Chapter 14 Vertebral Subluxation and the Anatomic Relationships of the Autonomic Nervous System
Autonomic, preganglionic, postganglionic, splanchnic, somatic, visceral efferent, visceral afferent, sympathetic, parasympathetic, enteric, somatovisceral reflex, viscerosomatic reflex
The subluxation is the greatest single factor in perpetuating any imbalance that may exist between the sympathetic and the parasympathetic nervous systems.
After reading this chapter you should be able to answer the following questions:
Question 1 | How are the three components of the autonomic nervous system differentiated? |
Question 2 | How does the location of preganglionic axons differ between the sympathetic and parasympathetic nervous systems? |
Question 3 | How do the functions of the sympathetic and parasympathetic nervous systems differ? |
Question 4 | On what basis can the treatment of visceral disorders be included in the scope of chiropractic practice? |
The structure of the nervous system is commonly divided into the central nervous system (CNS), which consists of the brain, brainstem, and spinal cord, and the peripheral nervous system (PNS), which includes all neuronal processes outside of the CNS such as cranial nerves and spinal nerves, as well as ganglia associated with these nerves. Beyond pure anatomic description, however, such subdivisions are inconsequential because functional components of the nervous system extend beyond these artificial structural limits. Numerous examples can be identified in which single neurons extend axonal processes either centrally or peripherally into or out of the CNS, crossing the anatomic barrier between the CNS and PNS to form an integrated, functional system. The student of neuroanatomy should strive to understand both structural and functional relationships within the nervous system because this integrated knowledge of neuroscience is not only much more interesting and meaningful but also abundantly more useful in the clinical setting. At the same time, students should realize that we are now only beginning to understand the mechanisms whereby the nervous system monitors and modulates functional activities throughout body systems and, conversely, how stimuli remote from the CNS may be integrated into neuronal functions within the brain and spinal cord.
It now appears that a more comprehensive definition of the ANS should include conscious control of external factors, such as somatic sensations, which influence the regulatory activity of the ANS. The ANS, although predominantly self-regulatory, is not limited to self-regulation. This is of particular clinical significance because therapeutic intervention that alters somatic or visceral function may have effects in body systems apparently remote from the site of applied therapy. A growing body of evidence suggests that there exists a close correlation between somatic (sensory and motor) functions and visceral (sensory and motor) functions. It appears that somatic and visceral functions are coordinated closely through somatovisceral and viscerosomatic reflex mechanisms involving the ANS, PNS, and CNS. Therefore therapeutic interventions such as vertebral manipulation, ingestion of analgesic or antiinflammatory agents, and even surgery, to name a few, can alter somatic sensations (proprioception, for example) in such a way that visceral functions may become altered. However, as stated previously, the underlying neuronal mechanisms require further elucidation through scientific investigation to understand the complexity of factors that interact to regulate organ function.1
Overview of the Autonomic Nervous System
The sympathetic and parasympathetic divisions are similar structurally, whereas the enteric division, modulated by other autonomic centers, is distinct structurally and functionally from other components of the nervous system. Sympathetic and parasympathetic divisions both originate from preganglionic neurons located within the CNS that extend thinly myelinated axonal processes that synapse with dendrites of postganglionic neurons located mostly in peripheral ganglia. All preganglionic neurons are similar functionally because, regardless of their location or which autonomic division they are part of, these neurons are cholinergic (Figure 14-1). In contrast, postganglionic neurons extend unmyelinated axonal processes that innervate specific viscera directly. In addition, unlike preganglionic neurons, which secrete the neurotransmitter acetylcholine, postganglionic neurons vary in the transmitters they synthesize and secrete. Sympathetic postganglionic neurons are largely catecholaminergic (those that innervate sweat glands are cholinergic), and parasympathetic postganglionic neurons are entirely cholinergic. Sympathetic postganglionic neurons are further subdivided functionally into α- and β-catecholaminergic neurons, which exert different influences on target tissues. Generally α-catecholaminergic innervation is excitatory to smooth muscle, and β-catecholaminergic stimulation is inhibitory.
The two-neuron chain (pre-postganglionic) pattern of innervation is unique to the ANS and is fundamentally different from the single-neuron innervation pattern that is characteristic of somatic neuronal systems. An anatomic feature that may assist in distinguishing conceptually between the sympathetic and parasympathetic divisions is the characteristic location of the ganglia, which contain postganglionic neurons. Sympathetic ganglia are localized near the CNS, indicating that sympathetic preganglionic axons are mostly short. Axons of parasympathetic preganglionic neurons are long because parasympathetic ganglia are located some distance away from the CNS near the viscus they innervate. (See Figure 14-1.)
Peripheral Components of the Autonomic Nervous System
Sympathetic Division
The peripheral components of the sympathetic division, as described by Warwick and Williams,2 include gray and white communicating rami, two bilaterally symmetric and ganglionated sympathetic trunks that house postganglionic neurons; clusters of prevertebral ganglia, which also house postganglionic neurons; splanchnic nerves, which innervate prevertebral ganglia; and vascular nerve plexuses, which conduct postganglionic axons to target viscera. In fact, most sympathetic postganglionic axons course along arterial vessels to reach viscera that they innervate. Many sympathetic axons within these vascular nerve plexuses penetrate the arterial or arteriolar wall along which they pass to supply smooth muscle in that wall and serve to regulate blood flow and blood pressure. Also in these vascular nerve plexuses are large numbers of parasympathetic axons that innervate viscera and numerous visceral afferent nerve fibers, which are sensory to the viscera and pass back to the CNS by autonomic nerves. In addition, vast numbers of sympathetic postganglionic axons course together with somatic nerve fibers within spinal nerves and their branches to reach target structures in the body wall and limbs. Still other postganglionic axons innervate viscera such as the heart and lungs as direct branches from the sympathetic trunks. The sympathetic division, which is the largest division of the ANS, therefore innervates all regions of the body by three different routes: (1) by arterial nerve plexuses, (2) as a component of somatic nerves, and (3) by direct nerve branches from the sympathetic trunks.
Spinal Origin and Peripheral Distribution of Sympathetic Preganglionic Axons
The sympathetic division is commonly referred to as the thoracolumbar portion of the ANS because all sympathetic preganglionic neurons are localized in the thoracic and upper lumbar (T1-L3) spinal cord segments. Within these cord segments, preganglionic neurons form a well-defined column of cells that is called the intermediolateral cell column because of its intermediate and lateral position between the posterior and anterior horns of gray matter. Axons of sympathetic preganglionic neurons, which are thinly myelinated, exit the spinal cord along with axons of somatic motoneurons through anterior roots of spinal nerves at the same spinal level as their soma of origin. On exiting the intervertebral foramen, preganglionic axons branch from the spinal nerve as a white communicating ramus (white because these axons are myelinated), which joins the paravertebral sympathetic trunk. Large numbers of preganglionic axons end by synapsing with dendritic branches of postganglionic neurons, the cell bodies of which are located in these ganglia. Many preganglionic axons innervate postganglionic cells in trunk ganglia located at the same vertebral level as the intervertebral foramen through which they emerge. Alternatively, numerous preganglionic axons or their collateral branches course along the length of the sympathetic trunk to innervate postganglionic neurons in trunk ganglia located more cranially or caudally than their spinal level of preganglionic origin. (See Figure 14-1.) Although all sympathetic axons enter the sympathetic trunk through white communicating rami, indicating that white rami are evident only at spinal nerve levels T1 through L3, not all of these axons terminate in sympathetic trunk ganglia as just described. Many preganglionic axons branch from the sympathetic trunk without having synapsed and course as splanchnic nerves that end in prevertebral ganglia, where additional postganglionic neuronal cell bodies are located. (See Figure 14-1.)
In summary, preganglionic axons terminate by synapsing with dendrites of postganglionic neurons located in one of two ganglionated structures, either ganglia of the sympathetic trunk or prevertebral ganglia. (Note: One exception to this general scheme that is described later is the medulla of suprarenal glands.) It is also important to realize that each preganglionic neuron normally innervates up to 20 postganglionic cells either within a single ganglion or distributed among a number of paravertebral or prevertebral ganglia. In one early study it was found that the ratio of preganglionic to postganglionic neurons in the superior cervical ganglion (described further) may be as high as 1 to 196.3 Functionally this innervation pattern allows for divergence of sympathetic activation and coordination of postganglionic response at several spinal levels.4 For this and other reasons that will become apparent, sympathetic activation results in a mass response, such as generalized constriction of cutaneous arteries, as compared with the more localized parasympathetic response.
Relationships of the Sympathetic Trunk
The paired sympathetic trunks and their ganglia extend the length of the vertebral column (paravertebral) and are closely related to the anterolateral aspect of vertebral bodies and intervertebral discs throughout their course. For this reason, conditions such as abnormal biomechanics, subluxations, bony spurs, and other pathologies at intervertebral and costovertebral joints, which are commonly seen in chiropractic offices, as well as more severe conditions such as ankylosing spondylitis and severe osteoporosis, may have a profound influence on sympathetic functions.5 The trunk courses through the cervical region posterior to the carotid sheath, where it is related closely to prevertebral muscles and fascia (Figure 14-2). In the thoracic region the trunk passes along the necks of upper ribs and is related directly to the fibrous capsules of costovertebral joints in the lower thoracic region (Figure 14-3). The sympathetic trunk continues into the abdominal cavity by coursing between the medial arcuate ligament of the diaphragm (anteriorly) and the psoas major muscle (posteriorly). As the trunk descends through the abdomen, it lies adjacent to lumbar vertebral bodies and intervertebral discs anterior to the psoas major muscle and posterior to the inferior vena cava (on the right) or posterolateral to the abdominal aorta (on the left; Figure 14-4). Near its caudal termination the trunk courses posterior to the common iliac vein, descends anterior to the ala of the sacrum just medial to the anterior sacral foramina, where it lies related directly to the origin of piriformis and terminates finally by joining the trunk of the opposite side anterior to the coccyx and coccygeus muscle as the single ganglion impar (Figure 14-5).
The Cervical Sympathetic Trunk and Its Branches
In the neck extensive fusion of sympathetic trunk ganglia takes place during embryonic development,2 resulting in three (superior, middle, and inferior) cervical ganglia that are joined by the cervical continuation of the sympathetic trunk. (See Figure 14-2.) The largest is the superior cervical ganglion, which is believed to have developed from the coalescence of the upper four cervical ganglia.2 This ganglion lies at the level of C1-C3 vertebrae interposed between the carotid sheath anteriorly and the longus capitis posteriorly and, like the lower cervical sympathetic trunk, is enveloped by prevertebral fascia. Preganglionic innervation of the superior cervical ganglion, as in the middle and inferior cervical ganglia, is derived from neurons in the upper three thoracic spinal cord segments.
Postganglionic axons of neurons in the superior cervical ganglion are distributed to target structures along branches of the internal carotid artery or through a number of direct nerve branches from the ganglion. In addition, the first four cervical spinal nerves, like all spinal nerves, receive gray communicating rami composed of unmyelinated axons of postganglionic neurons from the superior ganglion and its caudal connection with the middle ganglion. These postganglionic axons are distributed to blood vessels, erector pili muscles, and sweat glands in the territory of each of these spinal nerves. Extending from the superior limit of the superior cervical ganglion, the internal carotid nerve conducts postganglionic axons to the internal carotid artery, which lies immediately anterior to the ganglion within the carotid sheath. The internal carotid nerve in this way forms the internal carotid plexus, which supplies the artery and its branches to regulate cerebral blood flow, although this is now thought to be only a minor role of the sympathetic division. The internal carotid plexus enters the cranial cavity along the surface of the internal carotid artery as it passes through the carotid canal and provides the clinically important sympathetic innervation to arteries that supply the cerebrum, meninges of the anterior and middle cranial fossae, hypophysis, orbital contents, and the upper parts of the face and scalp. It is this portion of the sympathetic division that may be involved in the cause of migraine headache. As the internal carotid artery passes through the cavernous sinus, the nerve plexus on its surface extends branches that join the oculomotor, trochlear, abducens, and ophthalmic nerves through which sympathetic postganglionic axons are distributed. Thrombosis of the cavernous sinus, as may occur with infections of the orbit, nasal cavity, paranasal sinuses, and tympanic cavity, may therefore impinge on these cranial nerves, leading to characteristic cranial nerve signs and symptoms, including those associated with sympathetic blockage, as observed in a classic Horner’s syndrome. The signs associated with sympathetic nerve blockage at this site include ptosis caused by the loss of sympathetic innervation to the levator palpebrae superius through the oculomotor nerve and miosis caused by unopposed parasympathetic activation of the sphincter pupillae. Sympathetic postganglionic axons reach the eye to supply the dilator pupillae and arterial vessels of the eyeball along two routes. Some axons branch from the oculomotor nerve, pass through the ciliary ganglion without synapsing, and enter the eye with parasympathetic fibers in short ciliary nerves. Other postganglionic fibers continue along the nasociliary branch of the ophthalmic nerve (CNVI) to enter the eyeball as long ciliary branches.
The jugular nerve branches from the superior cervical ganglion to connect with the glossopharyngeal (CNIX) and vagus (CNX) nerves to also innervate the oral cavity and oropharynx. In addition, the meninges in the posterior cranial fossa receive sympathetic innervation through a plexus of postganglionic axons that originate in the superior cervical ganglion and join the internal jugular vein to enter the cranial cavity by way of the jugular foramen.
The cervical sympathetic trunk also contributes to the cardiac plexus through cardiac branches that arise bilaterally from all three cervical ganglia. The cardiac branch of the superior cervical ganglion courses inferiorly along the anterior aspect of the longus cervicis muscle (see Figure 14-2) partly enveloped by prevertebral fascia, where it may be influenced by damage or increased tonicity of this muscle. On the right the cardiac branch passes most commonly posterior to the subclavian artery, where it is related directly to the cupula of parietal pleura and may be affected by pathology in the apical region of the lung. The nerve continues into the thorax on the posterolateral aspect of the brachiocephalic trunk to enter the cardiac plexus posterior to the arch of the aorta, although some axons may contribute to the anterior cardiac plexus. In contrast, the left cardiac branch of the superior cervical ganglion enters the thorax most commonly along the anterior aspect of the common carotid artery to reach the anterior portion of the cardiac plexus anterolateral to the arch of the aorta. Like the right cardiac branch, the left nerve may contribute to the posterior cardiac plexus as well. En route, both the right and left cardiac branches of the superior cervical ganglia commonly receive communications from the external laryngeal, recurrent laryngeal, and cardiac branches of the vagus (CNX) nerve, indicating that, on reaching the cardiac plexus, the cardiac nerves are mixed, having both sympathetic and parasympathetic components. In addition, cardiac nerve branches of the middle and inferior cervical ganglia commonly have communicating branches with that of the superior cervical ganglion.
Most sympathetic postganglionic neurons are catecholaminergic including those that inner-vate the heart and coronary vessels, and their activation causes the release of norepinephrine (β-catecholaminergic). Interestingly, this neurotransmitter performs a dual role to control and regulate cardiac function. Cardiac myofibers are stimulated by norepinephrine to contract more forcefully and more rapidly. Concomitantly, smooth muscle in the walls of coronary arteries is inhibited, causing dilation of these vessels and increased blood flow to the heart, although Berne and Levy6 suggest that the coronary circulation responds primarily to the metabolic needs of the myocardium and is predominantly under nonneuronal control. In fact, the sympathetic division may serve its greatest regulatory role of cardiac function indirectly by affecting the release of norepinephrine and epinephrine into the bloodstream from medullary cells of the suprarenal glands.
In addition to sympathetic postganglionic axons, visceral sensory (afferent) axons are also present in all cardiac branches of the sympathetic trunk, except those arising from the superior cervical ganglia. Cardiac pain is transmitted through these sympathetic cardiac nerves to upper thoracic spinal cord segments and for this reason may be referred to the medial aspect of the arm and adjacent thoracic wall. These neuronal pathways also may provide a viscerosomatic reflex mechanism whereby cardiac pain provokes increased tonus and even spasm of muscles innervated by upper thoracic spinal cord segments, as observed in angina pectoris and cardiac arrest.
From the superior cervical ganglion the sympathetic trunk courses inferiorly along the anterior aspect of the longus capitis and longus cervicis muscles to connect with the middle cervical ganglion at the level of the sixth cervical vertebra. (See Figure 14-2.) Occasionally this ganglion is poorly defined or absent, in which case the postganglionic neurons normally present in the middle ganglion are dispersed along the length of the cervical sympathetic trunk. The middle cervical ganglion is formed most commonly by the coalescence of the fifth and sixth cervical ganglia and is related to the inferior thyroid artery, which is innervated by a sympathetic plexus derived from this ganglion. Sympathetic postganglionic axons are distributed to branches of this artery that supply deep posterior neck muscles, prevertebral muscles, and the external vertebral arterial plexus as well as the thyroid and parathyroid glands. Postganglionic axons derived from neurons predominantly in the middle and inferior cervical ganglia form a nerve plexus on the external carotid artery. This external carotid nerve plexus supplies the artery and follows its branches to innervate structures supplied by the artery in the neck and face.
A number of nerve cords that form the inferior continuation of the sympathetic trunk join the middle cervical ganglion to the inferior cervical (cervicothoracic) ganglion. Some nerve fibers of the cervical sympathetic trunk pass posterior and anterior to the vertebral artery to reach the inferior ganglion and may contribute to the nerve plexus around this vessel. A large nerve cord called the ansa subclavia passes inferiorly and anteriorly to the origin of the subclavian artery, loops around this vessel just medial to the internal thoracic artery, and joins the inferior cervical ganglion posterior to the subclavian artery, The ansa subclavia commonly contributes to the nerve plexuses surrounding both the subclavian and internal thoracic arteries. (See Figure 14-2.) The inferior cervical ganglion is considerably larger than the middle ganglion and is formed by the coalescence of the C7, C8, and T1 ganglia. Other names given to the inferior ganglion are the cervicothoracic ganglion, because of its embryonic origin, and the stellate ganglion, because of its shape. The inferior cervical ganglion is positioned anterior to the C7 transverse process. It extends inferiorly to the neck of the first rib just posterolateral to the origin of the vertebral artery. (See Figure 14-2.) The ganglion is also related directly to the cupula of parietal pleura and the lower vertebral attachment of the scalenus medius muscle. In some individuals the lateral border of the longus cervicis muscle is also related to the medial aspect of the inferior ganglion.
Postganglionic axons from neurons in the inferior cervical ganglion join the C7, C8, and T1 spinal nerves through gray communicating rami; they are distributed by these nerves to the periphery including cutaneous and muscular arteries of the forearm and hand. Other nerve branches of the inferior cervical ganglion are a cardiac branch, which joins the posterior cardiac plexus along with the cardiac branch from the middle cervical ganglion, and vascular branches, which form plexuses on the subclavian and vertebral arteries as well as on the thyrocervical and costocervical trunks. The subclavian plexus extends into the axilla along the first part of the axillary artery and its superior thoracic branch, but it rarely reaches arteries in the upper limb because these appendicular vessels receive direct sympathetic innervation from nerves of the brachial plexus. The large vertebral branch of the inferior cervical ganglion courses along the vertebral artery through foramina transversarii to form a vertebral nerve plexus, which supplies arteries to the cervical spinal cord, external and internal vertebral arterial plexuses, and deep muscles of the neck. The vertebral nerve plexus enters the cranial cavity with the vertebral arteries through the foramen magnum and courses along the length of the basilar artery and its branches as far as the posterior cerebral arteries. In this way postganglionic axons derived from the inferior cervical ganglion primarily supply sympathetic innervation that functions to regulate blood flow to occipital and temporal lobes of the cerebrum and cerebellum as well as vital neuronal centers in the brainstem and cervical spinal cord. It has been suggested recently, however, that neural control of cerebral blood flow may not be as important as once thought. Rather, it may be that the flow rate of blood in cerebral arterioles is regulated primarily by regional metabolic needs.6 Near the posterior portion of the circulus arteriosus, the vertebral sympathetic plexus meets that of the internal carotid plexus. If sympathetic function in the inferior cervical ganglion is compromised, as may happen in a variety of clinical conditions (for example, cervical rib; abnormal biomechanics of the lower cervical and upper thoracic spine), a number of signs and symptoms associated with the syndrome referred to as vertebrobasilar insufficiency are observed. Upper limb symptoms may be present, resulting in a classic thoracic outlet syndrome. Hyperactive sympathetics may give rise to tinnitus, hearing loss, dizziness, facial nerve (CNVII) palsy, blurred vision, nausea, and vomiting, as well as cardiac and respiratory arrhythmia,7 which result from insufficient blood flow to the brainstem and cerebellum. Migraine-type headache also has been reported to occur after whiplash injury to the cervical spine8 and may be the result of trauma or pressure on the cervical sympathetic trunks.
The Thoracic Sympathetic Trunk and Its Branches
The thoracic sympathetic trunk consists of a series of small ganglia that vary somewhat in number. Generally there is one ganglion for each thoracic spinal nerve, although the first thoracic ganglion is most commonly fused with the inferior cervical ganglion. As the sympathetic trunk descends through the thorax, the ganglia come to lie in direct contact with the fibrous capsules of costovertebral joints, except in the lower thorax, where they lie more medially adjacent to T10-11 and T11-12 intervertebral discs. (See Figure 14-3.)
Each thoracic spinal nerve receives both white and gray communicating rami from the sympathetic trunk and its ganglia, with the white ramus joining the spinal nerve slightly more distal than the gray ramus. (See Figure 14-3.) Occasionally, the white communicating rami are fused and only one mixed white and gray ramus is present. Each spinal nerve conducts these postganglionic axons to sweat glands and erector pili muscles, as well as cutaneous and muscular arteries within the thoracic and abdominal walls. Of particular clinical importance is the sympathetic nerve plexus formed by postganglionic branches of the thoracic sympathetic trunk on the aorta and its intercostal, esophageal, and bronchial branches. Segmental arteries that originate from intercostal arteries carry sympathetic innervation to the external and internal vertebral arterial plexuses and its radicular branches. By this route the autonomic nervous system can function to regulate blood flow to bones, joints, and ligaments of the thoracic vertebral column and structures within the spinal canal, including the spinal cord, spinal nerve roots, and meninges.
The pulmonary plexus receives direct input of sympathetic postganglionic axons from branches of upper thoracic (T2-T5) sympathetic trunk ganglia as well as indirect input from the anterior and posterior cardiac plexuses. As mentioned previously, the posterior cardiac plexus is formed in part by sympathetic postganglionic axons that enter the plexus directly from upper thoracic (T2-T5) trunk ganglia. From the pulmonary and cardiac plexuses, a small network of sympathetic postganglionic axons branch away to supply the mucosa of the trachea and esophagus. Within the root of the lung bilaterally, sympathetic axons from the pulmonary plexus form a delicate nerve plexus along the surfaces of pulmonary and bronchial arteries as well as on the bronchial tree to supply smooth muscle in the walls of these structures. Activation of the sympathetic division causes release of norepinephrine, which is inhibitory to bronchial smooth muscle and results in bronchodilation. It is excitatory to arterial smooth muscle, causing constriction of pulmonary and bronchial arteries. However, neuronal control of bronchial smooth muscle has been shown to be relatively insignificant when compared with the bronchiolar response to local tissue factors.8 For example, circulating levels of norepinephrine and epinephrine secreted into the bloodstream by suprarenal glands during sympathetic activation act as potent β-catecholaminergic receptor stimulants that elicit rapid dilation of the bronchial tree.9,10 In contrast, other local factors such as histamine and the slow reactive substance of anaphylaxis that are released from mast cells into lung tissues after exposure to allergens act as bronchoconstrictors.11 It appears that local physiologic needs of the tissues serve as the primary regulatory control of pulmonary function, whereas direct ANS influences are limited.
Similarly, the ANS is believed to have little control over the pulmonary circulation during normal daily activity. Pulmonary vascular resistance, which is a measure of the freedom with which arterial blood flows through the pulmonary circulation, is known to be inversely proportional to cardiac output.12,13 That is, pulmonary and bronchiolar arteries expand or collapse passively in response to an increase or decrease, respectively, in blood pressure.14 However, a clinically significant role of the sympathetic division in the regulation of pulmonary circulation has been suggested by Fishman,15 who indicated that pulmonary obstruction may reflexly stimulate sympathetic vasomotor activity to cause generalized constriction of pulmonary vessels and increased arterial pressure in the lung. It is also thought that constriction of larger pulmonary veins in response to sympathetic stimulation may be the primary mechanism whereby blood is shunted from the pulmonary to the systemic circulation when needed. In summary, the principal mechanism whereby the sympathetic division regulates pulmonary function is humeral because, as in the control of cardiac function, it has its greatest influence through activation of the suprarenal glands.
As mentioned previously, there are three pairs of bilaterally symmetric splanchnic (visceral) nerves, called the greater, lesser, and least (lowest) splanchnic nerves, that arise from the thoracic sympathetic trunks. The splanchnic nerves are composed of preganglionic axons of neurons located in the intermediolateral cell column of the thoracolumbar spinal cord and do not synapse within sympathetic trunk ganglia. Instead, these axons pass through the sympathetic trunk, branch medially away as splanchnic nerves, and terminate finally by synapsing with dendrites of postganglionic neurons in prevertebral sympathetic ganglia. (See Figures 14-1 and 14-3.) The prevertebral ganglia are located anterior to the lumbar spine and sacrum in association with major branches of the abdominal aorta. The largest splanchnic nerve branch of the thoracic sympathetic trunk is the greater splanchnic nerve, which is formed by five roots arising from the fifth through ninth (sometimes tenth) thoracic trunk ganglia. (See Figure 14-3.) Most preganglionic axons within the roots of the greater splanchnic nerve originate in the thoracic spinal cord segments adjacent to these ganglia. However, a small proportion of preganglionic neurons in upper thoracic spinal segments also may contribute to this nerve. The lesser splanchnic nerve is somewhat smaller, having only two roots of origin normally from the ninth and tenth (sometimes tenth and eleventh) thoracic trunk ganglia. The least (lowest) splanchnic nerve is the smallest, arising singly from the last thoracic trunk ganglion. All three splanchnic nerves course anteromedially and inferiorly along vertebral bodies and intervertebral discs to reach the diaphragm. The greater and lesser splanchnic nerves normally gain access to the abdominal cavity by piercing the crus of the diaphragm, and the least splanchnic nerve enters the abdomen with the sympathetic trunk by passing between the medial arcuate ligament of the diaphragm and the psoas major muscle. Often an enlargement of the greater splanchnic nerve called the splanchnic ganglion is present just before the nerve pierces the diaphragm.
The suprarenal glands are discussed in some detail because of the important functional role these glands play in the sympathetic division of the ANS. These glands are supplied by the greater splanchnic nerve, an innervation pattern that may appear unusual in that preganglionic axons innervate medullary cells of the gland directly. However, when one considers the embryonic origin of the gland, this innervation pattern can be better understood. Histologically the gland is composed of a layered cortex that develops from mesodermal cells near the developing dorsal mesentery of the embryo and a central medulla derived from neuroepithelial cells of the neural crest.16 The medullary cells of the gland in this way develop from the same primordium as all sympathetic postganglionic neurons and may be considered to be homologous to postganglionic neurons. Like most sympathetic postganglionic neurons, medullary cells synthesize and secrete the hormones (neurotransmitters) epinephrine and norepinephrine, which when released into the bloodstream evoke a generalized sympathetic (fight-or-flight) response.
The suprarenal nerve plexus is composed of axons of the greater splanchnic nerve, which reach the plexus on the anterior aspect of the crus of the diaphragm by passing through the celiac ganglion and plexus without synapsing. The suprarenal glands, located just lateral to the crus of the diaphragm adjacent to the superomedial pole of each kidney, are said to have the largest sympathetic innervation relative to size when compared with other organs. This is understandable considering the important role this gland serves during sympathetic activation. Preganglionic axons enter the gland and terminate on two types of medullary cells called chromaffin and ganglion cells. (Note: Cortical layers of the gland that function to synthesize and secrete glucocorticoid, mineralocorticoid, and corticosteroid hormones are not innervated.) The chromaffin cells, innervated by synapse-like junctions, synthesize the catecholamines epinephrine and norepinephrine. These products then are stored in separate cytoplasmic granules recognized at the electron microscopic level to be distinct for one specific hormone. On stimulation of chromaffin cells by preganglionic axons, the granules bind with the plasmalemma and release their contents into adjacent blood vessels. The second medullary cell type innervated by preganglionic axons is a multipolar neuron-like cell referred to as a ganglion cell. However, the axons of ganglion cells and their terminations have not been studied definitively. It is possible that ganglion cells may serve to magnify incoming excitatory sympathetic stimuli and disseminate the signals to chromaffin cells. After release into the bloodstream, these hormones circulate throughout the body and act to elicit the characteristic sympathetic response to stressful conditions that the individual may be facing.
The distribution of postganglionic axons from neurons in prevertebral ganglia follows a characteristic pattern; the axons course through delicate nerve plexuses formed on the external surfaces of large branches of the abdominal aorta near the ganglion in which they originate. Each arterial nerve plexus supplies smooth muscle in the wall of the vessels along which it passes and terminates by innervating viscera supplied by the vessel. Axons of neurons in the celiac ganglion are distributed along branches of the celiac trunk, which come off the aorta at the level of the twelfth thoracic vertebra near the aortic hiatus of the diaphragm. The right and left celiac ganglia are interconnected by a massive nerve plexus called the celiac plexus that extends inferiorly to the level of the first lumbar vertebra and surrounds both the celiac trunk and origin of the superior mesenteric artery. (See Figure 14-4.) Entering the plexus from each side are the greater and lesser splanchnic nerves and parasympathetic axons from the vagus (CNX) nerve (discussed further). Exiting the celiac plexus are sympathetic preganglionic axons, which supply the suprarenal glands, parasympathetic (vagal) axons, and sympathetic postganglionic axons that enter subsidiary plexuses to be distributed to abdominal viscera. These secondary nerve plexuses include the phrenic plexus, the left gastric, splenic, and hepatic plexuses, which course along branches of the celiac trunk, and the renal, gonadal, superior mesenteric, and inferior mesenteric plexuses, which follow lower branches of the abdominal aorta.
Sympathetic postganglionic axons in the hepatic plexus reach the liver along branches of the hepatic artery and portal vein. The innervation that reaches the liver by this route is believed to function only to regulate blood flow to that organ because the characteristic glycogenolytic response of the liver to sympathetic activation is a result of increased circulating levels of epinephrine and norepinephrine derived from suprarenal glands. A small cystic plexus originating from the hepatic plexus contains sympathetic postganglionic axons that are inhibitory to smooth muscle in the wall of the gallbladder but excitatory to the sphincter of the common bile duct. The hepatic plexus also contributes to the nerve plexus surrounding the gastroduodenal branch of the hepatic artery to innervate the right side of the stomach, duodenum, head of the pancreas, and the most distal portion of the common bile duct. (Note: Sympathetic innervation of the gastrointestinal tract functions to inhibit neuronal activity in the enteric division of the ANS, described subsequently.)
Also extending from the celiac plexus are the left gastric and splenic plexuses, which follow the arteries of the same name and terminate by innervating the left side of the stomach, tail of the pancreas, and the spleen. The sympathetic innervation of the spleen is excitatory to smooth muscle in the capsule of this organ and causes expulsion of the relatively large reservoir of blood into the general circulation at times of need, such as during exercise or serious blood loss caused by injury. More importantly, sympathetic influences on the spleen and other lymphoid organs function to regulate the immune system.17 The immunoregulatory role of the sympathetic nervous system is elaborated in the final section of this chapter.
The renal plexus is derived from the more inferior portion of the celiac plexus as well as the aorticorenal and renal ganglia, thus receiving input from the lesser and least (lowest) splanchnic nerves. Ganglia within the renal plexus give rise to postganglionic axons, which follow and supply branches of the renal artery and innervate glomeruli and convoluted tubules in the renal cortex. Both afferent and efferent arterioles of the glomeruli are innervated; however, the influence of sympathetic innervation on the glomerular filtration rate is only minor because neuronal control is superseded by renal autoregulatory mechanisms.18,19 From the renal plexus, the upper part of the ureter and gonadal (testicular/ovarian) arteries also receive sympathetic innervation, which is excitatory to smooth muscle in their walls.
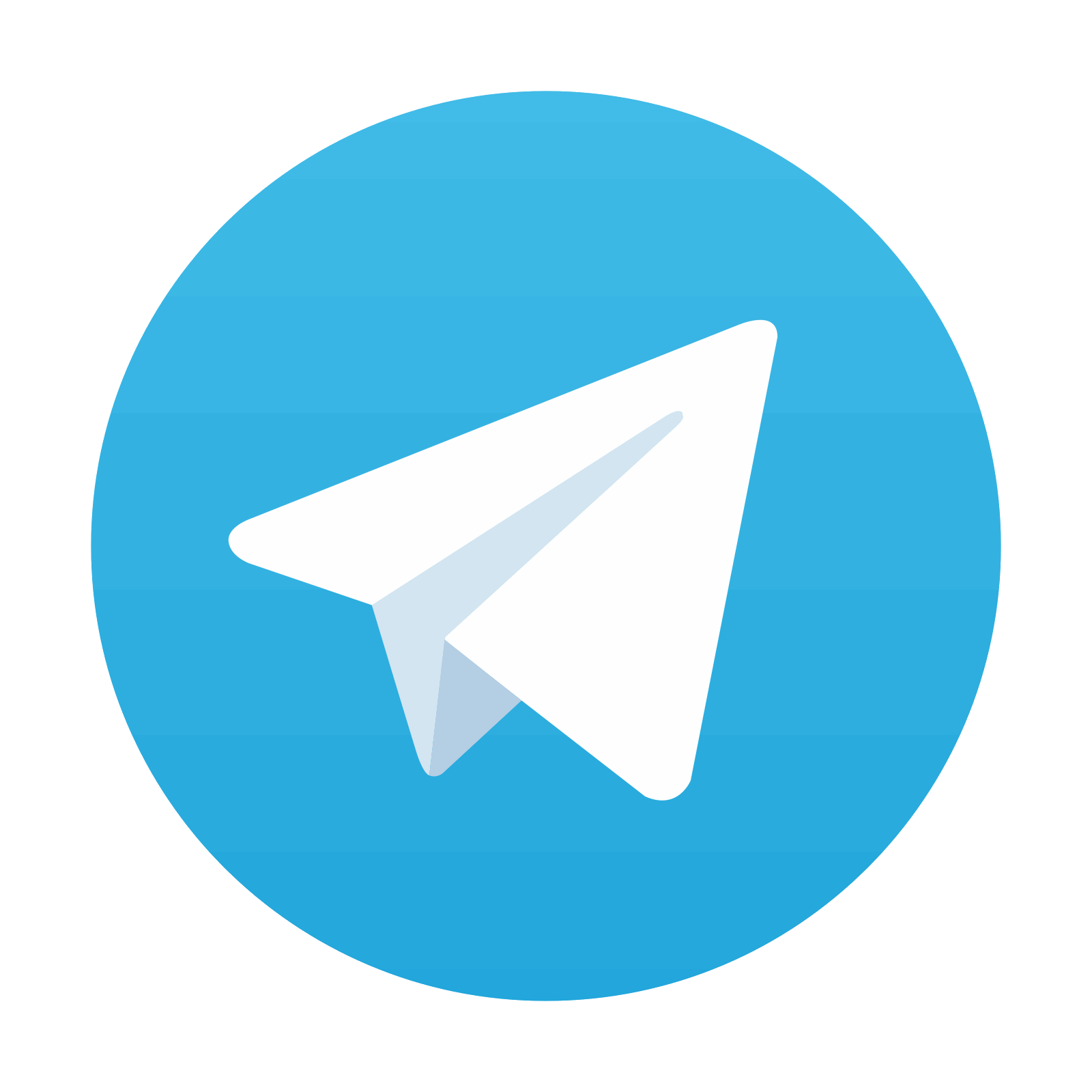
Stay updated, free articles. Join our Telegram channel

Full access? Get Clinical Tree
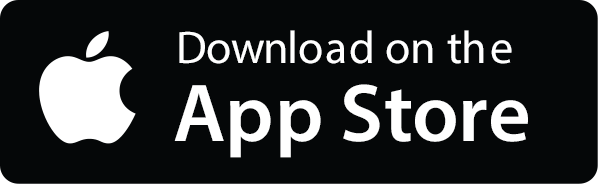
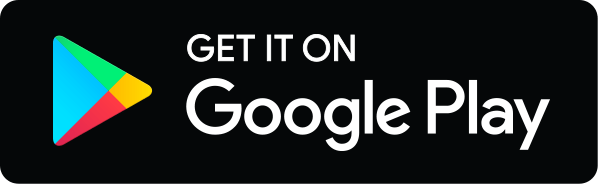