Fig. 33.1
(a) Gross specimen of a young adult pig meniscus on the tibial plateau. The meniscus surface appears smooth and glossy white. (b) Illustration showing cell types and collagen ultrastructure in each zone. (c) Histology of pig meniscus stained with picrosirius red under polarized microscope. White arrows indicate radial tie fibers. (d) Transmission electron microscopy images of the collagen fiber. (e) Histologies of pig meniscus stained with safranin-O/fast green. (f) Transmission electron microscopy images of the cells in three zones: superficial zone in avascular area, deep zone in avascular area, and vascular area. (b) Reprinted with permission from Kawamura S, Lotito K, Rodeo S.: Biomechanics and Healing Response of the Meniscus. Operative Techniques in Sports Medicine 2003; 11 (2): 68–76. (e, f) Reprinted with permission from Nakagawa Y, Sekiya I Kondo S, Tabuchi T, Ichinose S, Muneta T, et al., et al.: Relationship Between MRI T1rho Value and Histological Findings of Intact and Radially Incised Menisci in Microminipigs. J Magn Reson Imaging 2016; 43 (2): 434–45
The meniscus has a unique collagen structure orientation that is related to its function and consists of three different patterns of collagen fiber orientation: circumferential, radial, and random (Fig. 33.1b–d) [5]. Circumferential fibers are the most abundant in the tissue and are seen in the deep zone. Radial fibers are dispersed throughout the deep zone and are present on the periphery. Thus, the meniscus has anisotropic tensile properties. Tensile strength in the circumferential directions is much higher than that in radial directions. In the superficial zone, the fiber orientation is typically random.
Although the meniscus is mainly fibrocartilage tissue, there are zonal differences in the biochemical and ultrastructural composition within the meniscus. Meniscus cell population is classified into three types according to the different regions and cell morphology: superficial zone cells, fibrochondrocytes in the deeper zone in the avascular area, and fibroblastic cells in the vascular area (Fig. 33.1b, e, f) [6]. Recently, it has been found that the superficial zone contains a population of stem cells [7]. These cells are fusiform and are aligned parallel to the meniscal surface. These cells may contribute to tissue homeostasis and repair/regeneration. Fibrochondrocytes are predominantly oval to round in appearance similar to chondrocytes and can produce a large amount of type I collagen and lower but significant amounts of type II collagen and proteoglycan. These cells are responsible for synthesizing and maintaining the extracellular matrix. Fibroblastic cells have long cell extensions which are in contact with other cells as well as the extracellular matrix.
33.3 Tissue Engineering for Meniscus
33.3.1 Scaffold
The term “tissue engineering” refers to the combination of cells and/or chemical signals with a physical scaffold with the aim of host target tissue regeneration (Table 33.1). Various tissue grafts have been used for meniscus replacement, including human meniscal allograft, small intestinal submucosa (SIS) implants, and autogenous tendon grafts. Milachowski and Wirth performed the first free meniscal allograft transplantation in 1984 [8]. Currently meniscal transplantation is considered as an alternative treatment option for selected symptomatic patients who had previous complete or subtotal meniscectomy. Meniscus allograft transplantation improves knee function and reduces pain significantly in relatively young patients after short- and medium-term follow-up (Fig. 33.3) [9, 10]. However, whether meniscal allografts provide long-term protective benefits to the cartilage remains debatable, as the tissue can degenerate over time, leading to weakening of the material properties, extrusion, and graft shrinkage [11]. SIS and autogenous tendon grafts have not obtained satisfactory results [12, 13].

Table 33.1
Scaffolds, signals, and cells for meniscus tissue engineering

In regard to artificial scaffolds, biological and synthetic materials for meniscal tissue regeneration have specific inherent advantages, respectively. The main advantage of a biological meniscus scaffold is that they typically contain bioactive factors that may promote desirable cellular functions such as cell adhesion, proliferation, and differentiation. Furthermore, they are derived from natural materials which generally are highly biocompatible, and they are rapidly degraded. On the other hand, disadvantages include batch-to-batch variability, the possibility of pathogen transfer, inferior mechanical properties, and limited control over physiochemical properties. In addition, natural materials are complex and not well characterized. Thus, it is difficult to determine the mechanistic basis of their effects on cell function. Balint et al. [14] have reported the use of a collagen scaffold reinforced by a network of degradable tyrosine-derived polymer fibers that has demonstrated circumferential tensile strength, stiffness, and hoop stress behavior under compressive loading that mimics the normal meniscus.
Bioabsorbable synthetic polymers, such as polyurethane (PU), polyglycolic acid (PGA), polylactic acid, and poly (?-caprolactone) (PCL), are widely used and have played a key role in fabrication of meniscal scaffolds [15]. These polymers provide several advantages, such as versatility, satisfactory biomechanical properties, and potential availability as an “off-the shelf” implant. However, some disadvantages of synthetic polymers include their hydrophobic properties, lack of bioactivity, and production of aseptic inflammation or an immune response.
Hydrogels (natural or synthetic) are biochemically versatile and can be used as cell and growth factor delivery systems. Although their biomechanical properties can be tailored somewhat by substrate concentration and composition, they often fall short of native meniscal properties. To overcome this problem, Holloway et al. [16] proposed a fiber reinforced composite containing ultra-high molecular weight (UHMWPE) fibers in a poly-vinyl alcohol (PVA) hydrogel and varied the composition and orientation of the fibers to mimic native meniscal properties for total meniscal replacement. Upon implantation in a dynamic cadaveric model, this construct was found to restore contact mechanics to a comparable level as allogenic meniscus transplantation.
33.3.2 Cell Sources
Cell therapy represents a promising interventional strategy for promoting the functional regeneration of damaged tissues as well as for augmentation of scaffold-based approaches. Cells used for transplantation can be categorized as differentiated or stem cells.
Meniscus cells (fibrochondrocytes) and chondrocytes are differentiated cells that are mainly used for meniscus regeneration. Both of these cell types have been successfully incorporated into cell-scaffold complexes and demonstrate promising results in both in vitro and in vivo studies. The overwhelming issue with these cell types is availability. The use of autologous meniscus cells and chondrocytes requires a two-step operative process, to first harvest the tissue, followed by cell isolation and culture, and then subsequent surgical implantation.
Regarding stem cells, mesenchymal stem cells (MSCs) are the most frequent cell type studied in both basic and clinical studies due to the fact that they are easily accessible from peripheral connective tissue sites such as bone marrow [17], periosteum, adipose, and the synovium [18]. Additionally, they have been shown to have a good safety profile. The expanding scientific interest in these cells is owing to two main factors [19]. First, MSCs have been observed to differentiate into many terminally differentiated cell types, including osteoblasts, chondrocytes, adipocytes, and myocytes, and thus they represent an attractive potential means of regenerating damaged connective tissues including intra-articular structures of the knee, such as the meniscus. Second, MSCs secrete a large variety of cytokines and other trophic support factors and contribute to the healing process of injured tissue by providing paracrine trophic mediators. The transplantation of expanded or modified MSCs is a promising approach for biologic augmentation of meniscus healing. Recent literature has suggested that synovial tissue-derived mesenchymal stem cells may have the potential to aid in healing and regeneration of cartilage injuries, such as those involving the meniscus [20, 21]. Synovial mesenchymal stem cells represent an attractive cell source because they can be harvested in a minimally invasive manner from synovial tissue and are easily expanded in culture. In addition, multiple investigators have found that in vitro synovial mesenchymal stem cells possess a particularly high capacity for chondrogenic differentiation and proliferation compared with mesenchymal stem cells obtained from other tissues, such as bone marrow or periosteum [22]. Nakagawa et al. [23] showed that transplantation of synovial MSCs promoted healing of meniscal repair with the induction of synovium into a longitudinal tear in the avascular zone in the porcine meniscus. Ozeki et al. [24] demonstrated that synovial MSCs enhanced meniscus regeneration after autologous tendon transplantation in a partial meniscectomy model and also prevented development of osteoarthritis.
Embryonic stem cells and induced pluripotent stem cells have proven to be an emerging cell source for fibrocartilage tissue engineering. Some of the main characteristics which make this cell source ideal for tissue engineering are pluripotency and ultimate proliferative capacity. Attempts toward tissue engineering the meniscus using these cell sources are still in the early phase.
33.3.3 Chemical and Physical Signals for Meniscus Tissue Regeneration
The delivery of biophysical and biochemical signaling is intended to stimulate endogenous repair mechanisms by recruiting cells from the host instead of requiring the survival of implanted exogenous cells. The cues for tissue-engineering applications typically are categorized as recombinant proteins (growth factor), hormones, and small molecules. Several in vitro and in vivo studies have evaluated the effects of treatment with specific growth factors on meniscus [25]. For instance, basic FGF (bFGF) was used to stimulate type II collagen and aggrecan mRNA production in meniscal cells. In an ovine experimental model, meniscal fibrochondrocytes responded to bFGF by proliferating and producing new ECM [26]. Fibroblast growth factor–2 has been shown to recover the monolayer expansion of meniscal cells. Platelet-derived growth factor-AB (PDGF-AB) plays an important role in angiogenesis and cell development. The application of PDGF-AB in the peripheral part of the menisci resulted in a better healing response than the application in the central part [27]. However, this anabolic growth factor increased both cell proliferation and ECM formation in all zones of the meniscus, including the avascular zone. PDGF-AB also enhances meniscal cell migration. Growth factor supplementation has demonstrated improved cellular migration, proliferation, and matrix production and overall improved cell differentiation, but important translational considerations include determining the optimal mode of delivery and localization of cytokine effect to the desired site. Currently there is a focus on optimizing the timing of delivery and the release profile of these different biologic regulators.
Platelet-rich plasma (PRP) is an autologous substance rich in platelets that release a wide variety of growth factors from both alpha and dense granules. PRP contains PDGF, VEGF, TGF-β, IGF-1, b-FGF, HGF, and EGF [28]. It is likely that PRP could play a positive role in meniscus tissue engineering, but further studies are required.
In recent years, the use of bone marrow aspirate concentrate (BMAC) has become an increasingly popular method of augmenting bone [29] and cartilage regeneration [30]. BMAC is obtained through density gradient centrifugation of bone marrow (BM) typically aspirated from the iliac crest. Like platelet-rich plasma (PRP), BMAC includes platelets and growth factors that may contribute to its therapeutic potential. Unlike PRP, human BMAC also includes mesenchymal stem cells (MSCs) [31].
33.4 Meniscus Replacement and Regeneration
33.4.1 Meniscal Allograft Transplantation (MAT)
The aims of a meniscal replacement are to diminish pain and swelling in patients following meniscectomy, to prevent or delay osteoarthritis progression, and to improve the stability of the knee joint. Meniscal allograft transplantation (MAT) is the only current therapeutic option for total meniscal replacement. Two types of meniscal allografts are currently used for meniscal replacement: fresh (with viable cells) and frozen. Lyophilized allografts are no longer used owing to the structural alteration and shrinkage caused by this preservation technique. Frozen allografts are currently the most commonly used tissue due to easier tissue availability (Fig. 33.2) [32]. The comparative studies that have been performed have not shown a clear advantage to use of fresh meniscus tissue [33].


Fig. 33.2
Meniscus allograft transplantation (MAT). (a) Scheme showing the surgical procedure of MAT. (b) Allograft with bone plug attached to anterior and posterior horns. (c) Arthroscopic picture at time of transplantation. Left, posterior side of plug was inserted into bone tunnel. Right, graft was fixed to joint capsule by inside-out suture technique. (d) MRI (coronal and sagittal view) of lateral meniscus allograft at follow-up. White arrow indicated the extrusion of graft. (a) Reprinted with permission from H. Wang, T. Chen, A.O. Gee, I.D. Hutchinson, S.A. Rodeo, S.A. Maher, et al.: Altered regional loading patterns on articular cartilage following meniscectomy are not fully restored by autograft meniscal transplantation. Osteoarthritis and Cartilage 2015; 23: 462–468
The indications for meniscal transplantation are strict. This procedure is generally limited to patients aged under 50 years, with a stable, well-aligned knee, minimal arthrosis and with significant pain. The degree of OA present has a decisive effect on the final result, and this procedure is contraindicated in severe osteoarthritis. In patients with instability of the knee and indications for meniscal graft, it is necessary to stabilize the joint by ligament reconstruction prior to or concomitant with meniscus transplantation; in the case of malalignment, corrective osteotomy is required. Basic principles for surgeons performing this procedure include achieving or re-establishing normal knee alignment and stability; implanting a size-matched, non-irradiated graft with secure fixation of the meniscal horns; secure fixation of the meniscus to the capsule; and ensuring patients return only to moderate sports activities to maximize the chances for graft survival. Overall, the majority of clinical studies report improvement in knee symptoms in the short and mid-term, but there is very little data to support a chondroprotective effect of meniscus transplantation [32]. This procedure is currently seen as an interim approach to joint preservation, for young, meniscus deficient patients for whom we have no other good options.
The limitations associated with meniscus allografts include limited graft availability, potential for pathogen transmission, immune rejection, and anatomical mismatch. In addition, degenerative tear, transplant extrusion, and shrinkage may occur, all of which may affect the long-term function of the transplant. Meniscal tears usually occur at the posterior horn attachment site or at the central, avascular region of the tissue, and biomechanical factors such as high contact stresses are a possible causative mechanism. Shrinkage and size reductions in the area of protected tibial cartilage may be a major problem as well. Several studies have described shrinkage after MAT; however, no study has specifically quantified the rate or degree of shrinkage that occurs. Lee et al. [34] reported that gross morphologic alterations, as determined by width and thickness, were observed during the first postoperative year with substantial shrinkage in the mid-body which occurred progressively for 1 year in 16.1% of the cases, but had no association with the short-term clinical outcomes. Extrusion of the meniscus has also been reported as a complication after meniscus transplantation. Varying degrees of graft shrinkage, degeneration, and extrusion seen with MRI often do not correlate with symptoms. For example, most clinical studies have not found that extrusion affects the clinical outcome scores in the early and medium-term follow-up; however, there is concern that such extrusion will lead to an increased risk of osteoarthritis. Graft extrusion after MAT may be affected by horn fixation, which differs between medial and lateral meniscus transplantation.
33.4.2 Meniscal Scaffolds in Current Clinical Use
There are two meniscal artificial scaffolds available for clinical use in the European Union (EU): one is a biological scaffold, the collagen meniscus implant (CMI, ReGen Biologics, Franklin Lakes, NJ, USA) (Fig. 33.3a). Another one is a synthetic scaffold (Actifit, Orteq Limited, London, UK), which is made from polyurethane and polycaprolactone (Fig. 33.3b). The indication for both scaffolds is the treatment of painful, irreparable partial meniscal defects in the medial or lateral meniscus, as both implants require an intact peripheral vascularized rim and horns for fixation. Both constructs are biodegradable and facilitate tissue regeneration by providing an appropriate pore size for infiltrating host cells derived from synovium to support new meniscal tissue formation.


Fig. 33.3
Currently clinical available meniscus scaffolds. (a) Collagen Meniscus Implant (CMI; ReGen Biologics, Franklin Lakes, NJ, USA). Transmission electron microscopy. (b) Actifit (Orteq Limited, London, UK). Transmission electron microscopy. (c) NUsurface (Active Implants, Memphis, Tennessee)
The collagen meniscus implant is a scaffold that is composed of type I collagen and glycosaminoglycans (GAGs), including chondroitin and hyaluronic acid. It is chemically cross-linked with formaldehyde and sterilized using gamma radiation. Animal studies demonstrate that tissue ingrowth begins superficially and the maturation process progresses into the deeper layers, with active fibrovascular infiltration from the synovium. Patient biopsies from clinical studies have shown capillary ingrowth and progressive replacement of the CMI with immature collagen, demonstrating that CMI functions as a meniscal scaffold with remodeling within the construct [35]. At 5-year follow-up, Steadman and Rodkey showed fibrocartilage and organized extracellular matrix in all of the patients that they biopsied without evidence of immune reaction, inflammation, or infection [36]. In regard to clinical outcome, Zaffagnini et al. [37] and Monllau et al. [38] demonstrated significant improvement in pain and function without development or progression of degenerative knee disease in most patients, despite implant shrinkage at a minimum follow-up of 10 years.
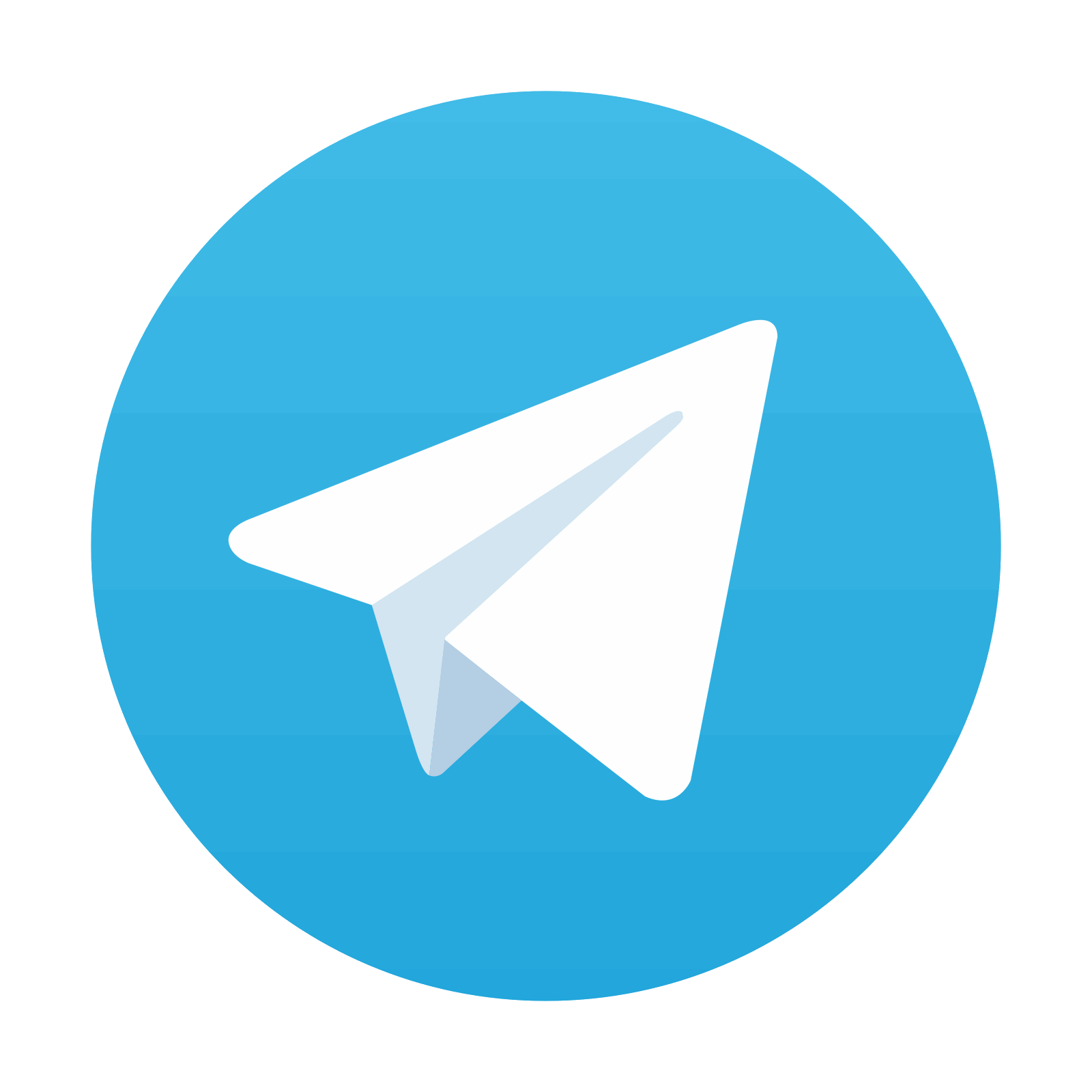
Stay updated, free articles. Join our Telegram channel

Full access? Get Clinical Tree
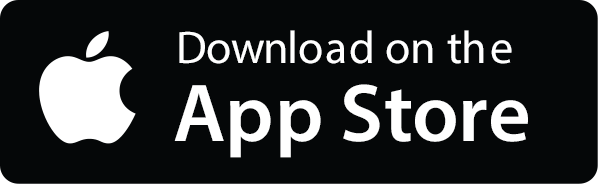
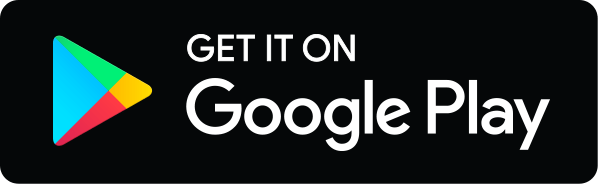