Chapter 4 Animal Models in the Study of Subluxation and Manipulation: 1964-2004
After reading this chapter you should be able to answer the following questions:
Question 1 | To what extent has chiropractic’s subluxation theory been explored by basic science research? |
Question 2 | What issues arise from animal model research? |
Question 3 | What are eight reasons for using animal models in research? |
Question 4 | What are Haldeman’s basic criteria for judging any proposed neurobiological mechanism for spinal manipulative therapy (SMT)? |
• Are different types of subluxation more serious in their biomechanical and systemic effects?
• Is there a “time window” when spinal manipulation is most effective in preventing or correcting the adverse consequences of subluxation?
• What are the effects of different therapeutic techniques for a given patient?
• What determines the optimal number or frequency of spinal manipulation treatments for a given patient?
Although studies conducted with animal models must be followed by human studies, the role of animal research is critical to human studies. Animal research provides essential data about biological mechanisms that are needed to identify and clarify questions for human research. In fact, the absence of relevant animal studies make clinical studies very difficult to justify to Institutional Review Boards that serve as the gatekeepers for human research.4 Moreover, the absence of basic research evidence prompts the larger scientific and health care communities to treat lightly the results of such clinical studies. This is particularly true for studies examining chiropractic treatment of visceral complaints. In his review of animal models used in subluxation research, Vernon5 noted the great need for basic science research if the chiropractic profession wants to persuade the larger health care community that chiropractic has a place in the treatment of nonmusculoskeletal conditions. He stated the following:
Vernon presented eight reasons for using animal models in research:
1. Test theories derived from conceptual models
2. Provide data to support clinical experience
3. High level of experimental control
4. Prospective; therefore can explore cause and effect relationships
5. Explore “treatment” effects when lesion is reversed
6. Explore physiologic components of subluxation
7. Chronic experiments may allow for exploration of behavioral effects
According to Vernon, there are essentially two ways to model the spinal subluxation. First, investigators may create the obligate precipitating elements of the subluxation, vertebral fixation, and misalignment and monitor the effects:
In this chapter we present an overview of animal models that have been used to study subluxation, or in the case of osteopathy, the osteopathic lesion. Of course, not all basic research that investigates subluxation and manipulation must involve animals. A number of valuable basic science studies have been performed in humans.6–8 However, as illustrated by Vernon’s eight reasons for using animal models in research, animal research provides research opportunities that cannot be accomplished in human studies.
“Ownership” of the Subluxation
It is often a jolting surprise to those arguing chiropractic ownership due to “original discovery and development” that neither the term subluxation nor its chiropractic usage began with the Palmers. The first published use of the term in chiropractic was by Smith, Langworthy, and Paxson in their 1906 two-volume textbook, Modernized Chiropractic.9 The earliest attribution of subluxation by the Oxford English Dictionary is to Holme in 1688; “Sublaxation [sic]—a dislocation, or putting out of joynt [sic].”10 A more “chiropractic” definition of the term is found in the work of Joannes Heironymi in his 1746 thesis De Luxationibus et Subluxationibus:
…subluxation of joints is recognized by lessened motion of the joints, by slight change in position of the articulating bones and pain…most displacements of vertebrae are subluxations rather than luxations.11
Finally, Edward Harrison was an outspoken advocate for the manual correction of subluxations.12–14 His words could have come right out of an early chiropractic text. Yet his description of the subluxation and its treatment was written at least 70 years before D.D. Palmer’s historic discovery:
Definition of Subluxation
In the first edition of this text, Gatterman15 stated the following:
Gatterman noted that it is important that the definition of a subluxation be broad enough to include the medical concept of subluxation, a lesion that is severe enough to be visible on radiographic examination. (See Chapter 8.) This led to further refinement of the term. It was acknowledged that some subluxations may be unstable, require surgical repair, or be aggravated by manipulation. For this reason, the term manipulable subluxation has been defined. A manipulable subluxation is “a subluxation in which altered alignment, movement, or function can be improved by manual thrust procedures.” (See Chapter 1.) Models that mimic subluxation in this chapter attempt to emulate the manipulable subluxation. They incorporate altered motion (fixation) with and without misalignment (malposition) while retaining contact between the joint surfaces. Gatterman noted that there are more than 100 synonyms for the subluxation. Why so many? Perhaps the reason can be found in two observations:
1. Most definitions of the chiropractic subluxation, spinal manipulation, or adjustment serve political or legal purposes. The originators of these definitions generally represent broad, sometimes conflicting, constituencies so their definitions are invariably broad and imprecise. By contrast, operational definitions used in scientific studies must be clearly defined, rather restrictive statements. These operational definitions permit scientists to write and subsequently test very focused research hypotheses.
2. The chiropractic subluxation remains an unvalidated theoretical construct because there is no widely accepted physical model of the subluxation. It remains an abstract concept, free to drift with the shifting winds of political/legal necessity and philosophical/entrepreneurial influence.
Definition of Manipulation
As noted above, there has been a longstanding polemic in the chiropractic profession about the use of the term manipulation preferred by researchers and the term adjustment used historically by chiropractic field practitioners. In the research literature, manipulation is broadly used to refer to any manual or mechanical movement of a joint by an applied load. This may include the application of an impulse thrust, most characteristic of the “chiropractic adjustment” or the more slowly applied “mobilization” forces commonly used by physical therapists and osteopaths. In a widely referenced taxonomy for manipulation developed by the Technique Council of the American Chiropractic Association, a six-tiered system is used to categorize the various chiropractic treatment procedures.16 (See Chapter 7.) This system distinguishes manually applied forces from mechanically applied forces, specific and nonspecific contacts, long and short lever applications, rate of application, and amplitude of joint motion. Authors using this taxonomy generally describe the doctor and the patient/subject setup, areas of contact (e.g., support and therapeutic load contacts), rate of load application, and the expected amplitude of joint motion. Therefore manual manipulations that are most common to chiropractic practice, impulse thrusts, are frequently described as high velocity, low amplitude (HVLA), while mobilization manipulations are often described as low velocity, high amplitude (LVHA). In clinical studies, descriptions of chiropractic manipulation are almost always based upon what the treating doctor “intended” to do. There are seldom kinetic (force) and kinematic (displacements, velocities, and accelerations) measurements of the applied loads and their outcomes. Therefore the actual application of the manipulation taxonomy relates more to the manipulator’s intended mechanical outcome rather than the true nature of the procedure. By contrast, most basic science studies (examining mechanisms) report actual kinetic and kinematic data. In this chapter our report of the specific treatment(s) applied in a given study was limited by the quality of description given by the authors.
Characteristics of Animal Models
Models provide an essential component in our attempt to understand both normal and pathologic processes in human beings.17,18 They are simplified representations of a system of interest that replicate or approximate critical features of that system. It follows that the validity of any given model lies in its ability to provide clarification or new understanding about the system of interest. Mechanical or computer models can provide useful information about simple physical or chemical interrelationships, but they are quickly overtaxed by complex interactions required to represent even the most primitive mammalian system. By contrast, direct examination of living tissues readily permit researchers to study complex biological interactions and even evaluate the effect of experimental intervention. Valuable work has been done with isolated tissue preparations, such as the study of cellular and synaptic pathologies in brain slices or the study of mechanical state encoding in isolated joint capsules.19,20 However, only intact animal models allow investigators to examine complex global interactions between major body systems.21,22 A good animal model for basic health care research should have at least five features. It should: (1) be appropriate for evaluating specific hypotheses relating to normal or pathologic mechanisms, (2) permit rapid and reproducible examination of numerous study subjects, (3) be economical, (4) not present serious ethical concerns, and (5) allow valid extrapolation to the human condition. One of the most powerful applications of a physical model is the emulation of a conceptual construct (conceptual model). In this chapter we introduce animal models that attempt to emulate the central foundational constructs of chiropractic—subluxation and spinal manipulation.
Issues in Animal Model Research
One of the five characteristics listed above for a good animal model was that it should “not present serious ethical concerns.” For some this is an impenetrable boundary. They conclude that there are no “good” animal models because all animal research presents intractable ethical problems from their perspective.23,24 However, researchers and the public generally recognize a continuum of animal research.25–27 The ends of this continuum are defined by reasoned consideration of potential health benefits as well as threats to animal welfare. Unacceptable animal research has low potential benefit and/or involves a disproportionate loss of animal life, pain, or distress. By contrast, good animal research will have both a demonstrable benefit to human and/or animal health and a proportionately low loss of animal life, pain, and distress. For this reason, animal research protocols must contain thorough explanations of why animals are required for the proposed work and include what steps were taken to find nonanimal alternatives. If it is established that there is no acceptable alternative to using animals, the animal species and the minimal number of animals required must then be explicitly justified. Potentially painful or stressful procedures must be explained and detailed strategies must be provided for limiting pain and distress (e.g., anesthetics, analgesics, and animal care). Critical review of proposed animal research projects and reasoned consideration of the potential benefits of each project, as well as its impact on animal welfare, are the responsibility of each institution’s Institutional Animal Care and Use Committee (IACUC). The IACUC also establishes and monitors species-appropriate housing and care for all animals at the institution. The constituency of this important committee and its procedures are prescribed by federal statute and closely monitored by two federal agencies, the Office of Laboratory Animal Welfare (OLAW) at the National Institutes of Health (NIH), and the Animal and Plant Health inspection Service (APHIS) at the United States Department of Agriculture (USDA).28 In addition, many state, county, and city statutes further govern animal research. The benefit of this work to human and animal health has been both essential and profound, demonstrated by the observation that 7 of the last 10 Nobel Prizes have relied, at least in part, on animal research.25,26,29
Frequently the question is asked: How can we learn anything meaningful about humans from animal studies when the differences between the species are so great? Most laboratory animals have organs and body systems that are quite similar to humans. From these similarities we have learned a great deal about human physiology. Also, many laboratory animals are susceptible to the same diseases that affect humans. Because of this, scientists have been able to apply information gained from animal studies not only to humans, but to pets, wildlife, and other animals. This information has led to a dramatic increase in life expectancy in the United States, from an average of 49 years in 1900 to 76 years at the turn of the twenty-first century. Americans are not only living longer—they are living healthier. Between 1994 and 1996, 72% of Americans 65 years of age and older reported their health was good or excellent.30,31 In addition, disability among older Americans is declining dramatically and at an accelerating pace. According to analyses from the National Long Term Care Survey, the percentage of people age 65 and older with disabilities fell 1.6% per year from 1989 through 1994 and 2.6% annually from 1994 though 1999.32 The improvements in recent years are also noteworthy for a newly observed decline in disability among black Americans as well as a decrease of at least 200,000 in the number of people estimated to live in nursing homes. These benefits are due in great part to improved health care that was advanced by animal research.
It is important to appreciate that many of the greatest scientific discoveries were achieved because researchers exploited important differences between humans and laboratory animals. Two animals used in neuroscience research nicely illustrate this point. The anatomy and physiology of the giant squid is dramatically different from that of a human (Figure 4-1). Giant squid axons can be up to 1 mm in diameter, 100 to 1000 times larger than mammalian axons! This tremendous size allowed researchers to obtain the first intracellular recordings of action potentials from nerve cells as well as the first experimental measurements of ionic currents, thereby allowing us to understand how the nervous system conveys information.33,34 The great size of the axon also permitted researchers to extrude the cytoplasm by pressing it out with a small roller, much like toothpaste in a tube, and measure its ionic composition. They were subsequently able to replace the axoplasm with artificial solutions and observe resulting changes in membrane potential. Moreover, some giant squid nerve cells form very large synaptic contacts with other giant nerve cells, allowing extraordinary access to these important neurological structures.35,36 Hodgkin and Huxley were awarded the Nobel Prize in 1963 for their body of work, much of it done with the giant squid axon.
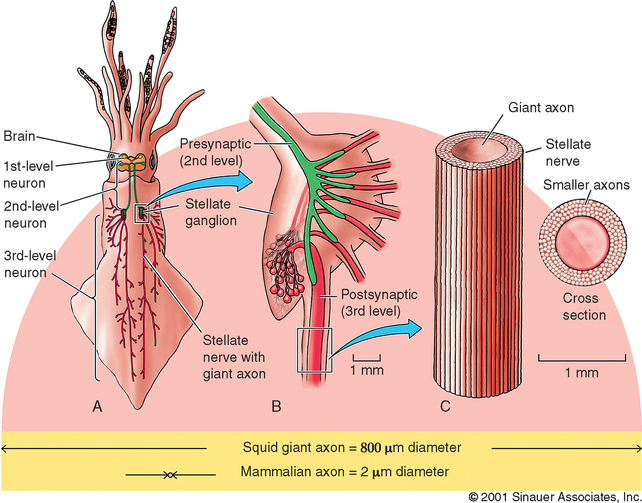
Figure 4-1 Giant squid axons can be up to 1 mm in diameter, 100 to 1000 times larger than mammalian axons! This tremendous size allowed researchers to attain the first intracellular recordings of action potentials from nerve cells as well as enabled the first experimental measurements of ionic currents, thereby allowing us to understand how the nervous system conveys information.33,34
(Reprinted with permission from Purves D, Augustine GJ, Fitzpatrick D, Katz LC, Lamantia A, McNamera JO et al. Neuroscience. 2nd ed. Sunderland, MA: Sinauer Associates, Inc.; 2001. p. 53.)
One final example suggests a possible National Enquirer headline decrying government waste: “US Government Spends Millions of Your Tax Dollars to Study Frog Eggs!” In reality, these studies combine molecular and physiological methods to make an exciting new research tool that takes advantage of unique features in the clawed African frog, Xenopus laevis.37 The eggs of the female frog are huge (approximately 1 mm in diameter), are easily harvested, and have few endogenous membrane-bound ion channels (Figure 4-2). These attributes permit scientists to inject exogenous mRNA into the oocytes, causing them to synthesize proteins that then produce large quantities of membrane-bound ion channels.38 The size of the eggs also permits the use of classical methods, such as the patch clamp technique, to study ionic currents generated by the newly formed channels.39,40 With this powerful tool, scientists can now directly study the relationship between channel structure and function. Defined mutations common to neurological diseases (often affecting a single nucleotide) can also be produced to assess the functional consequences of a mutation. These and other extraordinarily valuable animal studies have formed the foundation of modern neuroscience and, subsequently, clinical practice.
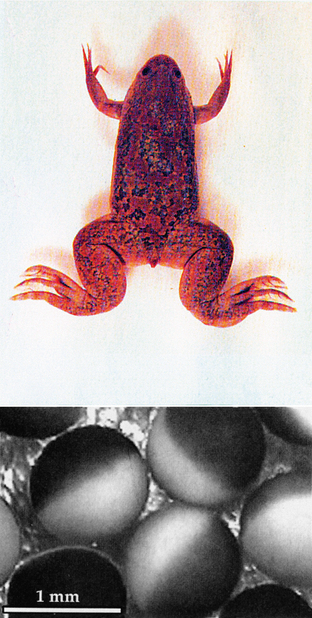
Figure 4-2 The eggs of the female African frog, Xenopus laevis, are huge (approximately 1 mm in diameter), are easily harvested, and have few endogenous membrane-bound ion channels. These attributes permit scientist to inject exogenous mRNA into the oocytes, causing them to synthesize proteins, which then produce large quantities of membrane-bound ion channels.38 With this powerful tool, scientists can now directly study the relationship between channel structure and function.
(Reprinted with permission from Purves D, Augustine GJ, Fitzpatrick D, Katz LC, Lamantia A, McNamera JO et al. Neuroscience. 2nd ed. Sunderland, MA: Sinauer Associates, Inc.; 2001. p. 85.)
Scientists interested in subluxation and spinal manipulation hope to make similarly important contributions, but we got off to a late start. It is generally agreed that modern chiropractic research began in earnest after the 1975 National Institutes of Neurological and Communicative Disorders and Stroke (NINCDS) conference, “The Research Status of Spinal Manipulative Therapy.”41 At the end of this conference, it was acknowledged that there was little or no direct evidence to support or deny the claims of manipulative therapists. The evidence presented was general and circumstantial. It was especially and painfully obvious that there were very few published clinical or basic science studies by members of the chiropractic community.
Two years later, a follow-up conference, “Research Workshop on Neurobiological Mechanisms in Manipulative Therapy,” was sponsored at Michigan State University by the National Institutes of Health (NIH). Scott Haldeman was asked to present a paper titled The Clinical Basis for Discussions of Mechanisms in Manipulative Therapy.42 He was given a set of four basic criteria for judging any proposed neurobiological mechanism for spinal manipulative therapy (SMT):
1. SMT must produce consistent clinical results under controlled conditions in the treatment of a specific pathologic process, organ dysfunction, or symptom complex.
2. SMT must demonstrate a specific effect on the musculoskeletal system.
3. The musculoskeletal effect must have a specific influence on the nervous system.
4. The influence on the nervous system must demonstrate a beneficial influence on abnormal function of an organ, tissue pathology, or symptom complex.
In a recent article, “Neurologic Effects of the Adjustment,” Haldeman43 noted that these criteria remain as valid today as they were in 1977. In agreement with this observation, each study synopsis in this review ends with an assessment of which of the four criteria are addressed by the study. Haldeman’s criteria were specific to SMT research, but as discussed below, may also be applied to subluxation studies.
Review of Subluxation Research
With small modifications, a figure from Haldeman’s article43 provides an interesting overview of biomedical research (Figure 4-3). The original figure has been modified to encompass both SMT research and subluxation research. In Figure 4-3, criterion 1 highlights the outcome of SMT on organ dysfunction, tissue pathology, or symptom complex. This describes the focus of most clinical research studies. In these studies the patient is pretty much considered like the proverbial “black box.” There is little attempt to examine mechanisms within the black box that underlie the clinical study observations. By contrast, it is precisely these mechanisms that are the focus of basic science research. Basic scientists are most interested in what goes on within the black box (criteria 2, 3, and 4). Sometimes this simple dichotomous distinction between clinical and basic science studies is blurred. Clinical studies may include the examination of mechanisms, and basic science studies may explore the outcome of a treatment or lesion upon clinically relevant outcomes, as can be seen in this review of animal models. Whether the focus of a study is SMT or subluxation, the Haldeman criteria may be applied. Figure 4-3 presents the original criteria as they relate to examining the purported therapeutic benefit of SMT as well as the putative consequence of subluxation. Figure 4-3 suggests the necessary integration of SMT-focused research and subluxation-focused research. To date, no published studies have combined an SMT model with a subluxation model. However, as will be described at the end of this chapter, research is currently under way that will accomplish just that. A successful subluxation mimic model is being used in conjunction with various SMT mimic models to study the effects of different spinal manipulative therapies on animals with established pathologic consequences of subluxation.
There have been relatively few attempts by scientists at chiropractic institutions to model the chiropractic subluxation. Rather than reflecting the difficulty of the task, as Vernon5 suggested, this may actually reflect the small number of basic science researchers in chiropractic. Chiropractic colleges have a considerable number of basic science faculty, but the nearly fatal combination of heavy teaching loads, insufficient basic research facilities, and meager research funding makes this work a very rare occurrence at chiropractic institutions. The lack of follow-up on several proposed subluxation models that are reviewed here, despite positive initial studies, is symptomatic of this problem.
The four modified Haldeman criteria (see Figure 4-3) have been assigned to the reviewed studies as seemed appropriate. Some will assert that there are many more studies addressing each of the criteria. Besides the 40-year time frame (1964 to 2004), this review is limited to animal studies of the spine by researchers examining the subluxation, osteopathic lesion, or spine manipulation. Of course, the greater body of published scientific research contains many additional articles that may be relevant to understanding, evaluating, or developing subluxation and spinal manipulation theory. The reader is encouraged to explore that body of literature as well as the works presented here. It is hoped that this review will serve as both an historical overview and a “state-of-the-art” introduction to field practitioners and students.
The synopses are organized according to the primary construct they model (subluxation or spinal manipulation) and whether they represent a full mimic of the construct or just one of its components. To facilitate comparison and discussion, the reviewed subluxation studies are grouped as Subluxation Mimic Models, in which the study investigators attempted to mimic the subluxation, or Subluxation Component Models, wherein the investigators simply assume the subluxation produces a certain component effect and then examine the influence of that specific effect. Spinal Manipulation Mimic Models are dichotomized according to the method of manipulation, that is, manual or instrument.
Subluxation Mimic Models
Manual Subluxation Mimic Models
AUTHORS: S.D. Miroyiannis, H.C. Hunter, J.W. Chatfield44
TITLE AND SOURCE: Effect of vertebral malalignment on Nissl granules in rabbit spinal cord
Journal of the American Osteopathic Association 1964;63:862-3
METHODS: The rabbits were divided into three groups. Group 1 received a manually induced spine lesion. While under pentobarbital anesthesia, the upper lumbar spine was forcibly extended and rotated. Group 2 animals received a surgically implanted wire that was positioned to laterally malalign the spinous process of a lumbar vertebra relative to its contiguous neighbors (Figure 4-4). Group 3 animals served as nonmanipulated (normal) controls. The Nissl granule content of anterior horn cells was then compared between the control group and the two experimental groups at 2, 4, 6, 8, or 10 days following the experimental procedure.
FINDINGS: The Nissl content of cells in the manually manipulated group decreased progressively from the second to the tenth day. By contrast, the Nissl content of cells in the surgically malaligned group decreased in amount on the second day, but gradually increased such that by the tenth day following the surgery it was the same as the control group. The authors noted that these results were consistent with earlier work by Cole,45 in which a similar manual spine trauma produced decreased Nissl granule content in anterior horn cells.
COMMENT: This study was one of the last of its kind in a long line of studies published in the osteopathic literature wherein the spine of an animal was manually traumatized to produce an “osteopathic lesion.”45–48 The study design did not permit the authors to discriminate between the consequences of acute trauma associated with the manual manipulation or surgical procedure and a possible chronic consequence of these interventions, an osteopathic lesion (or subluxation). In study 2, the authors did control for the surgical trauma.
Modified Haldeman Criteria: #3
AUTHORS: S.D. Miroyiannis, B.A. Raines, V.J. Jurczenko, H.C. Hunter49
Journal of the American Osteopathic Association 1965;65:48-52
METHODS: The authors repeated the study previously conducted in rabbits (study 1), but with four groups of rats: (1) manual manipulation, (2) manual control, (3) surgical lesion, and (4) surgical control. The manual lumbar manipulation was as described above (under pentobarbital anesthesia) but was repeated until palpable paraspinal muscle spasticity was observed. The manual control group rats were anesthetized, but they received no procedure other than daily palpation. Rats in the surgical lesion group received the spinous displacing implant procedure described in study 1 (Figure 4-4), while animals in the surgical control group only had the wire placed next to but not displacing the T11-L1 [sic] spinous processes. In each group, half of the animals were sacrificed at 8 days and the other half were sacrificed at 15 days. Rats in the manual manipulation group received regular, additional manipulations under anesthesia during the survival period to maintain paravertebral spasticity. At the end of the 8- and 15-day survival periods, spinal cord sections were examined for both the quality and quantity of Nissl granule changes.
Modified Haldeman Criteria: #3
TITLE AND SOURCE: An attempt to induce vertebral lesions in rabbits by mechanical irritation
Journal of Manipulative and Physiological Therapeutics 1981;4:119-27
METHODS: DeBoer evaluated the use of a Grostic Adjusting Gun as a means of inducing subluxation in rabbits using a single group pre-post design. The C1 vertebra of each rabbit was impacted with the gun in addition to one thoracic vertebra (Figure 4-5). The Grostic gun was set with a 4-mm tip displacement and delivered 15 pulses at approximately 66.2 N/pulse (15 lb/pulse). The tip was placed against the left or right C1 transverse process to drive the vertebra laterally or at the base of a thoracic spinous process (approximately 45-degree angle) to produce rotary and anterior displacement. These lesion inducements were performed two times per day for four consecutive days for each animal. Three blinded observers determined the presence and location of subluxations before and after subluxation inducement in the spine regions C1, and T1 to T8. The observers were chiropractic students with advanced standing, who had all received training in palpation. They were specifically trained to palpate normal and “experimentally subluxated” rabbits for approximately six weeks prior to the beginning of the study. They recorded their palpation findings as level (C1, T1, T2…T8) and severity (0, no lesion, to 3, grossly obvious lesion). All rabbits were also radiographed (lateral and dorsal-ventral) before and after subluxation inducement.
FINDINGS: DeBoer reported a statistically significant higher “vertebral lesion score” from palpatory findings after subluxation inducement in the rabbits compared to the preinducement score. However, he found that interexaminer postinducement palpation scores were only marginally significant. Also, radiographic examination demonstrated no reliability. He noted that inducing subluxations with the Grostic gun produced considerable soft tissue edema in some rabbits as well as muscle splinting, which interfered with palpatory assessment. Because of this and the benefit of having tactile feedback, he suggested that future studies would probably use a manual method for producing subluxation lesions.
Modified Haldeman Criteria: #2
European Journal of Chiropractic 1984;32:131-42
COMMENT: Once again, DeBoer’s work demonstrated the twin problems of subluxation research—producing and verifying a successful subluxation lesion. As might be expected with problems obtaining a reliable vertebral lesion and the great variability in baseline EMG data, the results of this study were inconclusive. Despite this, he persisted in trying to develop an experimental subluxation lesion and studying the biological consequences of subluxation for an additional nine years. He succeeded in demonstrating gut EMG changes with a manual subluxation mimic in study 5.
Modified Haldeman Criteria: #2 and #3
AUTHORS: K.F. DeBoer, M. Schutz, M.E. McKnight52
METHODS: These investigators reported the acute effects of a manually induced vertebral lesion on gastrointestinal myoelectric activity in conscious rabbits. The subluxation mimic was produced by manually displacing the spinous processes of two contiguous vertebrae through the intact skin (Figure 4-6). They concurrently recorded EMG activity in the distal stomach and proximal duodenum in response to this vertebral displacement, and in additional control experiments. Control experiments consisted of a sham manipulation as well as noxious and nonnoxious stimulation of the skin overlying the vertebrae. The sham manipulation was accomplished by holding the same vertebral segments with slight ventral pressure, but no spinous rotation. The noxious stimulation was achieved by clamping a skin fold with a small forceps and nonnoxious stimulus consisted of light manual compression of the skin fold. The GI electrode placement and recording procedures are described in study 4. Manual vertebra displacement (the subluxation mimic) was applied to each rabbit at four different sites (T1-2, T5-6, T11-12, and L2-3) for about 2.5 minutes. The conscious rabbit remained calm during the subluxation lesion procedure after an initial startle response. No residual vertebral lesions were found upon subsequent palpation.
COMMENT: This interesting study provided support for the widely held view by chiropractic clinicians that visceral problems can be related to segmentally specific subluxations (the Meric System).53–55 This work, along with that of Sato and Swenson (study 18), Budgell et al. (studies 23, 26, 27, and 28), and Kang et al. (study 31) demonstrate the existence of segmental and suprasegmental somatovisceral reflex pathways with afferent limbs in spinal and paraspinal tissues.
Modified Haldeman Criteria: #1 and #3
Surgical Subluxation Mimic Models
AUTHORS: S.D. Miroyiannis, H.C. Hunter, J.W. Chatfield44
title: Effect of vertebral malalignment on Nissl granules in rabbit spinal cord
AUTHORS: S.D. Miroyiannis, B.A. Raines, V.J. Jurczenko, H.C. Hunter49
TITLE AND SOURCE: Researching the subluxation on the domestic rabbit
International Review of Chiropractic: Scientific Edition 1965;1(8):1-23
METHODS: Cleveland applied a transcutaneous fixation system in which the spinous attachment device consisted of a compression clamp with a needle that pierced the spinous process of a single vertebra. Three contiguous spinous attachment devices were linked together by a superstructure that held the devices in various positions of fixation, with or without malposition (Figure 4-7). He reported two case studies in rabbits wherein a misalignment of T12 was produced—“the 12th dorsal vertebra was subluxated posterior and right.”
Modified Haldeman Criteria: None. If control experiments had been performed, Haldeman criteria #1 would have applied.
Proceedings of the 7th Annual Biomechanics Conference on the Spine. 1976;27-50
METHODS: Awad reported a surgical procedure to produce a reversible vertebral fixation rat model. He implanted small posts in the contiguous spinous processes (or anterior vertebral bodies) of rats which were then linked by a small rubber band, producing “an intervertebral disrelationship” (Figure 4-8). Vertebra linked via the spinous process posts could be released at a later date by making a small skin incision over the site and cutting the rubber band.
FINDINGS: Awad observed scoliosis in rats linked dorsally (in extension) or ventrally at the vertebral bodies (in flexion), but not in unlinked (control) rats. He commented that rats linked dorsally developed scoliosis more readily than ventrally linked animals (Figure 4-9). However, he did not provide data to support this observation.
COMMENT: This small study introduced a reversible animal model of spine fixation and suggested an interesting connection between fixation and scoliosis. However, no additional work with this model was ever published. A very similar subluxation mimic was suggested by Lin et al. two years later (study 8). Awad commented that previous animal models used methods that were not reproducible. He was referring to previous studies in which an “osteopathic lesion” was created by manual manipulation of the spine.44,58–60 This chapter reviews five studies that applied manual methods to produce a subluxation mimic (studies 1 to 5).
Modified Haldeman Criteria: #2
AUTHORS: H.L. Lin, A. Fujii, H. Rebechini-Zasadny, D.L. Hart61
TITLE AND SOURCE: Experimental induction of vertebral subluxation in laboratory animals
Journal of Manipulative and Physiological Therapeutics 1978;1:63-6
ANIMAL: Rats; number of animals not reported
COMMENT: The number of animals was not reported in this study and these investigators did not examine the putative pathophysiologic effects of the fixations they created. No further investigations were published with this model.
Modified Haldeman Criteria: #2
TITLE: Gastrointestinal myoelectric activity in rabbits with vertebral lesions: preliminary report
AUTHORS: K.F. DeBoer, M.E. McKnight62
TITLE AND SOURCE: Surgical model of a chronic subluxation in rabbits
Journal of Manipulative and Physiological Therapeutics 1988;11:366-72
ANIMAL: 16 Rabbits; actual number uncertain due to article discrepancies
METHODS: Ten adult rabbits were equally distributed between a control group and an experimental group. At least six additional animals originally planned for the experimental group were not used due to subsequent implant failure. In the experimental animals, a stainless steel bar was slid along the base of the spinous processes of three adjacent vertebrae within the T3-T7 region such that the bar moved the middle vertebra into rotatory misalignment with respect to the two end segments (Figure 4-10). The two ends of the bar were then sutured in place to the spinous processes of the end vertebrae. In the control animals, bars were initially placed similar to the experimental animals but then were immediately withdrawn and placed in a neutral force position (alongside, not between, the spinous processes). Successful “subluxations” were determined by static palpation and plain film radiographs. Successful bar implants were verified by visual inspection at the time of surgery and at autopsy.
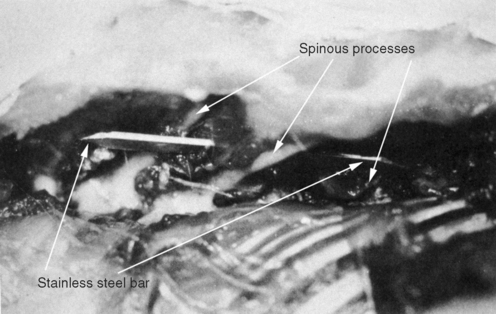
(Reprinted with permission from DeBoer KF, McKnight ME. J Manipulative Physiol Ther 1988;11:366-72.)
COMMENT: This study has been credited with introducing the first surgical subluxation mimic. While DeBoer’s work was certainly innovative and, until recently, has been the only continuous line of subluxation research in chiropractic, this was not the first surgical subluxation mimic. Four surgical models predate the DeBoer publication (studies 1, 2, 6, 7, and 8). Moreover, Miroyiannis introduced this very model in his 1964 and 1965 publications (studies 1 and 2). Unfortunately, this productive researcher published only one further study before leaving Palmer college for nonresearch pursuits (study 10).
Modified Haldeman Criteria: #2
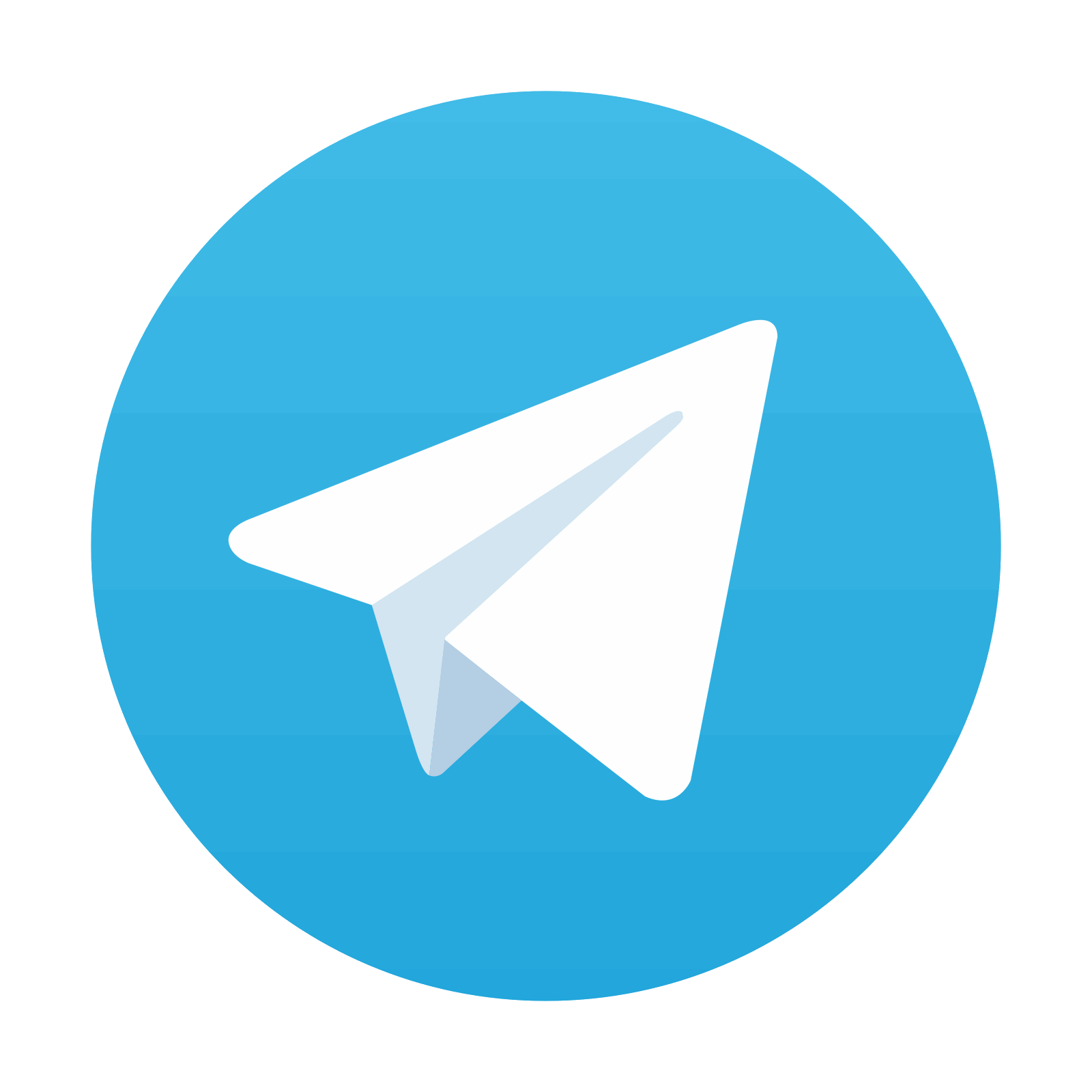
Stay updated, free articles. Join our Telegram channel

Full access? Get Clinical Tree
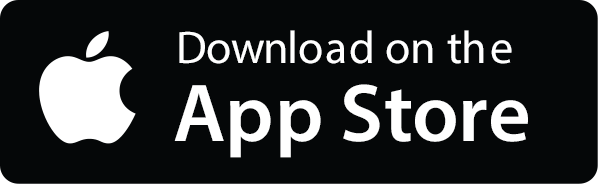
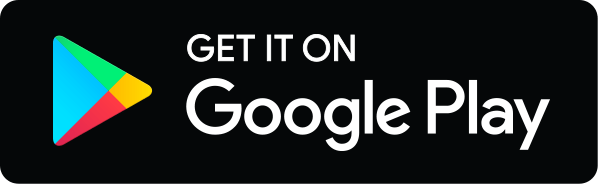