabeculations of the spongiosa blend into the internal face of the chondrous plate, whereas fibers from the nucleus and inner lamellae of the anulus penetrate its outer surface. As intimate as this union between the central disc and vertebra may appear, the outer bony ring affords the disc its firmest attachment because the stoutest external lamellar bands of fibers actually penetrate the ring as Sharpey fibers. Scraping the disc to the bone shows the concentric arrangements reflecting the different angles at which the fibers insert (see Fig. 2–12). The fibers of the outermost ring of the anulus have the most extensive range of attachment. They extend beyond the confines of the disc and blend with the vertebral periosteum and the longitudinal ligaments.
Regional Variations of the Disc
The discs in aggregate make up approximately one fourth of the length of the spinal column, exclusive of the sacrum and coccyx. Their degree of contribution is not uniform in the various regions. According to Aeby,17 the discs provide more than one fifth of the length of the cervical spine, approximately one fifth of the length of the thoracic column, and approximately one third of the length of the lumbar region.
The discs are smallest in the cervical spine. Their lateral extent is less than that of the corresponding vertebral body because of the uncinate processes (Fig. 2–13). Here, as in the lumbar region, they are wedge-shaped, the greatest width being anterior, producing lordosis. The thoracic discs are heart-shaped on section, with the nucleus pulposus being more centrally located than in the lumbar region. The thickness and the horizontal dimensions of the thoracic disc increase caudad with the corresponding increase in size of the vertebral bodies. The normal thoracic kyphosis results from a disparity between the anterior and posterior heights of the vertebral bodies because the discs are of uniform thickness. The lumbar discs are reniform and are relatively and absolutely the thickest in the spine. The progressive caudal increase in the degree of lumbar lordosis is due to the equivalent increase in the differential between the anterior and posterior thickness of the disc.
The cervical intervertebral discs have been a source of controversy because of the so-called joints of Luschka, or uncovertebral joints. These articular modifications are found on both sides of the cervical discs as oblique, cleftlike cavities between the superior surfaces of the uncinate processes and the corresponding lateral lips of the interior articular surface of the next superior vertebra. Because they initially appear in the latter part of the first decade and are not universally demonstrable in all cervical spines, or even in all subaxial discs of the same cervical spine, it is preferable to call them “accommodative joints” that have developed in response to the shearing stresses of the torsions of cervical mobility (see Fig. 2–13).
Spinal Ligaments
Anterior Longitudinal Ligament
The anterior longitudinal ligament is a strong band of fibers that extends along the ventral surface of the spine from the skull to the sacrum. It is narrowest and cordlike in the upper cervical region, where it is attached to the atlas and axis and their intervening capsular membranes. It widens as it descends the column to the extent, in the lower lumbar region, of covering most of the anterolateral surfaces of the vertebral bodies and discs before it blends into the presacral fibers. The anterior longitudinal ligament is not uniform in its composition or manner of attachment. Its deepest fibers, which span only one intervertebral level, are covered by an intermediate layer that unites two or three vertebrae and a superficial stratum that may connect four or five levels. Where the ligament is adherent to the anterior surface of the vertebra, it also forms its periosteum. It is most firmly attached to the articular lip at the end of each body. It is most readily elevated at the point of its passage over the midsection of the discs, where it is loosely attached to the connective tissue band that encircles the anulus (Fig. 2–14).
Posterior Longitudinal Ligament
These fibers are most firmly fixed at the margins of their lateral expansions. This produces a central rhomboidal area of loose attachment, or in some cases an actual fascial cleft of equivalent dimensions, on the dorsolateral aspect of the disc. At dissection, this characteristic may be readily shown by inserting a blunt probe beneath the intervertebral part of the longitudinal ligament and exploring the area to define the margins of the space where the fibers are strongly inserted (Fig. 2–15). This situation is particularly pertinent to problems involving dorsal or dorsolateral prolapse of the nucleus pulposus. With a dorsocentral protrusion of a semifluid mass, the strong midline strap of posterior longitudinal fibers tends to restrain the herniation. If an easily dissectible cleft offers a space for lateral expansion, however, the mass can extend to either side, dissecting the loose attachments.
Although not frequently included in anatomic discussions of the spine, an additional structure travels deep to the posterior longitudinal ligament, extending laterally and posteriorly to surround the dura of the cauda equina. It has been termed the peridural membrane, first by Dommissee in 197518 and later by Wiltse.19 The basivertebral veins cross the peridural membrane because it offers no obstruction to vascular communication between the intraosseous vessels of the vertebral body and the epidural space. Its possible clinical significance is that it may provide a containing membrane for herniated discs or hematomas, which may be noted on advanced imaging such as computed tomography (CT) or magnetic resonance imaging (MRI) as a delimiting barrier to the pathology.
Relationships of the Roots of the Spinal Nerves
The nerve root is intimately related to the pedicle of the vertebra. Ugur and colleagues20 found no distance between the upper cervical pedicles and their corresponding nerve roots in 20 cadaveric spines, whereas there was a slight distance in 4 of the 20 specimens in the lower cervical region. For all specimens, the distance from the nerve root to the inferior aspect of the upper pedicle ranged from 1 to 2.5 mm. The distance from the medial aspect of the pedicle to the dural sac ranged from 2.4 to 3.1 mm. A similar relationship between the thoracic nerve roots and pedicle exists.21 The distance from the pedicle to the superior nerve root in the thoracic spine ranges from 1.5 to 6.7 mm, and the distance from the pedicle to the inferior nerve root, 0.8 to 6 mm. Ebraheim and colleagues22 measured these distances in the lumbar spine, finding a mean distance of 1.5 mm from the pedicle to the inferior nerve root, 5.3 mm from the pedicle to the superior nerve root, and 1.5 mm from the medial pedicle wall to the dura.
Of particular interest is the distribution of epidural fat around and within the intervertebral foramen. This fat has a firm character and forms a mechanically supportive “bushing” for structures entering and leaving the spinal canal. A prominent extension of this fat body also follows the inferior and ventral surfaces of each lumbar nerve. It is interposed between the root and the external surfaces of the pedicle and vertebral body that define the inferior part of the intervertebral foramen. Its amelioration of the downward and ventral distraction of the nerve that accompanies the spine and lower limb motions is obvious. Histologically, it is composed of uniform cells that are contained within a fine membrane (perhaps the elusive peridural membrane).23 There is no fibrous tissue in normal epidural fat and only tenuous attachments to the dura.
Intervertebral Foramen
The intervertebral foramen is the aperture that gives exit to the segmental spinal nerves and entrance to the vessels and nerve branches that supply the bone and soft tissues of the vertebral canal. It is superiorly and inferiorly bounded by the respective pedicles of the adjacent vertebrae. Its ventral and dorsal components involve the two major intervertebral articulations. The dorsum of the intervertebral disc, covered by the lateral expansion of the posterior longitudinal ligament, provides a large part of its ventral boundary, whereas the joint capsule of the articular facets and the ligamentum flavum contribute the major parts of its dorsal limitation. Along with the root, the remaining space is filled with loose areolar tissue and fat (Fig. 2–16).
However ample the overall dimensions of the intervertebral foramen may be, its elliptical nature is responsible for many of its relational problems. In the lumbar region, the vertical diameter of the foramen ranges from 12 to 19 mm; this undoubtedly accounts for the fact that a complete collapse of the disc may produce little or no evidence of nerve compression. The sagittal diameter may be only 7 mm, however, making this dimension exquisitely sensitive to changes. Because the diameter of the fourth lumbar nerve can be just slightly less than 7 mm, the tolerance for pathologic alteration of the bony or connective tissue relationships is restricted.24
The existence of additional ligamentous elements in relation to the intervertebral foramen could limit further the space for the exiting spinal nerve. These structures, known as the transforaminal ligaments, are frequently found in the lumbar region.25,26 The transforaminal ligaments are strong, unyielding cords of fibrous tissue that pass anteriorly from various parts of the neural arch to the body of the same or the adjacent vertebra and may be 5 mm wide. Grimes and colleagues27 found these ligaments span from the nerve root itself. These investigators noted four different bands, the most significant of which spread from the nerve root to the anterior aspect of the facet capsule. Other bands spanned from the nerve root to the superior pedicle, the inferior pedicle, and the intervertebral disc anteriorly.
In the cervical spine, the space available for the exiting nerve root may be compromised by structures just lateral to the foramen. In 10 adult human cadaveric specimens, Alleyne and colleagues28 found the dorsal root ganglia of the C3 to C6 spinal nerves to be slightly compressed by the ascending vertebral artery. This compression was most pronounced at the C5 level, which the authors suggested as a possible explanation for the greater susceptibility of this nerve to iatrogenic injury during procedures such as laminoplasty.
Lumbosacral Nerve Root Variations
The most common variation involves atypical origins, or foraminal exits, of individual lumbosacral roots. Although myelographic studies indicated only a 4% incidence of lumbosacral root anomalies, an anatomic study by Kadish and Simmons29 reported an incidence of 14%. The L5-S1 level is the most commonly involved. Observations by these authors provided four types of variations: (1) intradural interconnections between roots at different levels, (2) anomalous levels of origin of nerve roots, (3) extradural connections between roots, and (4) extradural division of nerve roots.
A source of confusing neurologic findings may relate to the variant anatomy of the furcal nerve. The name furcal nerve has been applied to the fourth lumbar nerve because it exhibits a prominent bifurcation to contribute to the lumbar plexus (femoral and obturator nerves) and sacral plexus (lumbosacral trunk). Kikuchi and Hasue30 found that it is often indefinite in its intradural affinities, frequently exhibiting two dorsal root ganglia that have distinct root sources at the conus medullaris. They proposed that when symptoms indicate the involvement of two levels, suspicion should be directed toward four possible causes: (1) two roots compressed by a single lesion, (2) the presence of two lesions, (3) the anomalous emergence of two roots through the same foramen, or (4) the existence of the peculiarly doubled components of the furcal nerve (Fig. 2–17).
Infrequently, variant “fixation” alters the expected sequences of nerve root exit. In a prefixed lumbosacral plexus, the furcal nerve (the division between the lumbar and sacral plexuses) exits through the third lumbar foramen, and the preceding and subsequent nerves exit one vertebral level higher than in the conventional distribution. Conversely, in the postfixed plexus, the furcal nerve exits the L5-S1 foramen, and the lumbosacral nerve sequence is all one level lower than usually described.31
Although Kadish and Simmons29 noted that the existence of anomalous interconnections between nerve root levels dispels any notion of “absolute innervation,” Parke and Watanabe32 showed that there is a consistent system of intersegmental connections between the roots of the lumbosacral nerves. They described an epispinal system of motor axons that courses among the meningeal fibers of the conus medullaris and virtually ensheathes its ventral and lateral funiculi between the L2 and S2 levels. These nerve fibers apparently arise from motor neuron cells of the ventral horn gray matter and join spinal nerve roots caudal to their level of origin. In all the spinal cords studied, many of these axons commingled at the cord surface to form an irregular group of ectopic rootlets that could be visually traced to join conventional spinal nerve roots at one to several segments inferior to their original segmental level (Figs. 2–18 and 2–19). Occasionally, these ventral ectopic rootlets course dorsocaudad to join a dorsal (sensory) nerve root. Although the function and the clinical significance of this epispinal system of axons have yet to be explained, a given segmental level of motor nerve cells may contribute fibers not only to an adjacent segment, but also to nerve roots of multiple inferior levels.
< div class='tao-gold-member'>
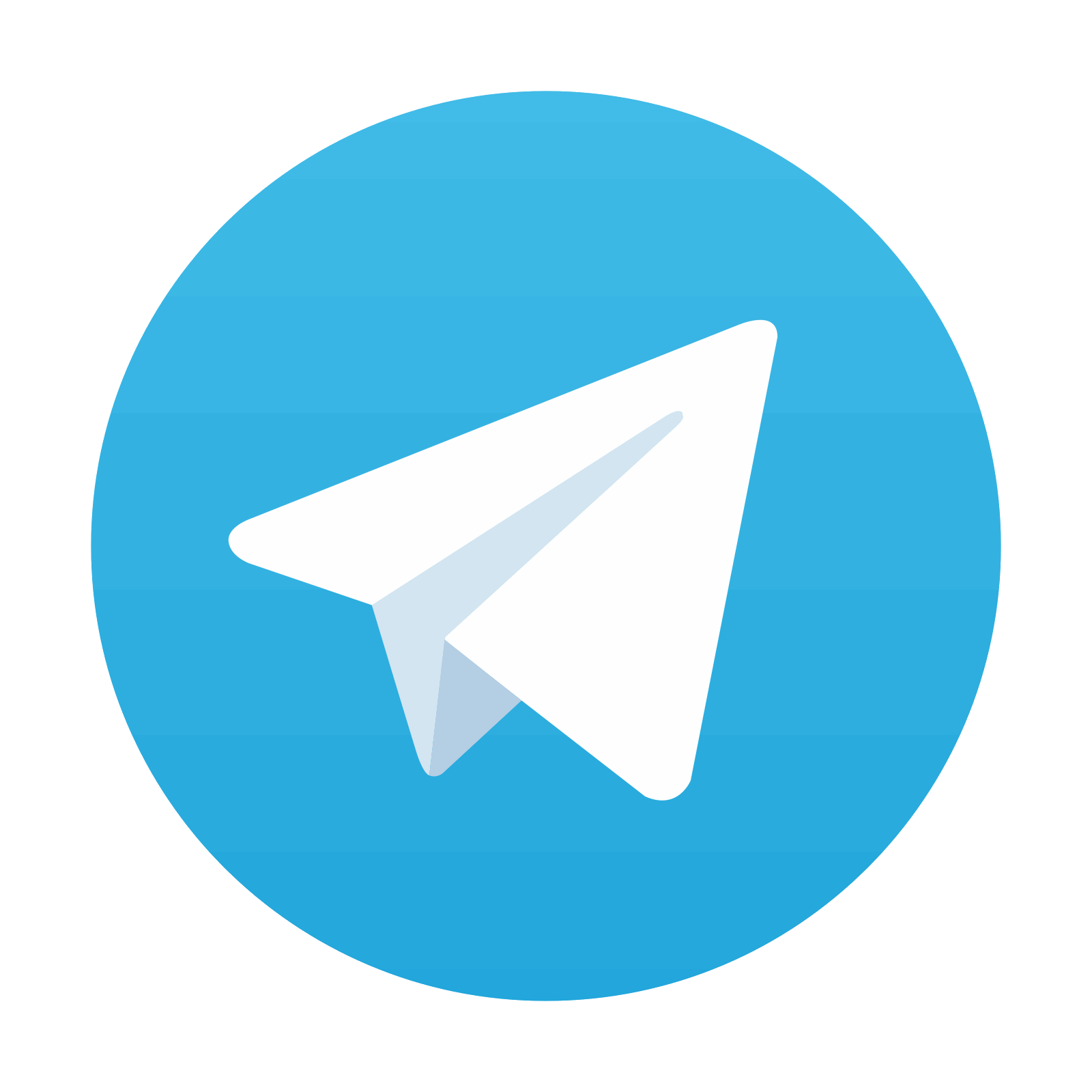
Stay updated, free articles. Join our Telegram channel

Full access? Get Clinical Tree
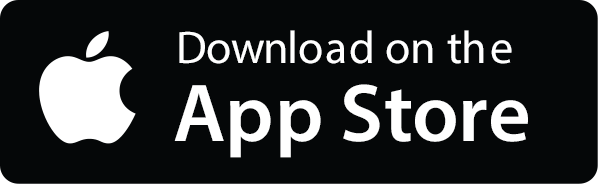
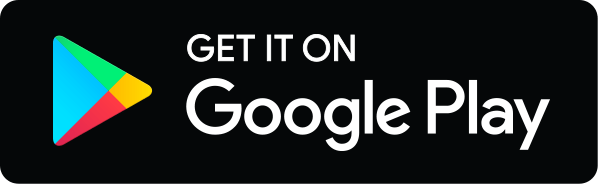