Chapter 49 Traumatic Brain Injury
Definitions
Defining Severity of Injury
TBI is often categorized as mild, moderate, or severe. The Glasgow Coma Scale (GCS) has become the most widely used primary initial assessment tool for determining the severity of injury (Box 49-1).426 During the initial stages of diagnosis in the field or emergency facility, TBI is suspected or indicated if there has been a significant blow to the head and/or there is an alteration of or loss of consciousness at the time of injury. An initial GCS score is obtained through clinical evaluation. As indicated in Box 49-1, the score is obtained by rating the best visual, verbal, and motor responses. The total score is simply a sum of these ratings, with scores ranging from 3 to 15. A score of 3T is given if the score obtained is due to medically induced chemical paralysis associated with intubation.
Generally accepted guidelines identify three levels of severity based on GCS scores: mild (GCS = 13 to 15), moderate (GCS = 9 to 12), and severe (GCS = 3 to 8). Individuals scoring in the mild TBI range should be evaluated at an emergency facility but might require minimal or no hospitalization. For more severe injuries involving loss of consciousness, the GCS is a widely accepted measure indicating the depth of coma and is generally serially measured until emergence from coma. However, the GCS has limited applicability to young children. The Pediatric Glasgow Coma Scale (PGCS) is a modified version of the GCS for use with pediatric TBI patients.309
Epidemiology
United States and Worldwide
Approximately 1.7 million TBIs occur each year in the United States.245 Of these, approximately 52,000 result in death and 1.365 million are mild injuries (often referred to as concussions), for which individuals are treated and released from the emergency room. This leaves approximately 275,000 individuals who survive moderate to severe TBI, generally requiring significant medical care and hospital stays. Many are left with long-term disability as a result of these injuries.245 According to one study, 40% of individuals hospitalized because of TBI reported at least one ongoing issue at 1 year after injury, with improvement of memory and problem solving listed as one of the most frequently unmet needs.86 Such cognitive impairments, in addition to physical and medical issues, have significant impact on the ability of an individual to perform everyday activities. In fact, it is estimated by the Centers for Disease Control and Prevention that at least 5.3 million Americans currently have a need for long-term or lifelong assistance with activities of daily living (ADLs) as a result of TBI.435
Globally, TBI is a leading cause of death and disability. Although exact worldwide statistics are difficult to obtain, a fairly recent review estimated approximately 775,000 new TBI hospitalizations per year in Europe.422 Available information suggests that the epidemiology of TBI on a global scale is similar to trends found in the United States. Incidence of TBI by age and gender varies slightly across regions but overall appears to follow patterns similar to those found in the United States.201 For example, the distribution of mild, moderate, and severe TBI is consistent across the United States, Europe, Australia, and Asia: 80% of injuries are mild TBI, whereas moderate and severe TBI each account for 10% of injuries.422
Causes of Injury
The leading causes of TBI in the United States include falls (35.2%), traffic-related crashes (17.3%), struck by/against events (sports) (16.5%), assaults (10%), and other injuries (21%).245 Sports injuries account for 1.6 to 3.8 million TBIs a year, although most of these are mild injuries that are not treated in the hospital or emergency department.246 It should be noted that these statistics vary with age, gender, geographic location within and between countries, and with group membership as a result of multiple factors, as explained below.
What is known of the global etiology of TBI is similar to U.S. rates, with a few notable exceptions. Sixty percent of TBIs in Europe are due to road traffic injuries.167 This figure is distributed evenly across most regions, suggesting that road traffic-related injuries are not associated only with high income countries. Twenty to 30% of injuries are due to falls, 10% violence, and 10% sports or work related.167 India has the highest reported rate of TBI related to falls.201,422 Latin America, Caribbean, and sub-Saharan Africa have the highest reported TBI rates as a result of road traffic incidents and violence.201
Associated Costs
The costs of TBI including direct medical costs and lost productivity in the United States were estimated to total $60 billion dollars in 2000.127 Worldwide statistics are less easily obtained. The costs associated with TBI go well beyond just the cost of medical care. The societal costs, or indirect costs, associated with the potential loss of productive work years for those who have been injured, as well as family members or others who might need to care for them, must also be considered. TBI also results in significant alterations in personal roles and responsibilities for the injured individual and family members. Reduced participation in complex leisure or recreational activities is also often a personal cost associated with TBI.
Demographics and Risk Factors
Age and Gender
The two age-groups most at risk for sustaining a TBI are 0 to 4 year olds and 15 to 19 year olds.245 Motor vehicle accidents result in the greatest number of TBI injuries for people aged 15 to 19.245 Another group at risk for TBI is adults over the age of 65. Fall-related injuries are highest among adults over the age of 65. Patients who sustain a TBI over the age of 75 have the highest rates of TBI-related hospitalization and death.245 Men are 1. 4 times as likely as women to sustain a TBI in the civilian population.245
Socioeconomic Status
Lower socioeconomic status (SES) in U.S. cities is correlated with an increased risk for injury as a result for increased exposure to high-risk occupations, personal violence, older vehicles, and substandard housing.235 Road traffic injuries are highest in areas of low SES.405 SES disadvantages are often associated with racial characteristics and rural locations. Minority populations in urban U.S. areas are often of lower SES and have elevated risk for injury.235 African Americans have a higher rate of TBI and associated mortality than other groups.235
Rural populations display a greater incidence of unhealthy lifestyle behaviors, including high alcohol consumption and psychosocial stress. Rural populations are also less likely to participate in preventive measures such as wearing seatbelts.322,440 This increase in risk-taking behaviors is evident in poorer health outcomes, specifically with injury-related outcomes like TBI.322,440
Violence
Assault or violence-related injuries account for 10% of TBI.245 Violence-related TBIs result from firearms, cutting instruments, blunt objects, or assaults such as pushing or hitting.145 Injury as a result of firearm use is the leading cause of death from TBI.442 Sixty percent of firearm-related TBI is self-inflicted, 32% results from intentional assault, and 4% is unintentional.38 People who sustain a TBI as a result of a violence-related injury are more likely to be younger, single, male, member of a minority group, and have a history of alcohol abuse.145
Child Abuse
Intentional TBI in children is referred to by many names, including shaken baby syndrome (SBS) and inflicted childhood neurotrauma.130 Approximately 1 million children are severely abused each year.486 Child abuse is most often conducted by parents or child care providers.362 The majority of perpetrators are male, either the child’s father or a boyfriend of the child’s mother residing in the home.389 Infants are at greatest risk for sustaining a TBI as a result of violent shaking,439 although older children are still at risk.381 One third of these children survive with no consequences; one third sustain permanent injury; and one third die.486 Risk factors for the occurrence of SBS include maternal factors such as mothers less than 19 years old, education less than 12 years, single marital status, African American or Native American, limited prenatal care, and newborns less than 28 weeks old.130 Child abuse rates resulting from unintentional injury increase during periods of economic decline.50
Psychosocial Factors
Part of the reason for the higher incidence of TBI for young males could be a tendency to engage in higher risk behaviors. Substance use is also related to TBI incidence, and up to 50% of individuals injured test positive for alcohol or other substances at the time of injury.83 Previous psychiatric history is a risk factor for TBI, particularly with those who have a history of anxiety, depression, and conduct disorder.445
Military Traumatic Brain Injury Epidemiology
An emerging group at risk for sustaining TBI is members of the U.S. military. Walter Reed Army Medical Center reports that 30% of service members evacuated from the field had sustained a TBI between 2003 and 2005. Army service members are relatively young with an average age of 28 years.341 Approximately 85% of military service members are male.341 Military members who sustain a TBI are also more likely to be male because they represent a larger percentage of the military service population.
Global Demographics and Risk Factors
What is known of global statistics suggests that countries with low to middle income harbor more risk factors that contribute to TBI. Risk factors vary by country and can depend on the incidence of such factors as poor road design, substandard vehicles, higher rates of violence as a result of war, and fewer prevention measures.201 Low- to middle-income countries typically have inadequate health systems to address TBI-related care.201 Accurate information on demographics and etiology of TBI in low- to middle-income countries is especially difficult to obtain. Despite this, the burden of TBI is evident worldwide.
Pathophysiology Associated With Traumatic Brain Injury
The pathophysiologic processes associated with TBI are complex and consist of (1) a primary injury that disrupts brain tissue and function at the moment of impact, (2) secondary injury through multiple biochemical cascades that propagate cellular dysfunction and lead to cell death, and (3) a chronic degeneration, repair, and regeneration process that occurs over the long term after the injury has occurred. Figure 49-1 depicts the continuation of injury and repair associated with pathophysiology after TBI.
Primary Injury
TBI occurs in conjunction with mechanical forces that cause disruption to the brain tissue. Closed head injuries can occur as a result of mechanical forces that shear axons, as well as by forces at the site of the impact or at the point opposite the impact.140 Contact forces occur when the head is prevented from moving after it is struck. Inertial forces occur when the head is set into motion and accelerates.178
Inertial forces associated with angular acceleration result in diffuse axonal injury (DAI). This is a process that causes tensile strains resulting in microscopic disruption of axons, cerebral edema, and neuronal disconnection. In angular acceleration, the brain’s center of gravity moves over a center of angulation, or fulcrum, located in the lower to middle cervical region.143 The severity of DAI depends on the duration, magnitude, direction of the angular acceleration, and associated impact.143 In severe cases of DAI, more than just superficial axons and deeper white matter structures are affected. The gray–white matter junction is also particularly vulnerable to DAI. Midline brain structures, such as the corpus callosum, are often affected by DAI, and DAI is also associated with loss of consciousness and coma. Recovery from DAI is gradual and can be linked to the duration of coma.178 Violent shaking associated with child abuse generates significant acceleration–deceleration forces, which result in shearing injury to brain tissue, disruption of blood vessels, and retinal bleeding.130
Inertial forces associated with translational acceleration result in a head movement that is in line with the brain’s center of gravity. The resulting differential movement of the brain relative to the skull causes focal injuries such as contusions. Cortical contusions often occur on gyral crests, particularly on the undersurface of the frontal and anterior temporal lobes, where bony prominences located in the basilar skull can create tissue and vascular disruption. Contusions can occur under the impact site (coup injury) and result from a rapid skull distortion during impact.143 Contusions remote from the injury site and opposite of the impact are contrecoup injuries and occur as a result of negative pressure generated from the impact, and are associated with translational acceleration.328 Patients with significant TBI can have one or more contusions, and deficits observed with cerebral contusions are linked with the lesion location and size.
DAI and focal contusions can result in neuronal disconnection, or diaschisis. The concept of diaschisis was first proposed by Constantin von Monakow in 1905 and refers to neurons remote from a site of injury, but anatomically connected to the damaged area, becoming functionally depressed. Because every structure in the brain is directly or indirectly connected to all other structures, the basic idea generated from this concept is that damage to one structure will be disruptive to other structures. Imaging studies suggest that diaschisis can result in the metabolic depression of areas both regionally associated and remote from the site of injury.121,295 Others suggest that focal cortical lesions can affect contralateral cortical functioning by way of intercallosal connections102 and can affect the function of subcortical structures, including the striatum.121 Resolution of diaschisis is one of several theories for recovery of function.121,295
Epidural hematomas (EDHs) result from local impact and subsequent laceration of underlying dural veins and arteries. The meningeal artery is commonly the source of EDHs, and damage to dural sinuses can also cause EDH. When an EDH develops from a disrupted artery, a neurologic emergency occurs because the EDH quickly expands and rapidly causes neurologic deterioration.178
Subdural hematomas (SDHs) result from inertial forces and the tearing of bridging veins.328 Bridging veins are susceptible to shear and rupture from brief high-velocity angular accelerations associated with falls.141 Traumatic subarachnoid hemorrhage (SAH) occurs when angular acceleration shears vessels located in the subarachnoid space.178
Secondary Injury
Secondary injury develops over the hours and days after the initial impact and is associated with disruption of cerebral blood flow and metabolism, massive release of neurochemicals, cerebral edema, and disruption of ion homeostasis leading to cellular injury and eventual cell death. Much of what is known about secondary injury is derived from postmortem analyses of human brain tissues, blood, cerebrospinal fluid (CSF), and parenchymal microdialysate fluid obtained early after clinical TBI. Much of the work exploring secondary injury has also used in vivo experimental models of TBI and in vitro injury methods. Experimental models of TBI produce one or more types of primary injury, which lead to the development of secondary injury cascades that closely resemble observations made in the human condition.104,276,404
Brain swelling occurs in response to the initial injury and early events involved with secondary injury and results in elevated intracranial pressure (ICP) and decreased cerebral perfusion pressure (CPP). If severe enough, brain swelling can lead to herniation, which has potentially fatal consequences. In some cases, elevated ICP is due to the development of focal extraaxial lesions like SDH and EDH described above. In other cases, elevated ICP and intracranial hypertension result from global mechanisms occurring on a cellular level that lead to brain edema. Some mechanisms that affect brain edema include vasogenic edema, increased tissue osmolarity, and vascular dysregulation resulting from increased cerebral blood volume. However, brain edema resulting from neuronal and astrocyte swelling secondary to increased glutamate metabolism after excitotoxic insult might impart the most damage.231
Excitatory amino acids (EAAs) like glutamate and aspartate are ubiquitous and important neurotransmitters for normal brain function. After TBI, excess excitatory amino acid levels are present in extracellular microdialysate fluid and CSF.53,452 Excess EAA release occurs, in part, from the mechanical stresses associated with stretch injury of axons. Elevations in extracellular glutamate levels after TBI contribute to excitotoxic injury by affecting other paths of secondary head injury through two primary mechanisms. By binding their target receptors, excess EAAs can trigger an influx of sodium and chloride that leads to acute neuronal and astrocytic swelling. Excess EAA levels can also lead to an intracellular calcium influx and the release of Ca++ from intracellular stores, leading to delayed cellular damage or death.70,415 EAA-mediated, Ca++-dependent production of nitric oxide, superoxide, and free radical damage to DNA and cellular membranes leads to cell death.265 Regional differences in the distribution of EAA-sensitive receptors contribute to selective vulnerability of some regions to excitotoxic injury like the hippocampus, a critical region for learning and memory.300
Lactate is the end product of glycolysis, and in neurons, lactate is converted to pyruvate for oxidative metabolism. Lactate/pyruvate ratios reflect the cellular energy balance and the cytosolic oxidation–reduction state.398 Increased metabolic demand after TBI is due, in part, to increased astrocytic glutamate uptake and results in increased lactate. Reduced cerebral blood flow, including pericontusional hypoperfusion, has been documented in both experimental models187 and in humans409 early after TBI and results in ischemic injury. Elevations in lactate/pyruvate have been measured in pericontusional tissue in humans448 and are associated with other aspects of secondary injury, including increased ICP and mitochondrial dysfunction.
Although extracellular lactate has classically been a marker for anaerobic metabolism with hypoxic injury, newer research indicates that lactate is also used by brain tissue during an insult as an important energy source in oxidative metabolism.356 Evidence supports a dichotomous role of lactate in which it is produced by the astrocyte via glycolytic metabolism, to be directly consumed by the neuron after uptake through lactate transporters,89,356 Physiologically337 and after TBI,356 excitatory amino acids are likely triggers to this metabolic process. After injury, estrogen facilitates lactate production for neuronal use by modulating lactate transporters.293 Experimental studies suggest that lactate is used as a neuronal energy substrate after TBI, and lactate administration after TBI is neuroprotective and decreases cognitive deficits.360
Mitochondrial dysfunction is often the result of energy failure and excitotoxic insult and results in the formation of highly reactive free radicals and subsequent oxidative damage to cell membranes and DNA. Hydroxyl radicals and other reactive oxygen species (ROS) react with many molecules found in living cells, including DNA, membrane lipids, and carbohydrates. Central nervous system (CNS) tissue is particularly vulnerable to oxidative injury because of the high rate of oxidative metabolic activity, the nonreplicating nature of neurons, high levels of transition metals, and a high membrane/cytoplasm ratio in neurons.10 Superoxide radicals are a principal mediator of the microvascular damage after TBI.242 Free-reactive iron generated from hemorrhage catalyzes the formation of free radicals.231 ROS production may also be a downstream effect of caspase-mediated cell death (apoptosis).126
Cell death in CNS tissues after brain trauma falls into two primary categories: (1) cellular necrosis and (2) apoptosis or programmed cell death. Necrotic cells display cellular and nuclear swelling as well as disruption of cellular membranes. In contrast, apoptotic cells are characterized by cellular shrinkage, nuclear condensation, and DNA fragmentation.216 Apoptosis is accompanied by a cascade of events required for cell death to occur.410 Cell death patterns in TBI often reflect a combination of both necrosis and apoptosis.
Apoptosis can be initiated through either extracellular or intracellular signaling pathways. Cysteine-aspartic acid proteases (caspases) play an essential role in cell death via both pathways. Extracellular binding of cell surface death receptors, including tumor necrosis factor (TNF)-α receptor and Fas ligand receptor, initiates apoptotic signals that include caspase-8 and caspase-3 within seconds of ligand binding. Intracellular signaling is initiated by mitochondrial dysfunction in response to disturbances in cellular energy balance, oxidative injury, and changes in ionic homeostasis. This mitochondrial stress leads to the opening of the mitochondrial transition pore and cytochrome c release. Cytosolic cytochrome c release induces multiple caspases, including caspase-9 and caspase-3, which leads to the cleavage of key cellular elements like cytoskeletal proteins and DNA repair proteins as well as the activation of endonucleases.77 In addition to this intracellular caspase-dependent process for apoptosis, intracellular apoptosis signaling can also occur via a caspase-independent pathway with apoptosis-inducing factor (AIF). AIF is released from the mitochondria and results in DNA fragmentation. The mitochondria house prosurvival and prodeath proteins that are both members of the BCL-2 protein family,2 each of which can be manipulated experimentally to effect cell survival. As an example, estrogen enhances BCL-2 expression and cell survival after CNS injury.199
Inflammation after TBI is characterized by complex cascades that largely include cytokines, or signaling molecules, that communicate with resident and infiltrating inflammatory cells. Cytokines like interleukin (IL)-1β and TNF are well studied in TBI. They are synthesized by peripheral cells and CNS neurons and glia, and are increased early after injury. IL-1β and other cytokines are also synthesized by activated microglia. IL-1β has potent leukocyte signaling properties that lead to cellular inflammatory responses after TBI. Cytokines generally can facilitate blood–brain barrier (BBB) disruption, cerebral edema, and cell death. Specifically, IL-1β and TNF can mediate synthesis of neurotoxic compounds such as arachidonic acid and are implicated in excitotoxic components of secondary injury.374
Cytokines are expressed in the brain and promote neuronal differentiation and survival under normal conditions. Cytokines play a dual role in secondary injury with TBI, and inflammatory cascades can either enhance cellular injury or provide neuroprotection. Some cytokines support neuroprotection and neurorepair through their effects on neurotrophin production, excitotoxicity, and antiinflammatory properties.223,307 Some studies suggest that cytokine effects might be dose dependent, with higher levels more likely to be associated with detrimental effects and lower levels being neuroprotective. Cytokine effects might also be time dependent, with beneficial effects more likely at delayed or chronic time points after injury.308 TNF is an example in which time-dependent functions have been demonstrated,387 with TNF expression being protective at later time points after injury.
Aside from BCL-2 and the prosurvival components of neuroinflammation, other CNS compounds serve as endogenous neuroprotectants after TBI. Adenosine is a purine ribonucleoside and is ubiquitous in the brain, where it largely functions as a major neuroinhibitory molecule. After experimental brain injury, extracellular adenosine levels increase by 50- to 100-fold compared with baseline,27 a phenomenon that is implicated as an endogenous neuroprotective mechanism. Adenosine functions as a neuroinhibitory molecule through its primary target, the A1 receptor. A1 receptor activation is a key local regulator of excitatory amino acid release during ischemia and trauma.12 Adenosine can also hyperpolarize neuronal membranes and attenuate intracellular Ca++ accumulation. Neurotrophins might also have a neuroprotective role after TBI, particularly because early increases in neurotrophin expression have been reported in experimental TBI.193,492
Concomitant Injury
TBIs (especially the more severe cases) often are not an isolated injury. High-speed vehicular crashes and active combat frequently result in multiple injuries that can affect outcome.230 In civilians, associated injuries are linked with more disability even 1 year after the injury.455 TBIs associated with multiple other injuries are also associated with longer acute care lengths of stay and need for rehabilitation resources.453 In addition to increased functional deficits, rehabilitation needs, and more disability, concomitant injuries can directly affect secondary injury and associated pathology, and these phenomena have received some attention in the TBI literature. For example, experimental models incorporating TBI and hemorrhagic shock show increased hippocampal injury compared with TBI alone, a phenomenon likely associated with increased secondary ischemia.92
Military Blast Injury
With the ongoing military conflicts in Iraq and Afghanistan, TBI is not only a common problem in the civilian population but also is a major problem for active combat military personnel. The frequent use of improvised explosive devices in these conflicts has resulted in a large number of military personnel (see previous epidemiology sections) sustaining TBI from blast injury. Blast injury can result in both blunt and penetrating trauma and follows a specific nomenclature (Box 49-2). Explosives can be categorized as high-order explosives (HE) or low-order explosives (LE), and each can cause a distinct injury pattern. HE produces a supersonic overpressurization shock wave that is associated with “primary injury.”441 In addition to middle ear damage, brain injury can occur from primary blast injury without overt evidence of injury. The rapid pressure changes generated from the overpressure wave result in shear and stress forces that lead to trauma such as concussion, SDH, and DAI.94,421 Penetrating head injury from flying debris is the result of secondary blast injury. Both HE and LE can result in tertiary blast injury from being physically thrown by a blast wind into the ground or other objects. Quaternary injury includes asphyxia or inhalation of toxic chemicals that can also contribute to brain injury. Many patients suffer from TBI induced from more than one facet of blast injury.
BOX 49-2 Immediate Effects of Blasts and Explosions in Traumatic Brain Injury
Modified from DePalma RG, Burris DG, Champion HR, et al: Blast injuries, N Engl J Med 352:1335-1342, 2008, and CDC Primer
Research is increasingly being conducted to develop relevant blast injury models of TBI to examine and characterize mechanisms of primary and secondary injury for blast TBI and to assess behavioral effects.21,60,264 The clinical difficulties after blast TBI of any severity can include headache, insomnia, decreased memory and attention, slower thinking, irritability, and depression.91 Additional research is focused on clinical symptom screening and outcomes, as well as physiologic markers and biomarkers associated with this type of TBI.7,418,436
Neurobiology of Neurotransmitter Dysfunction After Traumatic Brain Injury
The neurobiology of catecholaminergic dysfunction, particularly dopamine (DA) dysfunction, is the most characterized in the experimental literature.20 Early changes in DA levels occur after experimental TBI.278 Chronic DA receptor and synthetic protein changes after TBI also have been characterized using experimental TBI models. The DA transporter (DAT) is a key protein regulating the extracellular half-life of DA in the synaptic cleft, and the therapeutic target of neurostimulants like methylphenidate and amphetamine. Long-term changes in striatal and cortical DAT expression and intracellular trafficking have been identified451,462 that are gender specific.450 Functional changes in DAT after experimental TBI also lead to altered striatal neurotransmission.448,461 These experimental changes are corroborated by clinical neuroimaging studies demonstrating TBI-related decreases in DAT expression 1 year after injury.107,384 Other changes in postsynaptic secondary signaling mechanisms, as well as changes in presynaptic DA synthetic enzyme levels, have been identified.20
Behavioral pharmacology studies show that daily treatment with the DAT inhibitor methylphenidate (MPH) has a beneficial impact on cognition after experimental TBI.228 Recent work suggests that the cognitive benefits of MPH are more pronounced in males456 and that daily MPH therapy has a neurorestorative effect on striatal neurotransmission in males through MPH-mediated changes in DAT function.451,461 Daily treatment with the pleiotropic DA enhancer amantadine,103 as well as the D2 receptor agonist bromocriptine, also shows a benefit on learning and memory.226 Early treatment with bromocriptine also appears to decrease oxidative damage initiated by the injury.223
Evidence also suggests that norepinephrine (NE) systems are disrupted after experimental TBI but can be manipulated to improve recovery. Cortical NE levels are decreased in the first 24 hours after injury,348 and NE turnover is impaired chronically after injury.137 α1-Adrenergic receptor expression is also decreased.349 Local NE infusions early after injury enhance recovery,36 and stimulating adrenergic systems with amphetamine can decrease neuronal damage after TBI.153 Recent studies suggest that at least some of the beneficial effects of amphetamine might be due to its ability to increase neurotrophin production after injury.164 Daily treatment with low doses of the selective NE transporter (NET) inhibitor atomoxetine shows benefit on cognitive recovery after experimental TBI.355
Experimental TBI studies also demonstrate decreased hippocampal cholinergic transmission105 and alterations in cholinergic synthetic and metabolic enzyme production.67,105 Daily treatment with cytidine diphosphate (CDP)-choline, a key intermediary in the biosynthesis of phosphatidylcholine, improves cognitive recovery in experimental TBI and increases hippocampal and cortical acetylcholine levels.105 A single dose of the acetylcholinesterase inhibitor, rivastigmine, early after experimental TBI also improves cerebral edema, cognition, and motor functioning.67
Repair, Regeneration, and Recovery
One mechanism by which TBI recovery occurs is through the reversal of diaschisis. Contemporary thought on the concept of diaschisis suggests that the functional effects of diaschisis can be either reversible or permanent. The mechanisms by which diaschisis resolves, however, are poorly understood and likely multifactorial. The resolution of cerebral edema181 and blood flow regulation121 can contribute to early recovery.233 Later during the course, the alleviation of diaschisis might involve factors like synaptic plasticity,295,365 axonal sprouting,372 and cortical reorganization.238 Diaschisis after brain injury can be manipulated by both behavioral experience (rehabilitation) and pharmacologic intervention.325,392 For example, depressed thalamic metabolism after cortical infarction was reversed with administration of neurostimulants such as amphetamine.342
Experimental and clinical research suggests that DA systems are key pathways involved in attention, task salience, and cognition, and that these pathways are chronically impaired after TBI.20 Alterations in both presynaptic and postsynaptic dopaminergic proteins in the striatum and frontal cortex have been documented, including important proteins involved in DA synthesis and uptake.20,451,462,491 Decreases in striatal DAT expression, a key protein in regulating the extracellular half-life of DA, have been documented in humans.107 Further research demonstrates that striatal neurotransmission is impaired, and this might be the result of TBI-induced changes in DA protein functionality.451,462
Noradrenergic systems affect arousal, sleep–wake cycles, vigilance, and cognition, and the NET appears to affect NE neurotransmission, as well as interact with DA systems to affect DA neurotransmission.465 Noradrenergic function is impaired early after TBI. Previous reports suggest that cortical NE levels are decreased within 24 hours after brain injury, and adrenergic receptors are altered after injury.348,349 NE augmentation early after TBI is neuroprotective, however, and NE agonists aid in neurorecovery.37,108 It is important to remember that NE is critical for governing cortical plasticity and facilitating recovery post-TBI. By stimulating adrenergic systems with amphetamine and L-threo-dihydroxyphenylserine (or L-DOPS), a precursor of NE, neuronal damage after TBI can be minimized and sprouting is promoted.153,154,217,414,416
Cholinergic systems are important for memory and cognition, and evidence suggests chronic impairments can occur after TBI. Hippocampus expression of the vesicular acetylcholine transporter is increased; however, evoked release of acetylcholine in neocortex and hippocampus is reduced after experimental TBI.100,106,393 Daily local application of nerve growth factor also enhanced memory and hippocampal neuron function after experimental TBI.101
In addition to acute protection and cholinergic function, other studies support a role for neurotrophin administration in promoting long-term recovery. In contrast to acute increases in neurotrophin production, other work suggests that hippocampal brain-derived neurotrophic factor (BDNF) is decreased during chronic recovery from experimental TBI.66 An enriched environment can reverse these decreases,66 but chronic pericontusional administration of BDNF does not improve behavioral outcome or hippocampal cell survival.32 Intraparenchymal administration of both basic fibroblast growth factor and nerve growth factor reduces cognitive deficits in experimental TBI.289,402 With these positive studies, improved recovery might be secondary to stimulation of brain repair and neuroplasticity.
Evaluation and Treatment of Traumatic Brain Injury
Management of Mild Traumatic Brain Injury (Concussion)
Like more severe injuries, concussion results in significant alterations in brain physiology. After an initial increase in glucose use, brain metabolism is impaired and associated with the development of energy crisis, oxidative injury, and the initiation of biochemical cell death pathways. These derangements can occur for up to weeks after the concussion and persist even with a normal GCS score and general neurologic examination.151
There are several criteria and scoring systems for classifying degree or severity of mild TBI. The World Health Organization Collaborating Centre Task Force on Mild TBI states that key criteria for identifying persons with a mild TBI should include (1) at least one of the following: confusion, disorientation, loss of consciousness for less than 30 minutes, PTA for less than 24 hours, or other transient focal neurologic abnormalities, and (2) GCS score of 13 to 15 after 30 minutes or presentation to a health care facility. Findings with these criteria should be made in the absence of illicit drugs, alcohol, medications with sedating side effects, or other injuries or problems.58 Patients with uncomplicated TBI typically do not have associated abnormalities on standard imaging tests like computer tomography (CT). Concussion severity nomenclature has been developed for the purposes of injury characterization and injury management. Some examples of concussion are provided in Table 49-1.57,81,215
Patients with concussion frequently complain of any number of associated symptoms, including memory loss, poor concentration, impaired emotional control, posttraumatic headaches, sleep disorders, fatigue, irritability, dizziness, changes in visual acuity, depression, anxiety, personality changes, and seizures.9,481 These problems can affect ADLs, social relationships, and abilities in the workplace. For most individuals with mild TBI, the symptoms resolve over time. A subset of patients, however, will have persistent symptoms classified as postconcussional syndrome and can require outpatient follow-up evaluation. The types of symptoms, proportion of patients who have persistent symptoms, and the duration of symptoms required to define symptoms as persistent have been widely debated. Reports of symptom duration to define persistent deficits can range between 3 and 12 months. The fourth edition of the Diagnostic and Statistical Manual of Mental Disorders (DSM-IV) suggests that individuals showing objective evidence of cognitive deficits, as well as three or more subjective symptoms, that represent a change in baseline function for at least 3 months can be classified with postconcussional disorder. Other classification systems, such as the International Classification of Diseases, 10th edition (ICD-10), do not include objective evidence of cognitive deficits.481 Despite variability in definitions and classification, some studies suggest that females are more likely to have postconcussional syndrome.346
Athletes comprise a large portion of the population who sustain mild TBI, and many of these athletes are children. Special issues arise with this population with regard to “on site” concussion screening tools, determining the most effective algorithm for return to play, safety equipment use, management and prevention of recurrent injuries, and academic performance. As a general rule, athletes should be free of postconcussive symptoms before returning to competition. One report shows that 30% of high school football players return to play the same day of injury.168 Many return-to-play guidelines, however, outline that athletes with a grade 1 or 2 concussion have a minimum of a 7-day period free of symptoms before returning to play.169 Same-season injuries often occur within 7 to 10 days of the initial injury, a timeframe in which there is increased vulnerability to damage with the second insult.151 Most return-to-play algorithms emphasize a graded protocol for reintroducing the athlete to physical exertion. Recent studies, however, suggest that academic and cognitive exertion can affect postconcussive symptoms and cognitive testing performance and should be considered when developing return-to-play protocols for athletes.271 Several guidelines recommend termination of sports participation for the remainder of the season if an athlete sustains a third concussion within that season.57,358 Athletes who have one concussion are at increased risk for subsequent concussion,169 and making a return to contact sports even after a single concussion is a decision that requires careful thought and consideration.
Acute Medical Management of Moderate to Severe Traumatic Brain Injury
The care of the patient with TBI begins in the field. Evidence-based guidelines put forth by the Brain Trauma Foundation and the American Association of Neurological Surgeons support a regional organized trauma care system that addresses prehospital care and triage. Direct transport of a patient with severe TBI to a Level 1 or 2 trauma center is associated with decreased mortality compared with indirect transport.183 Facilities defined by the Guidelines for Prehospital Management of Traumatic Brain Injury as being appropriate facilities for transport include those offering CT scanning, neurosurgical care, ICP monitoring, and treatment capabilities. These facilities can provide 24-hour neurosurgical care, and intensive care treatment ICP monitors are routinely used. Each state can designate trauma facilities based on the availability of such care. Although treatment guidelines for initial management are not specific, the recommendations include complete and rapid physiologic resuscitation. Correction of hypoxia, including intubation, is appropriate. Sedation and neuromuscular blockade might need to be used to optimize the transport of the head injury patient.41
ICP has been the mainstay of monitoring after severe TBI. ICP can be monitored using a ventriculostomy, which is the procedure of choice because it allows therapeutic CSF drainage. ICP monitoring is appropriate in those patients with a GCS score of 8 or less after resuscitation, head CT showing contusions or edema, or systolic blood pressure less than 90 mm Hg.316 ICP monitoring can also be considered in patients with severe TBI and a negative head CT if they are more than age 40, posturing, and/or have systolic blood pressure less than 90 mm Hg.40 Elevated ICP is defined as 20 to 25 mm Hg in clinical practice. Maneuvers to decrease ICP include elevating the head of the bed 30 degrees, treatment of hyperthermia, mannitol administration, sedation, and brief hyperventilation. Prolonged chronic hyperventilation can negatively affect cerebral blood flow.39
CPP is defined as the mean arterial pressure minus ICP and is the pressure gradient driving cerebral blood flow. Studies have documented worsened clinical outcomes in TBI patients with episodes of low CPP.69 The current therapeutic recommendation is that CPP be maintained at more than 60 mm Hg in adults.42 Measurement of cerebral oxygen tension allows the practitioner to directly measure ischemia, rather than rely on the indirect measurement of CPP or ICP. Tissue oxygenation monitors, directly placed in brain tissue via an external ventricular drain (EVD), can detect focal ischemic changes that can go undetected with global measures. Interventions are then tailored to the cause of the decrease in tissue oxygenation. Studies have shown reduction in mortality with addition of this monitoring.411
Other treatment interventions have been studied in acute care clinical trials. A multicenter trial evaluating the role of corticosteroids in management of acute TBI showed an increase in mortality 2 weeks after TBI compared with placebo.367 Elevated CSF cortisol levels in severely injured patients might contribute to this outcome.368 Progesterone is a drug with pleiotropic neuroprotective properties identified in several preclinical studies occurring over decades.408 Recent clinical trials have shown its safety, and progesterone appears to have potential as a therapeutic agent.482,487
Animal studies have evaluated the protective use of hypothermia, with several showing positive results.76,96 Clinical trials using moderate hypothermia have shown some reduction in excitotoxic insult274,452 and neurologic impairment; however, the results have not been consistent. For example, a large multicenter trial of hypothermia did not show improved outcomes in those patients with severe TBI.78 To transition this treatment to routine human use, factors such as treatment window, rewarming rates, and type of anesthesia must be defined.407 Gender effects on treatment efficacy and physiologic response to treatment should also be considered.23,417,452
Surgical treatment of TBI is indicated when intracerebral fluid collections exert a significant mass effect. Mass effect can be gauged by degree of midline shift on neuroimaging, and the decision to intervene can require serial neurologic examinations and CT scans.52 Depressed skull fractures greater than the thickness of the skull should be elevated to decrease the risk for infection, especially in the setting of a dural laceration. Surgical treatment can also be used to lower ICP. The use of decompressive craniectomy to decrease ICP is an accepted intervention, although the reduction of morbidity and mortality has not been proven.477
Secondary complications occurring in the intensive care unit (ICU) can also negatively affect TBI outcomes. Elevated glucose levels are associated with increased mortality after TBI, presumably as a result of increased lactic acid production leading to ischemia and impaired phosphorus metabolism.375 Sodium imbalance can potentiate risks for seizure, as well as worsen level of consciousness. Sodium imbalance is directly related to volume status. Hyponatremia can be exacerbated by the use of excessive volumes of intravenous fluids. This occurs even in the setting of normal saline or lactated Ringer’s solution used for fluid resuscitation, making monitoring of electrolytes and volume status important in preventing secondary complications. Prevention and aggressive treatment of infections are also important. Hyperthermia is another source of acceleration of secondary insults to brain parenchyma, increasing ischemia.273
Early initiation of nutrition is vitally important in the TBI patient. Metabolic rates can increase 40% during the early stages after injury. Guidelines recommend replacement of 140% of resting metabolism expenditure (100% of resting metabolism expenditure in paralyzed patients). The use of the gastrointestinal tract is preferred to maintain nutritional status. The goal of treatment is to maintain a positive nitrogen balance, although this is difficult to achieve. Measures such as nitrogen balance via a metabolic cart study, prealbumin levels, and liver functions should be followed in the ICU. Delaying nutrition replacement is associated with increased mortality.184
The physiatrist plays a role in evaluating patients who have sustained TBI. The early assessment includes prevention of orthopedic and immobility complications. Early consultation can result in improved mobility, improved functional outcomes, and decreased acute care length of stay. In this model, rehabilitation assessment is a portion of the acute medical care, providing education and support for patients, families, and caregivers.453
Physiologic Measurements During Acute Care
Somatosensory-evoked potentials (SSEPs) are recorded from the scalp after stimulation of a sensory or mixed nerve in the peripheral nervous system. SSEPs are often used in severe TBI cases to predict survival and evaluate posttraumatic coma and persistent vegetative states. In some cases, SSEP testing is used as an adjunct to the clinical examination in determining brain death.174 Cant et al.56 found that bilateral loss of the median SSEP was indicative of impending death, and asymmetric findings observed in the cortical response were indicative of severe residual deficits. Their study indicated that serial SSEPs often change through the hospital course, and each study does not always correlate well with the ultimate outcome.
Like other neurologic evaluations, electroencephalography (EEG) can be beneficial when assessing TBI patients to detect injury severity and depth of coma.378 Degree of unconsciousness can quickly change, and continuous EEG (cEEG) monitoring can detect electrophysiologic signs of clinical deterioration.175 Initial EEG recordings taken within 24 hours of injury, however, are generally more abnormal and of less prognostic significance than those performed after 24 to 48 hours.419 cEEG is a standard part of care at some Level 1 trauma centers, and cEEG can be used to effectively identify subclinical electrographic seizures, which are associated with other physiologic factors that contribute to poor outcome, such as ICP elevations and increases in metabolic stress.447
Pupillary reflexes have been used not only as clinical indicators of surgical emergencies and complications in TBI associated with edema and herniation but also as a tool in determining lesion location, mortality, and global outcome after injury. The presence or absence of pupillary reflexes and degrees of anisocoria have been used in models predicting the probability of intracerebral hematoma and lesion location.68
Although not yet used routinely at the bedside, biomarkers have considerable potential as adjunctive tools in TBI diagnosis, prognosis, and monitoring treatment effect. Protein biomarkers that have been studied in TBI include either (1) markers of structural damage or (2) markers reflective of the cellular and molecular cascades observed with secondary injury and repair. Protein biomarkers have also been studied in both CSF and serum. CSF biomarker studies are valuable in that measured levels are largely a reflection of CNS pathology. CSF collection is only routine, however, in severely injured patients who require an extraventricular drain for management of ICP. Serum markers are attractive because of their potential application to mild and severe cases of TBI, but serum biomarker levels are often limited by the extent of BBB permeability present after the injury. Markers of acute injury have been much more thoroughly studied compared with chronic markers of injury.
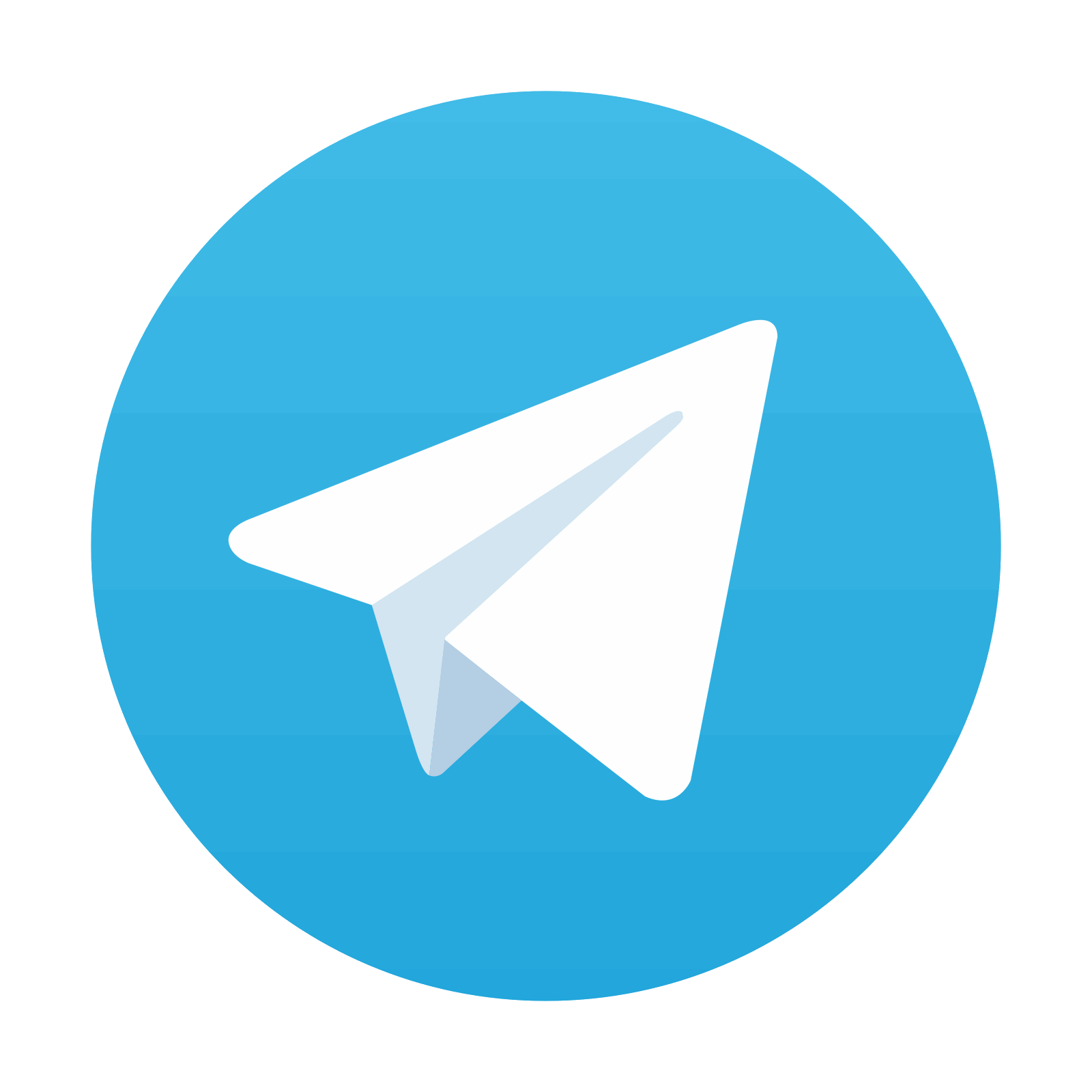
Stay updated, free articles. Join our Telegram channel

Full access? Get Clinical Tree
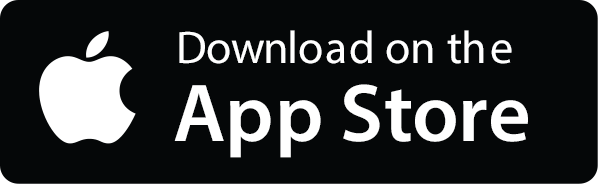
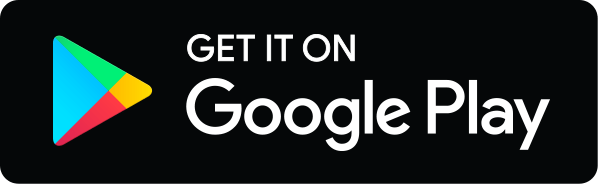