6 WBV as a warm-up prior to sport
Effects on flexibility
Whole body vibration and the effect on flexibility: a review
Nazarov and Zilinsky (1984) were the first researchers to start to experiment with the effect of vibration training (VT) on flexibility. They demonstrated that vibration stretching could increase the range of motion (ROM) in the shoulder of male gymnasts. Other authors followed these interesting preliminary results.
Issurin et al (1994) tested the effect of vibratory stimulation training on maximal force and flexibility in athletes. Twenty-eight male physical education students, aged between 19 and 25 years, underwent a localized vibration training, 44 Hz and 3-mm amplitude, applied through cables during exercise for three times a week for 3 weeks. The subjects stretched during the application of vibration by placing their foot onto a ring suspended from an overhead vibrating device. The vibrating ring provided stimulation for the leg in the ring while the subject stretched the leg. Each subject placed their foot into the ring and stretched for 6–7 s, which was followed by 3–4 s of rest, and this was repeated two to four times. The subjects also performed static stretching exercises with the same parameters as the vibration stretching. The group who underwent VT had a mean increase of 8.7% in the legs split compared with a mean average of 2.4% increase in the conventional group and only 1.2% in the placebo group. An even bigger result was seen for the trunk flexion component. The flexibility group showed a mean gain of 43.6% compared with 19.2% in the conventional group and 5.8% in the placebo group. Issurin et al (1994) concluded that vibration exercise resulted not only in an increase in the maximal strength, but also in increase in flexibility, much greater than in conventional training procedures. They stated also that the gain rate in strength, seen in their research, differed from the gain rate in flexibility during vibration stimulation. Issurin et al (1994) also measured what is called the flex-and-reach test (Lycholat 1990), which measures the distance between the fingertips and a horizontal mark at foot level when subjects flex their trunk–hip joint forward. Obviously this test includes the flexibility of several joints of the trunk and therefore this may result in a larger increase in ROM when compared with other studies where only one part of the body, or one joint, is tested. The increased flexibility measured by the author ranged from 10.9 cm, before training, to 15.65 cm after VT. As an underlying mechanism, for the effect on flexibility, they proposed that the Golgi tendon organs, which are sensitive to active tension (via Ib pathways), stimulated from the vibration, would evoke an autogenic inhibition of contraction resulting in relaxation of the muscle. They postulated also that vibration of contracting muscles would prevent squeezing of the capillaries which normally occurs during muscle contraction. Hence, the rhythmical oscillation of the vibration would elicit a mechanical pump effect on the intramuscular tissue and therefore cause an increase in blood flow and enhance the local muscle temperature. They concluded by suggesting that the alleviation of pain by vibration may also be a component during the active stretching regimens which elicits such good results.
Van den Tillaar (2006) assessed whether WBV training on a vibration platform would have a positive effect on flexibility training (contract–relax method) by enhancing the ROM of the hamstring musculature, which is a muscle group where frequent muscle strains occur. Nineteen physical education students (12 women and 7 men, mean age 21.5 ± 2.0 years) were randomly allocated to either a WBV group or a control group. Both groups underwent a 4-week programme, where they were stretching, according to the contract–relax method (three times with a 5-s isometric contraction on each leg followed by 30 s of static stretching), three times per week. Before each stretching exercise the WBV group would stand on a vibration platform, Nemes Bosco system (28 Hz with 10-mm amplitude) for 30 s, six times per training session in a squat position with knees bent at 90° of flexion. The result demonstrated a significant increase in hamstring flexibility by 14% in the control group and 30% in the WBV training group.
The authors explained their results with three possible mechanisms. One possibility would be enhanced local blood flow which takes place immediately (9 min) after VT (Kerschan-Schindl et al 2001). Increased blood flow can generate additional heat which can facilitate ROM during stretching exercise because muscle elasticity is enhanced. The second mechanism may be related to the tonic vibration reflex. Vibration can cause soft-tissue deformation which is capable of activating muscle spindles (Wakeling et al 2003) and may lead to an enhancement of the stretch–reflex loop (Cardinale & Bosco 2003). The frequency used in the present study was 28 Hz, which reflects the natural frequency of the quadriceps (Wakeling et al 2003), and therefore the authors hypothesized that the stretch reflex of the quadriceps muscles was stimulated to damp the induced frequency. Cardinale and Lim (2003) demonstrated that WBV transmitted through a vibrating platform at 30 Hz, in half-squat position, was able to produce the highest electromyograph (EMG) activity in vastus lateralis of the quadriceps compared with non-vibrating conditions. Cardinale and Bosco (2003) suggested that VT appears to inhibit activation of the antagonist muscles through Ia-inhibitory neurones. Thus, activating the quadriceps muscles would relax the hamstring muscles and thereby have a positive influence on the stretching exercise. Cardinale and Lim (2003) also stated that vibrations were perturbations perceived by the central nervous systems which modulates the stiffness of the stimulated muscle groups. The reflex muscle activity could then be considered a neuromuscular tuning response to minimize soft-tissue vibrations. These responses are individual and therefore related to individual capabilities in damping external perturbations in order to avoid resonance effects. The third mechanism mentioned by Van den Tillaar (2006) is the proprioceptive feedback potentiation of inhibition of pain and increased pain threshold. As stated already by Issurin et al (1994), subjects reported that the sensation of pain was reduced within 10–15 s of beginning the static stretching during vibration.
Sands et al (2006) in their study wanted to determine whether vibration-aided static stretching could enhance ROM more than static stretching alone, in the forward split position. Ten young male gymnasts, participating in intensive gymnastics training (age = 10.1 ± 1.5 years), were randomly assigned to experimental (n = 5) and control (n = 5) groups. The test consisted of performing what is called the ‘forward split position’ for stretching on the vibration devices in two different positions. In the first position the athlete places their forward leg on the vibrating device such that the posterior calf area is supported by the device (Figure 6.1). In the second position the gymnast assumes a lunge position with the rear thigh directly on top of the vibrating device (Figure 6.2). The height of the anterior iliac spine of the pelvis was measured at the lowest split position.
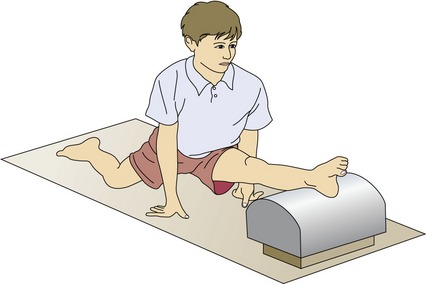
Figure 6.1 Forward split stretching position on the vibration device.
The targeted leg in this position is the forward leg.
Reprinted from Sands WA, McNeal JR, Stone MH et al (2006) Flexibility enhancement with vibration: acute and long-term. Medicine and Science in Sports and Exercise 38:720–725, with permission.
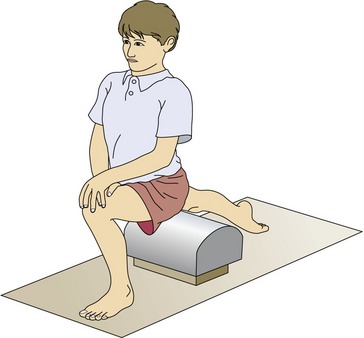
Figure 6.2 Forward split stretching position on the vibration device.
The targeted leg in this position is the rear leg.
Reprinted from Sands WA, McNeal JR, Stone MH et al (2006) Flexibility enhancement with vibration: acute and long-term. Medicine and Science in Sports and Exercise 38:720–725, with permission.
The protocol consisted in stretching of the forward and rear legs to the point of discomfort for 10 s followed by 5 s of rest, repeated four times on each leg (right and left) resulting in 1 min of total stretching in each position, for a total of 4 min for one complete session of stretching. The experimental group stretched with the device turned on; the control group stretched with the device turned off. The devices were floor units which consisted of a heavy base to which was attached an upper section that was vibrated by an electric motor that resulted in a sinusoidal vibration frequency of approximately 30 Hz and an approximate displacement of 2 mm. A pre-test was followed by an acute phase post-test; thereafter, a second post-test measurement was performed following 4 weeks of treatment. The acute effect of the vibration treatment resulted in immediate and dramatic increases in forward split flexibility for both legs (p < 0.05). The long-term effects showed that one split side reached a statistically significant increase in ROM only on the right rear leg (p < 0.05), whereas the other did not. Sands et al (2006) stated in their analysis that the lack of statistical significance on one side during the long-term post-test may have been the result of the increased variability of the splits observed in the control group. The present study is similar to that of Issurin et al (1994) regarding the period of time and the protocol of stretching used. Sands et al (2006) concluded the study, mentioning that vibration effects on range of motion enhancement are incompletely understood and may provide a window into further understanding of the role of muscle spindles, Golgi tendon organs and the importance of higher central nervous system influence on polysynaptic reflexes, and other aspects of motor control.
Kinser et al (2008) wanted to test the effects of simultaneous vibration stretching on flexibility and explosive strength in competitive female gymnasts. Twenty-two female athletes (age = 11.3 ± 2.6 years) composed the experimental vibration stretching (VS) group, which performed two tests: flexibility and jumping. There were four control groups whose constituents were subpopulations of the VS group who did stretching only (SF) (n = 7) and vibration only (VF) (n = 8). Explosive strength-control groups were stretching only (SES) (n = 8) and vibration only (VES) (n = 7). The vibration device [similar to that used by Sands et al (2006)], 30 Hz, 2-mm displacement, was applied to four sites, four times for 10 s, with 5 s of rest in between as in based on the same protocol [Sands et al (2006)]. Right and left forward split (RFS and LFS) flexibility was measured by the distance between the ground and the anterior superior iliac spine. A force plate (sampling rate, 1000 Hz) recorded countermovement and static jump characteristics. Explosive strength variables included flight time, jump height, peak force, instantaneous forces and rates of force development. The results demonstrated a statistically significant increased flexibility (p) with large effect sizes (d) in both the right forward split (p =1.28, d = 0.67) and left forward split (p = 2.35, d = 0.72) (Figure 6.3, Table 6.1). A very interesting finding in this research is that the addition of vibration to stretching not only increases flexibility but also maintains explosive strength (e.g., jumping ability). This observation is important considering that the athletes were already warmed up, as evidenced by the lack of change in ROM among the groups not receiving any vibration. As a possible mechanism affecting flexibility by vibration and stretching, the author mentioned decreased musculotendinous stiffness, muscular antagonist inhibition and increased pain threshold. It is important to note that, in the present study, stretching alone resulted in a loss of jump performance; however, the addition of acute vibration seems to have preserved the ability to express explosive strength (Tables 6.1 and 6.2).
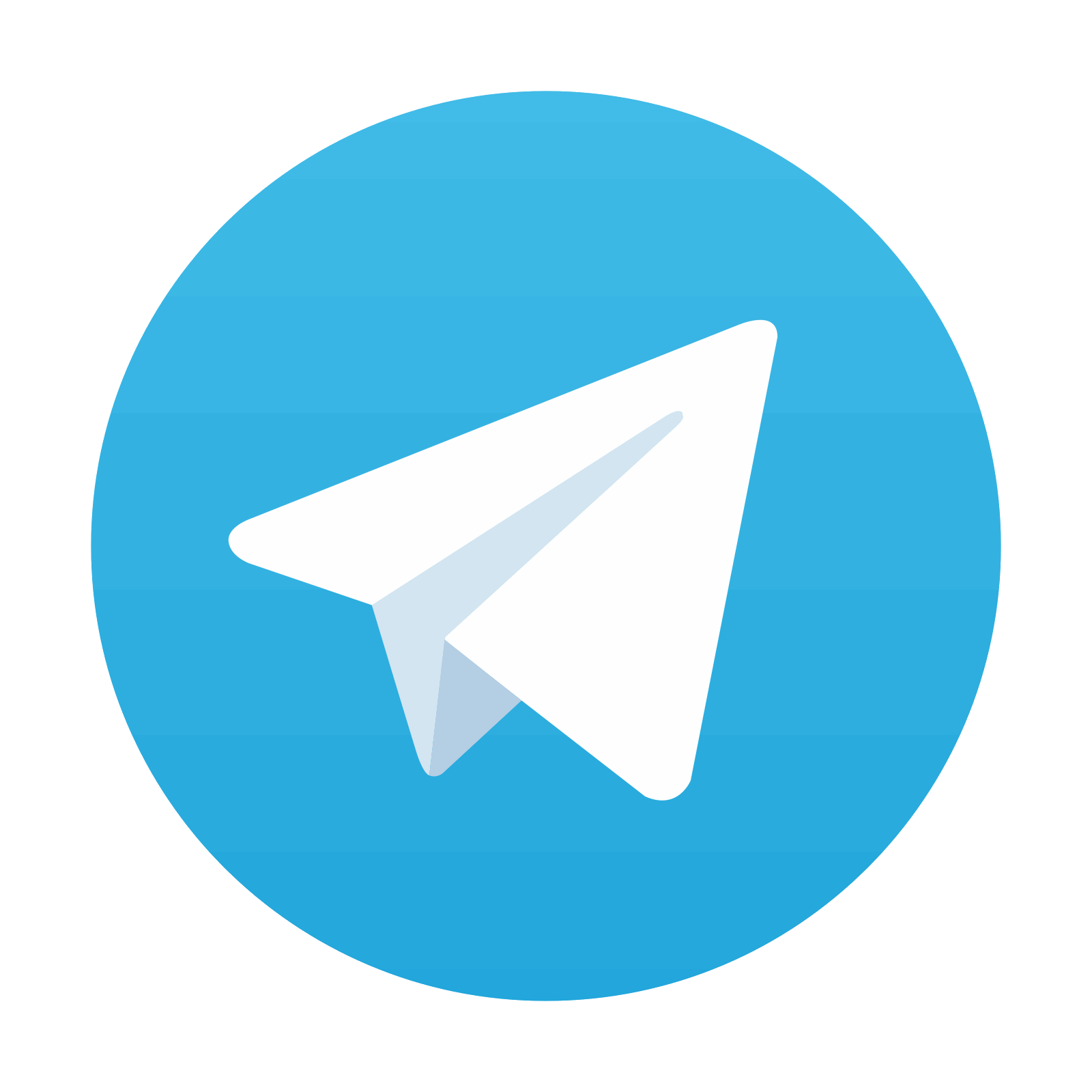
Stay updated, free articles. Join our Telegram channel

Full access? Get Clinical Tree
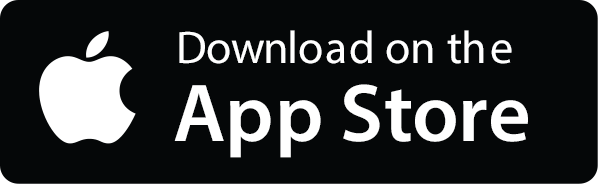
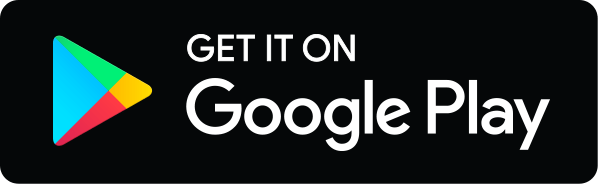