, Wellington K. Hsu1, Phuc Dang2, Ranjan Gupta2, Bastián Uribe-Echevarría3, Brian R. Wolf3, Matthew T. Provencher4 , Daniel J. Gross5 , Amun Makani5 and Petar Golijanin5
(1)
Department of Orthopaedic Surgery, Northwestern University Feinberg School of Medicine, Chicago, Illinois, USA
(2)
Department of Orthopaedic Surgery, University of California – Irvine, Orange, California, USA
(3)
Department of Orthopaedics and Rehabilitation, University of Iowa, Iowa City, Iowa, USA
(4)
Sports Medicine and Surgery, Harvard Medical School, Massachusetts General Hospital, 175 Cambridge St. Suite 400, Boston, MA 02114, USA
(5)
Sports Medicine and Surgery, Massachusetts General Hospital, 175 Cambridge St. Suite 400, Boston, MA 02114, USA
1 Bone
Sharath S. Bellary6 and Wellington K. Hsu6
(6)
Department of Orthopaedic Surgery, Northwestern University Feinberg School of Medicine, Chicago, Illinois, USA
Take-Home Message
Bone regeneration depends on an osteoinductive stimulus, osteoconductive matrix, source of responding cells, and sufficient vascular supply.
The mechanical environment will influence bone grown in accordance with Wolff’s Law.
If one or more of these factors is lacking, new bone formation is significantly decreased.
Osteoinduction
Characterized by the process of recruitment of immature pluripotent cells.
Subsequent stimulation causes differentiation into pre-osteoblasts.
Several growth factors have been identified as critical components in osteoinduction (Table 1 and Figure 1).
Fig. 1
Factors influencing bone formation
Table 1
Mediators of bone formation
Type
Source
Action
Bone morphogenetic protein −2, −7 (TGF-β[beta] superfamily)
MSCs, ECM, vascular endothelium
Recruitment and osteoblastic differentiation of mesenchymal cells, ossification of ECM
Fibroblast growth factor – 18
Vascular endothelium basement membrane
Bone development
Insulin-like growth factor
Liver
Activation of osteocytes, anabolic for bone tissue
Platelet-derived growth factor
Platelets, smooth muscle cells, activated macrophages, vascular endothelium
Mitogen for mesenchymal cells, angiogenesis
Vascular endothelium growth factor
Vascular endothelium, smooth muscle
Angiogenesis
Hydroxyapatite
Osteocytes, major component of bone ECM
Contributes to density and strength of bone, initiation of osteoblastic differentiation from MSCs
Osteoprotegerin (TNF-α[alpha] superfamily)
Vascular endothelium and smooth muscle, osteocytes
Blocks RANK ligand interaction with RANK receptor
RANK ligand
Vascular endothelium and smooth muscle, osteocytes
Osteoclastic differentiation and activation
The most widely studied are those in the bone morphogenetic protein (BMP) family, of which over 20 have been identified.
BMPs are members of the TGF-β [beta] superfamily of growth hormones with BMP-2 and BMP-7 having the most development for therapeutic applications.
BMP supplementation is FDA-approved to augment spinal arthrodesis and to treat nonunion or open fractures of long bones.
Absorbable collagen sponges have been commercialized as a method of local delivery of BMP to surgical sites; endogenous BMP molecules are also naturally released during trauma to bone and during bone remodeling.
Inflammatory cytokines and damage to the bone ECM can liberate matrix-bound growth factors which induce differentiation and proliferation of osteoprogenitor cells.
Under the influence of an osteoinductive environment, these cells terminally differentiate into osteoblasts and begin to deposit calcified extracellular matrix (ECM).
Osteoconduction
The process of bone growth on a surface or scaffold, the initial deposit of osteoid and its conversion to woven and lamellar bone, is an example of this process.
Implantation of bone grafts and scaffolds are other examples of osteoconduction.
There are many characteristics of a scaffold that optimize its ability to aid bone regeneration such as compressive strength, biocompatibility, adhesive properties, and pore size.
It has been previously reported that osteoconduction does not occur on some materials such as copper and silver, with increasing success on materials of higher biocompatibility such as titanium.
Due to stress shielding, bone growth around and within a scaffold is optimized when the Young’s modulus (stiffness) of the scaffold closely correlates to that of bone; pore size of the scaffold can be adjusted to meet this requirement in nonabsorbable scaffolds.
Increased porosity allows for a greater surface area upon which recruited cells may grow, too much of which makes the matrix unstable.
Scaffolds are also often coated with or made from biologic agents to encourage cell recruitment and adhesion or provide extended release of osteoinductive compounds.
Materials such as chitin, Gelfoam, hydroxyapatite, polyethylene glycol, or collagen have been investigated.
With rapidly absorbable scaffolds, the rate of degradation should correlate with the rate of new bone growth, presenting a challenge in the design of novel material implants.
Responding Cells
There are four primary cell types in bone tissue: osteocytes, osteoblasts, osteoclasts, and osteoprogenitor cells.
A source of responding cells is required to provide a renewable source of primary osteoblasts and osteoclasts that will aid in the continuous formation and remodeling of bone.
Cells can either be delivered through a cell-based strategy or endogenously confined through binding epitopes. Each strategy is designed to offer a long-term source of osteogenic activity.
Cell-based therapies often require concomitant regeneration of vasculature in addition to bone and therefore osteogenic and vasculogenic cell types have been grown in coculture to promote heterotypic interactions.
During an acute fracture, bone marrow is the main source of stem cells for bone repair. Mesenchymal cells under the influence of chemotactic agents are recruited to the site of injury, where local osteoinductive effects encourage differentiation of these cells into pre-osteoblasts.
These osteoprogenitor cells further differentiate into osteoblasts and after a period of months may terminally differentiate into osteocytes.
Osteoblasts are responsible for bone formation by depositing osteoid and mineralizing it. The absolute number of osteoblasts tends to decrease with age.
Osteocytes are long-lived stellate cells that are trapped within the matrix they secrete.
Osteoclastic cells are derived from hematopoietic progenitor cells which differentiate under influence from mesenchymal stromal cells. The balance between osteoblast and osteoclast activity is responsible for the continuous remodeling of bone tissue.
Vascular Supply
Bone is a highly vascular tissue, receiving 10–20 % of the resting cardiac output.
Fracture healing is a delicate physiological process dependent on adequate vascular supply to damaged tissue.
Preservation of tissue planes during surgical exposure is an important tenet to avoid disrupting the existing blood supply to the area.
Osteocytes receive nutrition via cell–cell communication through bone canaliculi, while Volkmann and Haversian canals allow routes for blood vessels to penetrate through bone tissue.
The ultimate strength of bony repair hinges on the vascular supply to the anatomic area. For example, fractures which occur in a rich vascular environment (distal radius metaphysis) have a much higher healing rate than those with poor circulation (scaphoid).
Avascular necrosis (AVN) of bone tissue has been well described (femoral head AVN, Kienbock’s disease). It is also well known that poor vascular supply is associated with unfavorable outcomes following surgery.
VEGF has been shown to be the most important growth factor for angiogenesis and bone healing at a fracture site. Hypoxia has been shown to enhance angiogenic response and levels of VEGF in rodent bone marrow cells.
Local delivery of these factors may lead to enhanced angiogenesis which may affect outcomes. Vascular tissue is an important source not only for nutrition and a route for responding cells, but also for osteoinductive stimuli.
Bibliography
1.
Albrektsson T. The healing of autologous bone grafts after varying degrees of surgical trauma. A microscopic and histochemical study in the rabbit. J Bone Joint Surg Br. 1980;62:403–10.
2.
Albrektsson T, Johansson C. Osteoinduction, osteoconduction and osseointegration. Eur Spine J. 2001;10(Suppl 2):S96–101.
3.
Beenken A, Mohammadi M. The FGF family: biology, pathophysiology and therapy. Nat Rev Drug Discov. 2009;8:235–53.
4.
Caplan AI. Mesenchymal stem cells. J Orthop Res. 1991;9:641–50.
5.
Chan O, Coathup MJ, Nesbitt A, Ho CY, Hing KA, Buckland T, Campion C, Blunn GW. The effects of microporosity on osteoinduction of calcium phosphate bone graft substitute biomaterials. Acta Biomater. 2012;8:2788–94.
6.
Cui Q, Botchwey EA. Emerging ideas: treatment of precollapse osteonecrosis using stem cells and growth factors. Clin Orthop Relat Res. 2011;469:2665–9.
7.
Harada Y, Wang JT, Doppalapudi VA, Willis AA, Jasty M, Harris WH, Nagase M, Goldring SR. Differential effects of different forms of hydroxyapatite and hydroxyapatite/tricalcium phosphate particulates on human monocyte/macrophages in vitro. J Biomed Mater Res. 1996;31:19–26.
8.
Hoffmann BR, Wagner JR, Prisco AR, Janiak A, Greene AS. Vascular endothelial growth factor-A signaling in bone marrow-derived endothelial progenitor cells exposed to hypoxia. Physiol Genomics. 2013;45:1021–34.
9.
Kempen DH, Lu L, Heijink A, Hefferan TE, Creemers LB, Maran A, Yaszemski MJ, Dhert WJ. Effect of local sequential VEGF and BMP-2 delivery on ectopic and orthotopic bone regeneration. Biomaterials. 2009;30:2816–25.
10.
Keramaris NC, Calori GM, Nikolaou VS, Schemitsch EH, Giannoudis PV. Fracture vascularity and bone healing: a systematic review of the role of VEGF. Injury. 2008;39(Suppl 2):S45–57.
11.
Kleinheinz J, Stratmann U, Joos U, Wiesmann HP. VEGF-activated angiogenesis during bone regeneration. J Oral Maxillofac Surg. 2005;63:1310–6.
12.
Kubota T, Elalieh HZ, Saless N, Fong C, Wang Y, Babey M, Cheng Z, Bikle DD. Insulin-like growth factor-1 receptor in mature osteoblasts is required for periosteal bone formation induced by reloading. Acta Astronaut. 2013;92:73–8.
13.
Lamplot JD, Qin J, Nan G, Wang J, Liu X, Yin L, Tomal J, Li R, Shui W, Zhang H, Kim SH, Zhang W, Zhang J, Kong Y, Denduluri S, Rogers MR, Pratt A, Haydon RC, Luu HH, Angeles J, Shi LL, He TC. BMP9 signaling in stem cell differentiation and osteogenesis. Am J Stem Cells. 2013;2:1–21.
14.
Lounev VY, Ramachandran R, Wosczyna MN, Yamamoto M, Maidment AD, Shore EM, Glaser DL, Goldhamer DJ, Kaplan FS. Identification of progenitor cells that contribute to heterotopic skeletogenesis. J Bone Joint Surg Am. 2009;91:652–63.
15.
Luginbuehl V, Zoidis E, Meinel L, von Rechenberg B, Gander B, Merkle HP. Impact of IGF-I release kinetics on bone healing: a preliminary study in sheep. Eur J Pharm Biopharm. 2013;85:99–106.
16.
Matsubara H, Hogan DE, Morgan EF, Mortlock DP, Einhorn TA, Gerstenfeld LC. Vascular tissues are a primary source of BMP2 expression during bone formation induced by distraction osteogenesis. Bone. 2012;51:168–80.
17.
Nagayama T, Okuhara S, Ota MS, Tachikawa N, Kasugai S, Iseki S. FGF18 accelerates osteoblast differentiation by upregulating Bmp2 expression. Congenit Anom (Kyoto). 53:83–8.
18.
Rao RR, Stegemann JP. Cell-based approaches to the engineering of vascularized bone tissue. Cytotherapy. 2013;15:1309–22.
19.
Ross M. Bone In: Pawlina W, editor. Histology: a text and atlas. Philadelphia: Lippincott Williams & Wilkins; 2010. p. 218–53.
20.
Sanghani A, Chimutengwende-Gordon M, Adesida A, Khan W. Applications of stem cell therapy for physeal injuries. Curr Stem Cell Res Ther. 2013;8:451–5.
21.
Stevenson S, Emery SE, Goldberg VM. Factors affecting bone graft incorporation. Clin Orthop Relat Res. 1996;(324):66–74.
22.
Szpalski C, Nguyen PD, Cretiu Vasiliu CE, Chesnoiu-Matei I, Ricci JL, Clark E, Smay JE, Warren SM. Bony engineering using time-release porous scaffolds to provide sustained growth factor delivery. J Craniofac Surg. 2012;23:638–44.
23.
Tsiridis E, Upadhyay N, Giannoudis P. Molecular aspects of fracture healing: which are the important molecules? Injury. 2007;38(Suppl 1):S11–25.
24.
Wang X, Wang Y, Gou W, Lu Q, Peng J, Lu S. Role of mesenchymal stem cells in bone regeneration and fracture repair: a review. Int Orthop. 2013;37:2491–8.
25.
Wang H, Zhi W, Lu X, Li X, Duan K, Duan R, Mu Y, Weng J. Comparative studies on ectopic bone formation in porous hydroxyapatite scaffolds with complementary pore structures. Acta Biomater. 2013;9:8413–21.
26.
Yang F, Wang J, Hou J, Guo H, Liu C. Bone regeneration using cell-mediated responsive degradable PEG-based scaffolds incorporating with rhBMP-2. Biomaterials. 2013;34:1514–28.
27.
Yang J, Wang J, Yuan T, Zhu XD, Xiang Z, Fan YJ, Zhang XD. The enhanced effect of surface microstructured porous titanium on adhesion and osteoblastic differentiation of mesenchymal stem cells. J Mater Sci Mater Med. 2013;24(9):2235–46.
2 Peripheral Nervous System
Phuc Dang7 and Ranjan Gupta7
(7)
Department of Orthopaedic Surgery, University of California – Irvine, Orange, California, USA
Take-Home Message
Axons are surrounded by three layers of connective tissue including the endoneurium, the perineurium (blood–nerve barrier), and the epineurium.
Seddon’s classification of nerve injury: neurapraxia, axonotmesis, neurotmesis.
Early tension-free nerve repair in young patients results in best chances for functional recovery.
Definitions
The primary cellular components of peripheral nerves are axons from neurons and glial cells. Neurons are polarized cells with dendrites and axons that process and transmit information through electrochemical signals.
In the peripheral nervous system (PNS), the primary glial cell is the Schwann cell which serves to ensheathe axons in myelin and provides trophic support through release of important neurotrophic factors.
Peripheral nerves are part of the PNS that convey signals between the spinal cord to the limbs and organs. Nerves are composed of cellular processes from multiple different neurons including motor, sensory, and autonomic. The efferent neurons (motor and autonomic) receive signals from the central nervous systems (CNS) and transmit messages to their target end organs. Afferent nerves receive signals from specialized cell types, such as Pacinian corpuscles for fine sensation, and relay this information to the CNS.
Embryology
The PNS are primarily derived from neural crest cells. Neural crest cells are groups of ectodermal cells that delaminate from the dorsal neural tube in early development. These cells then migrate throughout the body and develop into neurons, glia, and neurosecretory cells of the PNS.
Histology/Physiology
A neuron usually ranges from 5 to 150 μm in diameter and contains a cell body and at least two cellular processes, the dendrite and the axon (Fig. 2). Impulses from the dendrites are directed toward the cell body, which in turn generate an action potential which is directed down the axon toward the synapse, or a target end organ such as a neuromuscular junction (NMJ). Axons can be myelinated (large fibers such as motor) or unmyelinated (small fibers such as type C pain fibers) (Table 2). Myelin, produced by the Schwann cells, acts as insulation surrounding the axon and serves to reduce the dissipation of action potential into the surrounding environment. Within myelinated axons, there are gaps without myelin called nodes of Ranvier, which allows propagation of action potential via saltatory conduction so as to increase nerve conduction velocity without increasing the diameter of an axon.
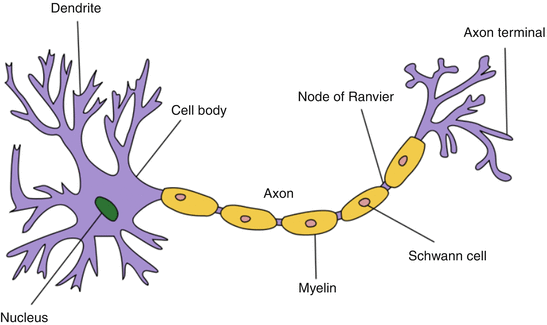
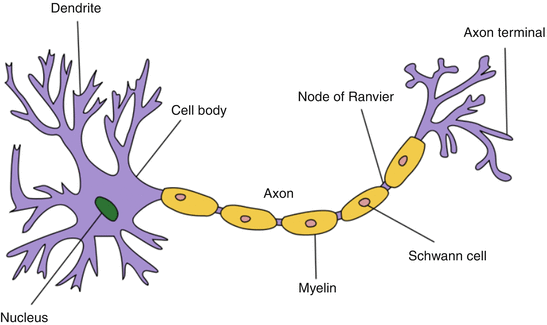
Fig. 2
Neuron morphology
Table 2
Nerve fiber types and function
Nerve fiber types | Function | Myelination | CV (m/s) | |
---|---|---|---|---|
A | alpha | Motor – skeletal muscle | Yes | 100 |
beta | Touch, pressure | 30–70 | ||
delta | Muscle spindle, proprioception | 15–30 | ||
gamma | Fast pain (cold/touch) | 12–30 | ||
B | Preganglionic autonomic | Yes | 3–15 | |
C | Pain (thermal), mechanoreceptor | No | 0.5–2 | |
Postganglionic autonomic | 0.7–2.3 |
Cross-Sectional Anatomy
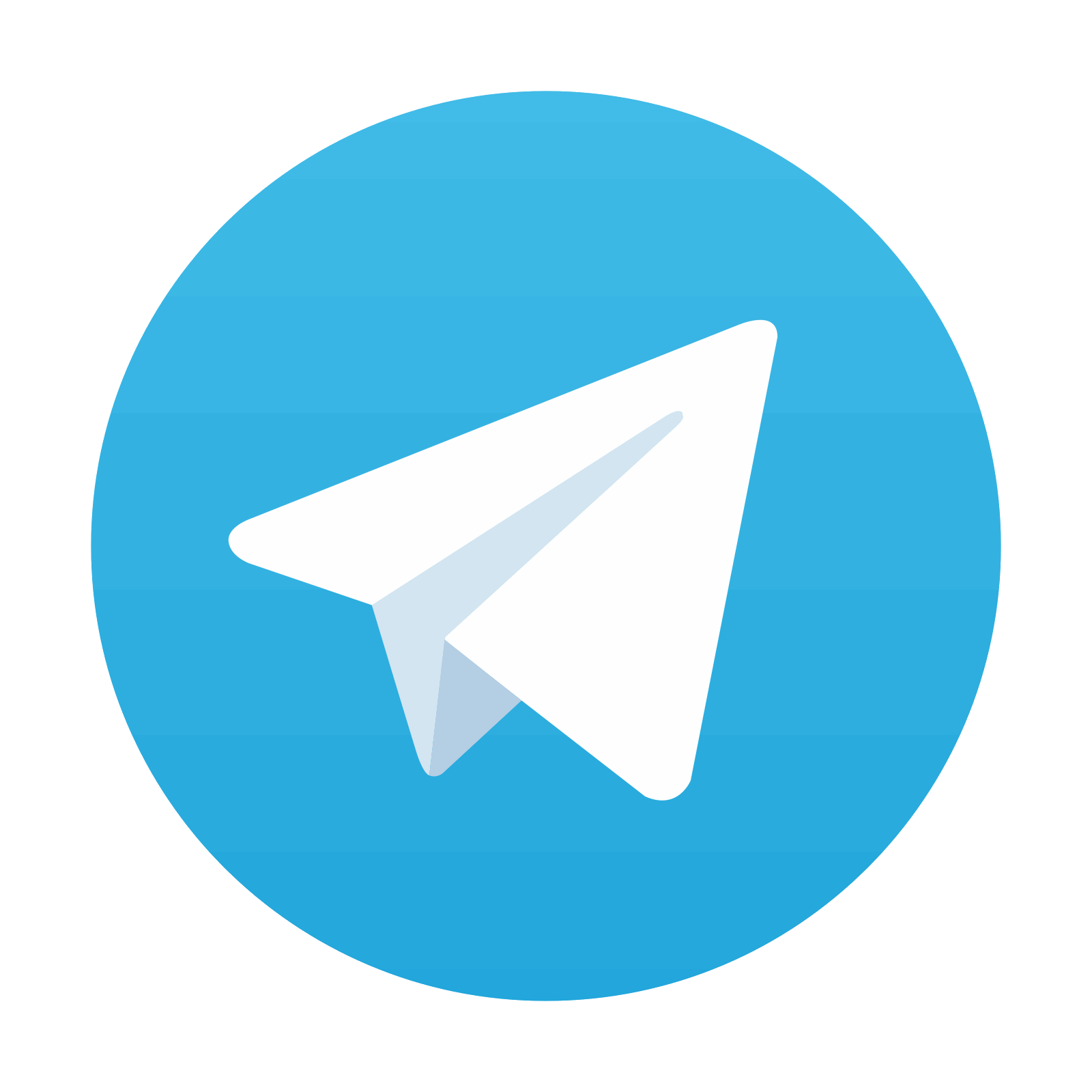
Stay updated, free articles. Join our Telegram channel

Full access? Get Clinical Tree
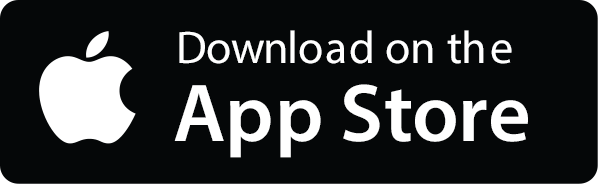
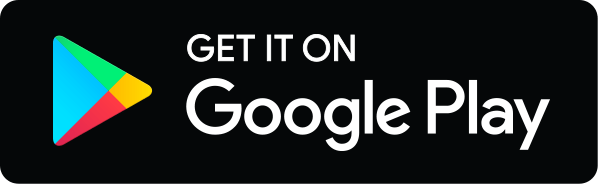