A. Anatomy—The kyphosis of the thoracic spine is produced and maintained by the wedge shape of the vertebral bodies (taller posteriorly than anteriorly). Normal kyphosis of the thoracic spine ranges from 20° to 50°. In contrast, the lordosis of the lumbar spine ranges from 40° to 70° (average 50°) and is principally created by the intervertebral disc configuration, taller anteriorly than posteriorly. Unique to the thoracic spine is its anatomic continuity with the rib cage and sternum; providing significant added stiffness and thus spinal cord protection. This stiffness contrasts with the mobile lumbar spine, and thus produces a transition zone at the thoracolumbar junction (T10–L2). This zone is marked with the loss of ribs, and a transition from a small thoracic spinal canal diameter to a larger lumbar canal diameter. Concurrently, the facet joint orientation transitions from coronal (thoracic) to sagittal (lumbar). This portion of the spine is distinctly “straight” from T10–T11 through L1 to L2.
Of all fractures, 6% involve the spine and 90% involve the thoracic and lumbar regions. Most occur in the T10-L2 transitional area with 40% of these having a spinal cord injury. In the presence of high-energy mechanisms, there is a 6:1 ratio of complete/incomplete neurologic injuries.
The spinal cord generally terminates around the L1 to L2 interspace; thus in addition to it being the structural transition area, the thoracolumbar spine is also a neurological transition area. Injuries at the conus medullaris and the cauda equina often have a more favorable prognosis than the more rostral spinal cord areas due to the presence of spinal nerves in addition to the terminal spinal cord. Spinal nerves are generally more resilient with improved capacity for recovery compared to the spinal cord. Due to this highly variable mix of spinal cord and spinal nerves, minimal correlation has been demonstrated between the degree of neurologic injury and the degree of canal compromise.
B. Epidemiology—Most common site of vertebral fractures (T10–L2—50% all spinal fractures).
1. Age—bimodal—most common <30 years old and geriatric
2. Motor vehicle accidents (young) and falls (elderly)
3. Gunshot wounds are increasing in frequency (Fig. 28-1)
4. Gender—Males > Females
5. Noncontiguous injuries—5-15%
6. Other injuries—pulmonary injuries (20%), peritoneal and retroperitoneal bleeding (10%—liver/spleen)
C. Mechanisms
1. Axial compression—Axial loading produces compressive loading to the vertebral bodies. With sufficient loads, failure occurs initially at the end plates (end-plate impaction fractures—perhaps due to the intervertebral disc driven through the end plates). The common vertebral body compression fractures (wedge fractures—Figs. 28-2 and 28-3) occur anteriorly with relative sparing of the middle and posterior body portions. A transition to burst fractures (Figs. 28-3 and 28-4) occurs with further axial and, subsequent, flexion loading. A pincer fracture (Fig. 28-5) is a unique vertebral body fracture characterized by the same axial loading mechanism; however, disc implosion through the vertebral body creates a coronal splint with separate anterior and posterior fragments. The disc below and above the involved vertebrae is in contact. This combined disc and vertebral body injury creates poorer healing.
2. Flexion—Tensile forces are created posteriorly while compressive forces act at the vertebral body. When there is a loss of greater than 50% of the vertebral body height, the posterior ligamentous integrity needs to be carefully evaluated. With an intact posterior osteoligamentous complex, this injury pattern is deemed mechanically “stable.” Failure to diagnose and treat posterior ligamentous injuries can result in instability with angular deformity and potential neurologic injury. MRI is the study of choice to directly assess the integrity of these structures (T2 and STIR images).
FIGURE 28-1 Sagittal computed tomography (CT) image demonstrating a missile (GSW) lodged in the right T3–T4 spinal canal. The patient had a Brown Sequard syndrome and the foreign body was removed via laminectomy.
FIGURE 28-2 Vertebral compression fracture.
FIGURE 28-3 Midsagittal Short Tau Inverted Recovery (STIR)-weighted magnetic resonance image demonstrating multiple acute and chronic osteoporotic compression and burst fractures in a caucasian obese 70-year-old female on steroids for chronic lung disease. A chronic T3 burst, acute T4 compression (mild), acute T5 burst, and acute T6 compression fracture are noted with edema (increased signal intensity) denoting acute fractures.
FIGURE 28-4 Vertebral burst fracture.
FIGURE 28-5 Burst Fracture with incomplete traumatic cauda equina syndrome, ventral and dorsal traumatic durotomies treated with posterior open reduction, decompression, and fusion with durotomy repair. MRI (A) and CT (B) demonstrate a burst fracture with pincer morphology with retropulsion, severe canal and cauda compromise, ligamentous injury, and lamina fracture. Operative photos demonstrate intradural fragments (C, D), which after dural repair were reduced via tamping fragments anteriorly away from the neural elements. Post-reduction CT is seen in Figure. 28-12B with adequate reduction and no need for anterior decompression with strut grafting.
3. Lateral compression—Lateral compression forces create lateral vertebral body fractures with or without contralateral or posterior ligamentous disruption. These are best seen on anteroposterior (AP) X-rays. Unrecognized injuries can lead to subacute deformity, pain syndromes, and neurologic deterioration.
4. Flexion-rotation—Flexion-rotation forces usually include an anterior bony injury with an increased probability of posterior ligamentous and facet capsule failure. The resultant anterior and posterior column involvement typically makes these unstable injuries. Pure dislocation at the thoracolumbar level is uncommon due to facet orientation; when it does occur, it is usually associated with a significant spinal cord injury.
5. Flexion-distraction (FDI)—Also known as “Chance Fractures” (Figs. 28-6 and 28-7) or “seat belt injuries” are classically seen in patients wearing only a lap belt during a motor vehicle collision. The generally accepted mechanism involves a flexion injury with the axis of rotation anterior to the spinal column creating posterior to anterior tensile (distractive) forces across the entire spinal segment with resultant posterior ligamentous or posterior bony avulsion injuries. Forces then continue anteriorly to create either injury to the disc, vertebral body, or both. Vertebral body injury usually exits into the adjacent disc spaces across the end plate (“osseoligamentous” chance fracture) or exits the anterior cortex of the body, creating a pure “bony” chance fracture. Isolated bony chance fractures, most common at L1 to L3, generally heal with proper immobilization while osseoligamentous injuries, most common at the thoracolumbar junction, heal significantly poorer. In addition, secondary axial loading thought to be related to deceleration forces with instantaneous displacement of the axis of rotation can produce vertebral body fractures on a continuum from compression to burst fractures as noted by Court Brown and Gertzbein who devised a flexion-distraction classification based on anterior or posterior fracture involving disk and soft tissue elements and/or bony elements.
FIGURE 28-6 Flexion-distraction (Chance) injuries. A. One-level injury with the injury through bone. B. One-level injury with the injury through soft tissues.
6. Extension—Rare shearing injuries (“lumbarjack” injuries): extension and distraction (ED) have opposite injury patterns and mechanisms compared to flexion injuries. Anterior tensile failure with posterior compressive forces leads to posterior element fractures including laminae, facets, and/or spinous processes (Fig. 28-8). Retrolisthesis of cephalad on caudad vertebral body and anterior disc injury (due to tension) can lead to angular deformities. One must be aware of these injury patterns and associated fractures in patients with stiff spines such as those with Diffuse Idiopathic Skeletal Hyperostosis (DISH) or Ankylosing Spondylitis (AS).
II. Evaluation
A. Associated Injuries—50% of patients with thoracolumbar fractures have non-spinal injuries. Forty-five percentage of flexion-distraction injuries have intraabdominal injuries (i.e., splenic or liver lacerations). Noncontiguous injuries occur 20% of the time. Head injuries and extremity injuries are also common in falls from a height.
B. Overview—The primary trauma survey should be conducted with the “ABCs” (airway, breathing, circulation) and Advanced Trauma Life Support (ATLS) with identification of life-threatening injuries, oxygen, and hypotension management. Cervical collar placement and full spine immobilization precedes the secondary survey.
1. History—Mechanism with likelihood of associated injuries may be determined. Witnesses are helpful as are full details of motor vehicle accidents (speed, location of impact, restraint use). Evaluation of neurologic symptoms may provide insight into spinal cord or neural element pathology.
2. Physical examination—“Log rolling” with full spine visualization and palpation for tenderness, spinal process step-offs or soft tissue defects, and crepitus. Concomitant rectal examination should be performed with notice of tone, perianal sensation, evaluation of anal wink and bulbocavernosus reflex. Fifty percentage of spinal injuries are missed on initial evaluation. Serial neurologic exams include motor and sensory testing, and reflex examination. Careful evaluation of the trauma patient in shock is necessary to determine the etiology including consideration of spinal shock as causative. Abdominal tenderness and ecchymosis should raise suspicion for a “seatbelt” injury.
FIGURE 28-7 Parasagittal CT (A) image demonstrates a flexion-distraction injury with superior facet fracture with dislocation. Midsagittal MRI (B) demonstrates severe spinal cord compression with edema. This patient had a T9 ASIA B injury (see Table 25-3) with only sacral sparing. Treatment was via posterior open reduction with compression instrumentation (C) with iliac crest autograft.
C. Clinical Evaluation and Steroid Use
1. Spinal shock and complete versus incomplete injury—Spinal shock is a physiologic spinal cord dysfunction occurring below the level of anatomic cord injury with flaccid paralysis, areflexia, and absent sensory modalities resolving in 99% of cases by 48 hours. Re-examination with return of the bulbocavernosus reflex hails the end of spinal shock. Total absence of motor (Frankel system grades 0 to 5) and sensory function below the anatomic injury level denotes complete neurologic injury whereas incomplete injuries maintain residual cord or root function below the injury level. The American Spinal Injury Association (ASIA) has devised the standards for describing spinal cord injuries based on motor and sensory levels in addition to the presence or absence of sacral sparing.
FIGURE 28-8 Midsagittal CT (A) and postoperative lateral radiograph (B) demonstrate an unstable T9–T10 extension-distraction fracture in a patient with Diffuse Idiopathic Skeletal Hyperostosis (DISH). MRI did not show epidural hematoma. Positioning on a Wilson frame on a Jackson table facilitated reduction in flexion followed by posterior compression instrumentation.
2. Incomplete spinal cord injury patterns (see Chapter 25)
D. Steroids—(see Chapter 25)
E. Imaging—After clinical evaluation, multiple modalities can be utilized to effectively visualize thoracolumbar fractures. One must remember to image the entire spinal column once spinal fractures are found in order to avoid missing noncontiguous fractures (typically CT).
1. Radiographs—AP and lateral views combined with swimmer’s views that are occasionally necessary to visualize the cervicothoracic junction tend to define vertebral anatomy. The lateral view allows vertebral, facet, spinous process, and intervertebral foramina alignment and assessment. Loss of vertebral body height (>50%) and cortical margins also denote compressive spinal injury patterns. Particular care should be noted to the posterior vertebral line or angle as this can differentiate burst (from compression) fractures with spinal canal compromise. AP views may demonstrate lateral compression fractures, spinous process and pedicular alignment or widening allowing diagnosis of posterior element injury and secondary evaluation of spinal posterior ligamentous complex (PLC) instability (>30° kyphosis). Lateral radiographs also allow Cobb measurements of sagittal kyphotic deformities, translation anteriorly or posteriorly (>2.5 mm). Close attention to the endplates on the AP view will also identify subtle injuries missed on lateral views. Plain films are becoming increasingly less popular due to low sensitivities and the utility of screening CT scans for chest, abdomen, and pelvis. However, stable fractures treated nonoperatively should have standing lateral radiographs in an orthosis to ensure a good baseline radiograph as well as no collapse or kyphosis once mobilized.
2. Computed tomography (CT)—CT allows ideal characterization of bony fracture patterns with sagittal, coronal, and 3-D reconstructions. In polytrauma evaluations, screening CT scans serve well for both visceral and bony injury as Inaba and coworkers demonstrated superior sensitivity and interobserver variability of reformatted CT scans compared with plain radiographs for localizing, classifying, and delineating the thoracolumbar spinal injuries (25% injuries are missed on radiograph alone with gross underestimation of burst spinal canal compromise). Abdominal CT is recommended when a FDI is suspected to evaluate for intraabdominal injury.
3. Magnetic resonance imaging (MRI)—MRI is the modality of choice for visualization of disc herniation, epidural hematoma, ligament injury and SCI. Injury to ligamentous and neurologic structures can be characterized and classified regarding presence of disruption, edema, hematoma, traumatic disc herniation, and presence of cysts or syrinx. Discontinuity of the “black stripe” (classically the ligamentum flavum) with focal stripe of T2 fluid extending superficially indicates instability with tension band disruption. Spinal cord edema or hemorrhage can easily be seen on T2 or T1 weighted images. Use of MRI is controversial in GSW patients.
F. Spinal Stability
1. Injury classifications generally relate to the concept of spinal stability. White and Panjabi define clinical instability as “loss of the ability of the spine under physiologic loads to maintain relationships between vertebrae in such a way that there is neither damage nor subsequent irritation to the spinal cord or nerve roots and no development of incapacitating deformity or pain”.
2. Holdsworth predominately identified the posterior ligamentous complex as the key structure(s) to thoracolumbar spinal stability. He classified fracture-dislocations and shear injuries as unstable, whereas all other fracture patterns were deemed stable. The two-column theory arose from belief that the vertebral body and disc were more important as a weightbearing column with a tension band column posteriorly (facet capsules and interspinous ligaments).
3. James et al. confirmed biomechanically the importance of the posterior (instead of middle) column in 1994 emphasizing nonoperative treatment in neurologically intact individuals without posterior column involvement. In vitro studies demonstrated that in addition to posterior ligamentous complex (PLC) disruption, a rotational torque or sectioning of the posterior aspect of the anterior column (posterior annulus) was needed to produce instability.
4. Denis proposed a three-column theory identifying a middle column injury to deem an injury unstable and needing operative intervention. The middle column is an osseoligamentous segment including the posterior half of the vertebral body, nucleus pulposus, annulus, and posterior longitudinal ligament (PLL).
5. Denis classified thoracolumbar spine fractures into four categories: (a) compression fractures, (b) burst fractures, (c) flexion-distraction injuries, and (d) fracture-dislocations.
6. Mechanical instability is defined as the presence of injuries to two or more of the three columns, which allows abnormal motion across the injured spinal segment. However, burst fractures involving the anterior and middle column can have an intact PLC, maintaining sufficient ligamentous integrity to allow nonoperative treatment. This demonstrates the weakness of the three-column approach.
7. Others such as Panjabi and White maintain a vague yet pragmatic method defining clinical stability present when under normal physiologic loading the spinal column can maintain its normal pattern without displacement creating any additional neurologic deficit, incapacitating pain or deformity.
III. Injury Classifications
A. Holdsworth Classification—This early classification system conceptualizes the spine as composed of two columns (anterior/posterior). Holdsworth believed that the PLC ultimately determined stability at each segment. All posterior column injuries were hence unstable.
B. Denis Classification—The three-column classification scheme involves structures of the anterior (ALL, anterior ½ body/disk/annulus), middle (posterior ½ body/disk/annulus, PLL), and posterior (all posterior bony and ligamentous structures including pedicles/laminae/facets/spinous process/ligamentum flavum/spinous ligaments) columns. Injuries were described as minor (15% to 20% of fractures involving the spinous and transverse processes, pars interarticularis, and facet articulations) and major (compression fractures, burst fractures, flexion-distraction injuries and fracture-dislocations). Definitions of stability and instability are described above. Middle column injury essentially defined stability. Criticism has evolved due to lack of insight in determining stable/unstable injuries especially in light of modern biomechanical studies questioning middle column importance, advances in imaging modalities, and inability to direct fracture management.
C. McAfee Classification—McAfee’s classification (wedge-compression, stable and unstable burst, Chance, flexion-distraction, and translational) arose in response to criticisms of Denis’ classification by utilizing CT to describe the mode of failure of the middle column, and the classification emphasizes various injuries as stable/unstable with emphasis on the importance of the PLC. The “stable burst fracture” was coined by McAfee and involves the anterior and middle columns with compression fractures but an intact PLC whereas unstable burst fractures involve disruption of the PLC. Chance fractures consist of a horizontal vertebral avulsion injury with the axis of rotation anterior to the ALL. The other two modes include flexion-distraction and translational injuries.
D. AO/ASIF and OTA Classification (Magerl and coworkers)—The AO/ASIF classification is based on the three primary forces applied to the spine. Type A injuries are those caused by compressive loads, Type B injuries are distractive injuries, and Type C injuries are rotational and multidirectional. Each fracture type is divided into three subtypes depending on the severity of load applied and structure(s) compromised (bone vs. soft tissue). The classification provides rationale for determining treatment and prognosis but is limited due to its complex scheme yielding low interobserver reliability.
E. McCormack “Load Sharing”—Assesses vertebral body comminution, fragment displacement, and kyphosis to predict which injuries can be treated with nonoperative management, short-segment transpedicular constructs, or additional anterior column support. Total points greater than 6 require additional anterior column support. Biomechanical and clinical reports have validated its use.
F. Thoracolumbar Injury Classification and Severity Score (TLICS)—TLICS was developed by Vaccaro and coworkers as a practical comprehensive system to aid in decision making regarding operative versus nonoperative care in unstable injury patterns. TLICS is based on three injury characteristics: (1) injury morphology on radiographic appearance, (2) integrity of the posterior ligamentous complex, and (3) neurologic status of the patient. Each characteristic is assigned points and if the sum totals less than 3, nonoperative treatment is recommended. If the sum is greater than 5, surgical intervention is recommended. If the score is 4, injuries might be handled operatively or nonoperatively. In addition, TLICS guides the surgical approach to injuries as seen in Table 28-1. General principles include (a) anterior procedure for incomplete neurologic injuries in which neural elements are compressed from anterior spinal elements, (b) posterior procedure for PLC disruption, and (c) combined approaches for combined incomplete neurologic injury and PLC disruption.
1. Injury morphology—Fracture patterns are similar to the AO classification and described as compression, translation/rotation, and distraction.
From Vaccaro AR, Lehman RA Jr, Hurlbert RJ, et al. A new classification of thoracolumbar injuries: the importance of injury morphology, the integrity of the posterior ligamentous complex, and neurologic status. Spine. 2005;30:2325–2333, with permission.
• Compression (1 point, 2 points for burst)—Compression fractures include axial, flexion, and lateral compression or burst fractures secondary to vertebral body failure under axial loading.
• Rotation/translation (3 points)—These fracture patterns include translation/rotation compression or burst fractures, and unilateral or bilateral facet dislocations with or without compression or burst characteristics. These patterns generally occur under torsional and shearing forces.
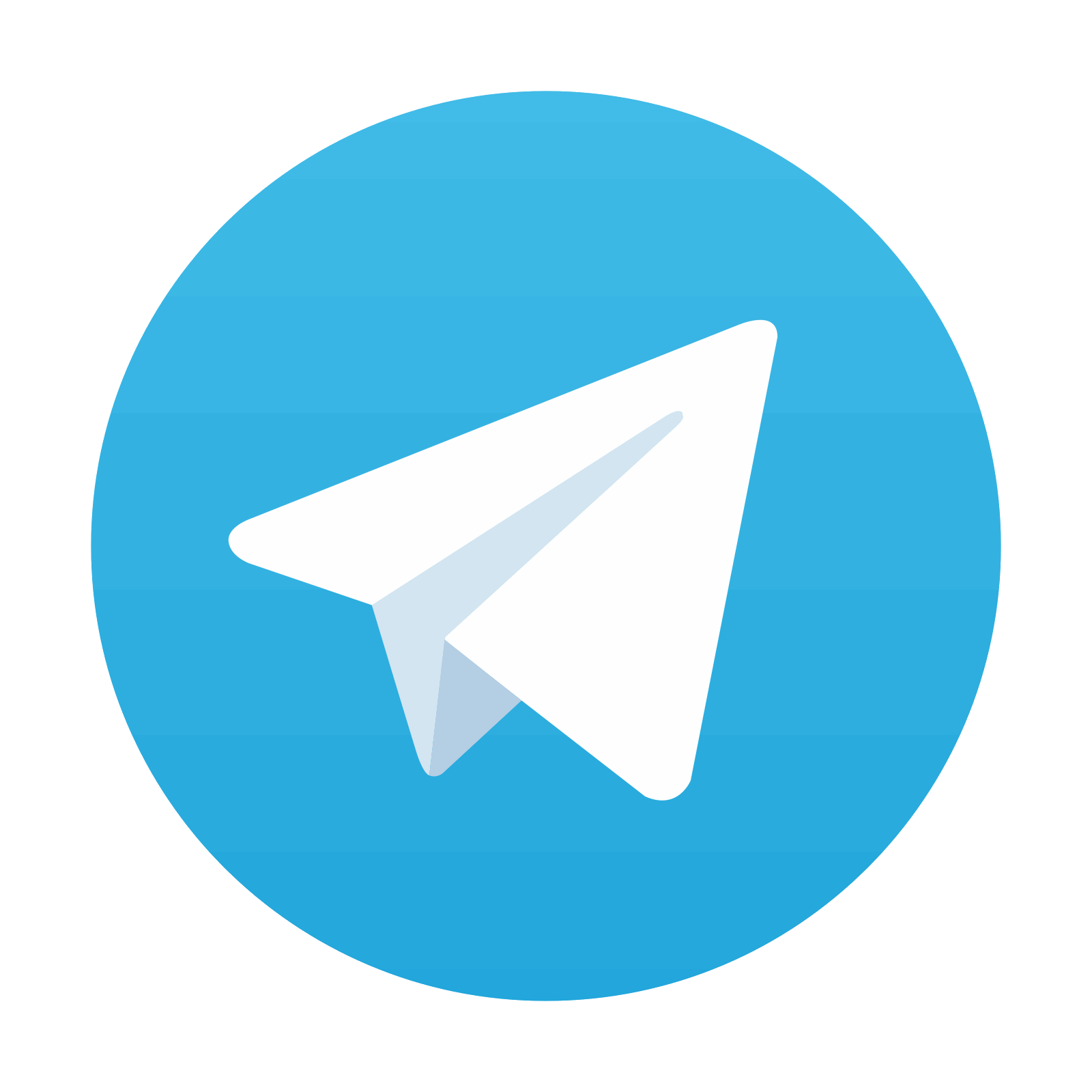
Stay updated, free articles. Join our Telegram channel

Full access? Get Clinical Tree
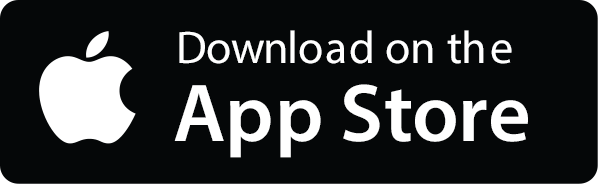
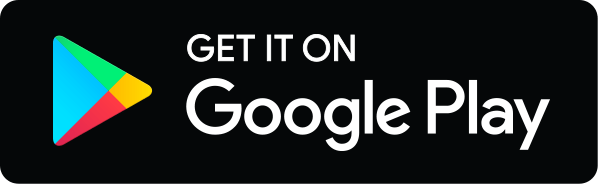