I. Introduction—Few conditions are as devastating as spinal cord injury (SCI). Patients with SCI usually face several medical problems related to their initial immobilization, prolonged rehabilitation periods, significant readjustment in their lifestyle, and potential complications in the chronic stages of their disease. The earliest description of SCI as an entity was reported by the Egyptians in 1700 BC. Even at that time, patients were described with injuries so severe that they were considered to harbor an “ailment not to be treated.” At the beginning of the century, the mortality of SCI was around 90%. At the end of World War I, special care of the urinary tract, respiratory system, and skin of patients with SCI led to a significant improvement of their survival. Despite this improved survival, patients were left with significant disabilities, and their recovery did not seem to benefit from any kind of therapy. Early studies on dogs by Allen showed that the spinal cord’s secondary response to injury was responsible for a progression of the tissue damage created by the initial insult and that in certain cases, active early treatment such as decompression, could improve the neurological recovery. Goals of SCI treatment in recent decades have therefore been focused toward early aggressive treatment and prevention of secondary injury mechanisms.
II. Epidemiology—The incidence and prevalence of SCI in North America have been studied in detail over the past two decades (Table 25-1). The exact figures are difficult to determine because of inherent methodological limitations. For example, multiple admissions of a single patient may overestimate the actual rate while misclassification of SCI when the patient presents with multiple injuries may underestimate it. The incidence of SCI, defined as the number of new cases in a given population in a discrete geographical region within a specific time frame is estimated to be around 40 per million per year. The prevalence of SCI, that is, the number of individuals with SCI within a specific population at a specific point in time, averages 70 per 100,000 or approximately 200,000 to 250,000 total patients in the United States.
As in most traumatic injuries, the incidence of SCI is significantly higher in males than in females (75% of cases occurring in males). The average age for both males and females at the time of injury is around 35 years old. Peak age of incidence is 20 to 24 for males compared with 25 to 29 for females. The incidence among males increases dramatically after age 15, declines after age 30, and increases again steadily in the later decades. The initial peak for females is approximately 5 years later than it is for males.
The causes of acute SCI are varied but have remained relatively consistent over the past two decades (Table 25-2). Motor-vehicle accidents are still the most frequent etiology of SCI, accounting for approximately 50% of all cases. Falls, either accidental or from suicide attempts, also account for a significant proportion of SCI cases at approximately 20%. Violent assaults in urban areas have increased dramatically over the past 15 years and are now estimated to be the etiological factors in 15% to 20% of SCI. The vast majority of penetrating injuries of the spinal cord involve firearms. Acute SCI occurs in the context of sports and recreational activities in approximately 10% to 20% of cases. Diving injuries account for two-thirds of these injuries. Because of widespread awareness campaigns and media coverage, sports-related SCI has been steadily decreasing since 1975.
In addition to the significant physical and psychological impact of SCI, the financial burden related to hospitalization, rehabilitation, and environment modification of victims is tremendous. The lifetime costs for SCI range from $600,000 to $1 million, depending on its cause. Based on the incidence of SCI, total direct costs for all causes of SCI in the United States are as high as $8 billion.
III. Anatomy of the Spinal Cord
A. Meningeal Layers—The adult spinal cord lies in the vertebral canal and extends from the foramen magnum, where it is continuous with the medulla, to the first lumbar vertebra (Fig. 25-1). Three meningeal layers cover it. The spinal dura, the outermost layer, is a tubular continuation of the meningeal layer of the cranial dura. In contrast to its cranial counterpart, the spinal dura is separated from the inner periosteum of adjacent vertebrae by the epidural space. This space contains a variable amount of loose areolar tissue (epidural fat) and the internal vertebral venous plexus. The pia mater is adherent to the surface of the spinal cord and forms a triangular-shaped condensations on each side, at regular interval, which attach the cord to the inner surface of the dural tube. These structures are called denticulate ligaments. The arachnoid matter is located between the dura and the pia mater, and extends to the proximal roots of spinal nerves. The space between the pia and arachnoid is termed subarachnoid space. It is in this space that the cerebrospinal fluid circulates. The spinal cord has two enlargements situated at the cervical and lumbar areas, which are associated with the spinal roots innervating the upper and lower limbs. The termination of the spinal cord has a conical shape and is termed conus medullaris. The conus is attached to a condensation of pia mater, the filum terminale. The filum extends caudally until it becomes invested by the end of the dural sac and forms the coccygeal ligament, which continues to the coccyx where it becomes continuous with the periosteum.
B. Blood Supply—The arterial blood supply to the spinal cord consists of descending branches of the vertebral arteries and multiple radicular arteries derived from segmental blood vessels. There are two posterior spinal arteries, each arising from its respective vertebral artery posteriorly. They descend on the posterior surface of the spinal cord, just medial to the dorsal roots. They receive a variable amount of supply from the segmental posterior radicular arteries along the way down, forming two plexiform channels. Together, they supply the posterior third of the spinal cord. Paired anterior spinal arteries unite caudally just after they branch off their respective vertebral artery and descend as a single vessel that enters the anterior median fissure of the spinal cord. Branches from anterior radicular arteries anastomose with the anterior spinal arteries at different levels in order to form one continuous vessel, although it may become discontinuous or very small at certain levels. The anterior spinal artery supplies the anterior two thirds of the spinal cord. The radicular arteries arise from segmental vessels such as the ascending cervical, deep cervical, intercostal, lumbar and sacral arteries. Once it enters the intervertebral foramen, a radicular artery becomes an anterior radicular or posterior radicular artery or divides into both branches. Radicular arteries usually arise from the left side in the thoracic and lumbar region, while both sides supply the cervical cord equally. The thoracic cord has the greatest distance between each of its supplying radicular arteries, rendering it more prone to ischemia in the event of an occlusion of one of these vessels. The artery of Adamkiewicz is an anterior radicular artery found in the thoracolumbar area and is appreciably larger than all others. It usually arises between T8 and L2, mostly from the left.
FIGURE 25-1 Human spinal cord in vertebral column seen in mid-sagittal section. Note the position of the spinal cord segments with reference to the bodies and spinous processes of the vertebrae. Locations of entrance and exit of spinal roots are indicated.
C. Segmental Anatomy—An understanding of the segmental anatomy of the spinal cord is essential in order to interpret the different neurological syndromes that patients with SCI present with. A complete anatomical description is beyond the scope of this chapter, and only the relevant structures and pathways will be described. Figure 25-2 illustrates a transverse section of the spinal cord. The gray matter consists of a symmetrical butterfly-shaped structure in the middle of the cord. The dorsal horn is in the posterolateral position and contains mainly sensory neurons that receive afferents from sensory fibers whose cell bodies are located in the ipsilateral dorsal root ganglion. The anterior horn contains motoneurons that send axons to respective segmental skeletal muscle fibers and to intrafusal muscle fibers via the ventral root. In the thoracic cord, there is a lateral horn, which extends from C8 to L2. It consists of preganglionic sympathetic neurons. The sympathetic fibers from these cell bodies exit with the anterior root at the T1 to L2 levels and give white rami to sympathetic chain nuclei located on each side of the spine. There they synapse with postganglionic sympathetic neurons and the sympathetic fibers travel with blood vessels to the viscera and vascular beds they innervate.
1. Sensory pathways—Two main pathways carry sensation. The discriminative touch pathway carries sensations for two-point discrimination, proprioception, and vibration via large fibers in the dorsal root. Most of the fibers enter the cord and ascend immediately in the ipsilateral dorsal columns located in the posterior midline of the cord. They synapse with second-order neurons in the brainstem and then cross the midline, course cranially, and synapse in the contra-lateral thalamus. The third-order neurons in the thalamus send their projections to the somatosensory cortex. The pain and temperature pathway carries sensations of nociception, heat, cold and simple (primitive) touch via smaller fibers in the dorsal root. The fibers enter the cord and synapse with second-order neurons located in the dorsal horn. The axons of the second order neurons, after ascending or descending one or two segments, cross the midline within the spinal cord. The crossing fibers form the anterior commissure, ventral to the central canal, and reach the spinothalamic tract located in the ventro-lateral aspect of the cord. They then ascend to synapse with third-order neurons in the contralateral thalamus. The third-order neurons in the thalamus send their projections to the somatosensory cortex. Since the first pathway crosses the midline above the spinal cord and the second within the cord, dissociate sensory loss may occur in partial cord syndromes where only one side of the cord is affected (see later section).
FIGURE 25-2 Segmental anatomy of the spinal cord. The white matter is organized in three columns (dorsal, lateral and ventral) running in the log axis of the cord. The dorsal columns are involved in touch, proprioception and vibration sensation and the spinothalmic tracts, located ventrolaterally are involved in pain and temperature sensation. The corticospinal tracts, located laterally, carry the axons of upper motor neurons. The central gray matter is divided into the dorsal horn, comprising sensory cells, and the ventral horn, which contains motoneurons. The intermediate zone, between the dorsal and the ventral horns contains the preganglionic neurons of the sympathetic (thoracic cord) and sacral parasympathetic systems. The main components of the central gray matter and the surrounding white matter are illustrated.
2. Motor pathway—The motor pathway originates in the cerebral motor cortex, as well as from deeper extra pyramidal nuclei. The bulk of the fibers travel caudally in the internal capsule and enter the brainstem, where they form the corticospinal tracts located ventrally. In the medulla, the fibers form the pyramids, which are located ventrally. In the lower medulla, approximately 80% of the fibers cross the midline just before entering the spinal cord. The corticospinal tract in the spinal cord is located in the mid-lateral position and its fibers synapse with motoneurons of the ventral horn at appropriate levels.
3. Lamination of fibers—Fibers in the different tracts of the spinal cord are organized in a specific pattern. Figure 25-3 illustrates the location of the axons supplying the arms and legs in the dorsal columns and spinothalamic tracts. Although this lamination of fibers helps understanding the clinical presentation of certain incomplete syndromes in SCI, it is now clear that such a somatotopic organization may not exist in the corticospinal tract (see later section).
4. Dermatomes and myotomes—Each segment of the spinal cord innervates a certain sensory area of the body called dermatome and specific groups of skeletal muscles called myotome. Figure 25-4 illustrates the major myotomes and all dermatomes of the human body.
IV. Classification of SCI—Consensus in classification of SCI based on neurological examination is essential to determine prognosis as well as for follow-up examinations and longitudinal studies. The most recent classification, The International Standards for Neurological and Functional Classification of Spinal Cord Injury was developed in 1992 by the American Spinal Injury Association (ASIA) and the International Medical Society of Paraplegia (IMSOP). This system is now widely used internationally by all teams involved in the treatment of SCI (Table 25-3 and Fig. 25-4).
A. ASIA/IMSOP Impairment Scale—The ASIA/IMSOP impairment scale shown in Table 25-3 consists of five grades of impairment. Grade A is defined as complete injury, while Grades B, C and D represent varying degrees of incomplete injuries, and Grade E denotes a normal neurological examination. Complete SCI is defined as an absence of motor and sensory function in the lowest sacral segments, S4 and S5. As can be seen in Figure 25-4, this system requires the examination of 10 key muscle groups on the left and right as well as 28 dermatomes on the left and 28 on the right. The scoring system for the motor function uses the MRC scale from 0 to 5 (see Fig. 25-4), with a maximum possible score of 100. The sensory examination is based on a scale of 0 to 2 for pinprick with a maximum possible score of 112.
In order to determine if a lesion is complete, the sensory and motor functions of the lowest sacral segments S4 and S5 have to be tested. Sensory function is assessed at the perianal region and at the musculocutaneous junction while deep anal sensation and voluntary contraction of the external anal sphincter must be examined during digital rectal examination. Determination of the completeness of a lesion needs to be made early because the prognosis for recovery is much better in incomplete injuries.
The neurological level of a SCI is determined by both the sensory and the motor exam, on both sides of the body. The sensory and the motor levels are defined as the most caudal (lowest) segment with normal sensory or motor function, respectively. The 1992 classification defined normal motor function as Grade 4 or 5. This statement led to some confusion in interpreting the neurological level in certain patients, especially those with incomplete injuries. A revision to the classification was thus made in 1996 where the motor level is defined as the lowest key muscle that has a grade of at least 3. Since the motor and sensory examination may differ on each side of the body, there may be up to four different levels identified. If dermatomes and myotomes remain partially innervated below an identified level, they are classified as a zone of partial preservation.
FIGURE 25-3 Segmental anatomy of the spinal cord illustrating the lamination of fibers in the main white matter tracts. The dorsal columns and the spinothalamic tracts are somatotopically organized.
B. Spinal Shock—Spinal shock is defined as the loss of somatic motor, sensory, and sympathetic autonomic function after SCI. The loss of somatic motor function results in flaccid paralysis and areflexia. Sensory loss may be complete to all modalities. The loss of autonomic function, mainly sympathetic innervation, can manifest in hypotension, bradycardia as well as skin warmth and hyperemia. The severity and duration of spinal shock varies, but it correlates with the severity of the SCI and the level of the injury. Thus, is it usually most severe in complete cervical and upper thoracic cord injuries, less severe in incomplete injuries, and minimal in lumbar injuries. In its most severe form, the loss of sympathetic tone (to the heart and vasculature) results in sustained hypotension and bradycardia which leads to neurogenic shock. The etiology of spinal shock is unknown, although suspected mechanisms include imbalances in transmembrane ionic concentrations and permeabilities secondary to local tissue trauma, such as an increase in extracellular potassium concentration resulting in inhibition of local neuronal excitability. The presence of spinal shock can cause a significant amount of confusion in the initial neurological assessment of the patient. It is therefore recommended to assume that its effect on the somatic motor and sensory exam have resolved by 1 hour after the initial injury and that the autonomic and reflex dysfunctions may persists for days to weeks.
Acute SCI has been associated with pulmonary edema in a number of cases. Its mechanism is uncertain but it is suspected that massive sympathetic discharge at the time of injury results in a catecholamine surge. The rapid rise in catecholamines leads to acute left ventricular failure from a sudden increase in afterload as well as fluid shifts from the periphery to the pulmonary vasculature.
FIGURE 25-4 ASIA/IMSOP classification of SCI. The diagram illustrates the principal information about motor, sensory International Medical Society for Paraplegia: International standards for neurological and functional classification of SCI, and sphincter function required for accurate classification and scoring of acute spinal cord injuries. The 10 key muscle groups to be tested are shown on the left along with the MRC grading system, and the 28 dermatomes to be tested on each side for the sensory examination are shown on the right. (Courtesy: American Spinal Injury Association, revised 1996, Chicago, 1996, The Association and The Society.)
V. Incomplete Spinal Cord Syndromes—When there is preservation of sensation or motor function in the lowest sacral segments S4 and S5, a SCI is said to be incomplete. It therefore falls in the B, C, or D category of the ASIA/IMSOP classification. Patients with incomplete SCI often present with specific patterns of neurological deficits. The incomplete syndromes are generally classified according to the anatomic location of the injury in the transverse section of the spinal cord. This classification is useful in understanding the pathophysiology and mechanisms involved in specific types of injury, which can help direct treatment in the early phase. Since the potential for neurological recovery differs for different syndromes, this classification also helps in the assessment of prognosis upon presentation.
A. Cervicomedullary Syndrome—This syndrome occurs in cervical spinal injury, where the high cervical cord and medulla are involved, although it can extend caudally as far as C4 and rostrally to the pons. It is caused either by direct injury to the cord or medulla or concomitant disruption of the vertebral arteries during cervical spine trauma. Other terms have been used for this presentation such as “bulbar-cervical dissociation.” In its most severe form, this syndrome results in respiratory arrest, hypotension, tetraplegia and anesthesia, usually below C4. Without immediate first-aid treatment, death occurs shortly after the initial injury from cardiopulmonary arrest. Improved on-site emergency airway management and cardiac resuscitation has led to an increase in patients presenting with this syndrome due to an increase in survivors. The mechanism of injury usually consists of traction on the high cervical cord and medulla caused by dislocation or anteroposterior compression from a displaced fracture or intervertebral disc rupture. The vertebral artery enters the transverse foramen of C6, ascends vertically through each vertebral foramen, exits at C2, and courses over the lateral mass of C1. Entrapment at any point along its way may occur during cervical spine injury. It is particularly susceptible in cases of fracture-dislocation and acute torsion of the upper cervical spine.
The clinical presentation of the cervicomedullary syndrome has two important characteristics.
1. Dejerine pattern of anesthesia—Patients can present with the classic Dejerine pattern of anesthesia of the face (anesthesia of the outer periphery of the face with sparing of the middle portions including the nasal alae to the vermilion border of the lips). This peculiar presentation occurs because the sensory fibers of the face course downward in the spinal trigeminal tract after entering the brainstem and synapse with the spinal trigeminal nucleus as low as C4. The fibers supplying the periphery of the face travel the furthest down while those supplying the center of the face synapse almost immediately after entering the brainstem, which explains why they are spared when the injury is at the level of the cervical cord. Anesthesia is present below the level of injury but there can be sparing of the collar area supplied by C2 to C4. This is thought to be due to the fact that the sensory fibers from these levels are carried via two different pathways; the classic lateral spinothalamic tract and the spinal trigeminal tract, which ascends caudally before crossing in the brainstem. The second tract may be spared from injury because of the difference in the level of crossing. A complete sensory exam, including the face, is thus essential in the assessment of all patients with cervical spinal injuries in order to recognize this pattern.
2. Cruciate paralysis of Bell—Cervicomedullary syndrome may also mimic central cord syndrome in producing more significant weakness in the arms than the legs. This form of weakness pattern is termed cruciate paralysis of Bell. The neuroanatomical explanation proposed to account for this syndrome was outlined in 1901 by Wallenberg. He suggested that the somatotopic organization of the corticospinal fibers in the pyramids resulted in different decussation locations for the leg fibers compared with the arm fibers. However, no neuroanatomic evidence has ever been presented to support this but the theory prevailed. It is now thought, based on several lines of evidence, that the reason why hand function is more affected is that the corticospinal tracts are more important for hand function. Detailed studies of the corticospinal tract organization confirm that a somatotopic organization is lacking. Marchi degeneration studies as well as modern neuroanatomic tracers have shown that the corticospinal fibers serving the upper and lower extremities are diffusely distributed within the pyramids and their decussation and within the corticospinal tract of the spinal cord. In his review of spinal cord syndromes, Schneider found that the pyramids are susceptible to compression by the odontoid process during fracture of the odontoid or atlantooccipital and atlantoaxial dislocations, or other lesions in the vicinity of this structure, such as tumors. Localized injury to the pyramids could explain the disproportionate hand weakness of cruciate paralysis of Bell. In a review of 14 patients with a clinical diagnosis of cruciate paralysis, Dickman and coworkers reported fractures of the upper cervical spine (C1 to C3) in eight patients. In each of the eight patients with fractures, the C2 vertebra was involved. Of the seven patients who had MRI studies performed, three demonstrated contusions or edema localized to the anterior or anterolateral segments of the cervicomedullary junction and superior cervical cord; this supports the concept that the injury must be mild to moderate, damaging motor function (subserved by the corticospinal tracts in the pyramids) yet preserving life.
B. Central Cord Syndrome—This syndrome was also described initially by Schneider. It is characterized by a disproportionately greater loss of motor power in the upper extremities than in the lower extremities, bladder dysfunction usually in the form of urinary retention, and a varying degree of sensory loss below the lesion. Pathological reports of patients presenting with this condition who succumbed after the initial injury revealed that the spinal column and ligaments were intact and that the cord often had a central area of hemorrhage. Taylor has shown that it is possible to have compression of the spinal cord without damage to the vertebral column. He performed cervical myelography on cadavers with their necks in different positions and demonstrated that in the forced extension position, there were a series of indentations on the posterior surface of the spinal column at the level of the interlaminar spaces. These indentations appeared to be caused by inward bulging of the ligamentum flavum, resulting in a narrowing of the spinal canal by as much as 30%. The narrowing was even more marked when osteophytic protrusion in the posterior vertebral bodies were present. It is thus believed that the major mechanism of injury involved in central cord syndrome consists of hyperextension of the cervical spine resulting in acute anteroposterior compression of the spinal cord. In his analysis of spinal cord segments of patients with central cord syndrome, Holmes found a significant amount of edema in the tissue located at the level of the injury.
Schneider postulated that the syndrome is caused by hematomyelia and surrounding edema in the central cord and suggested that the discrepancies in arm and leg weakness is due to the lamination of fibers in the corticospinal tracts. He also postulated that the involvement of anterior horn cells in the cervical cord could account for the more pronounced weakness of the arms. As explained earlier, modern studies have failed to show a somatotopic organization of the corticospinal tracts in the spinal cord. An alternative explanation may be that the function of the corticospinal tract
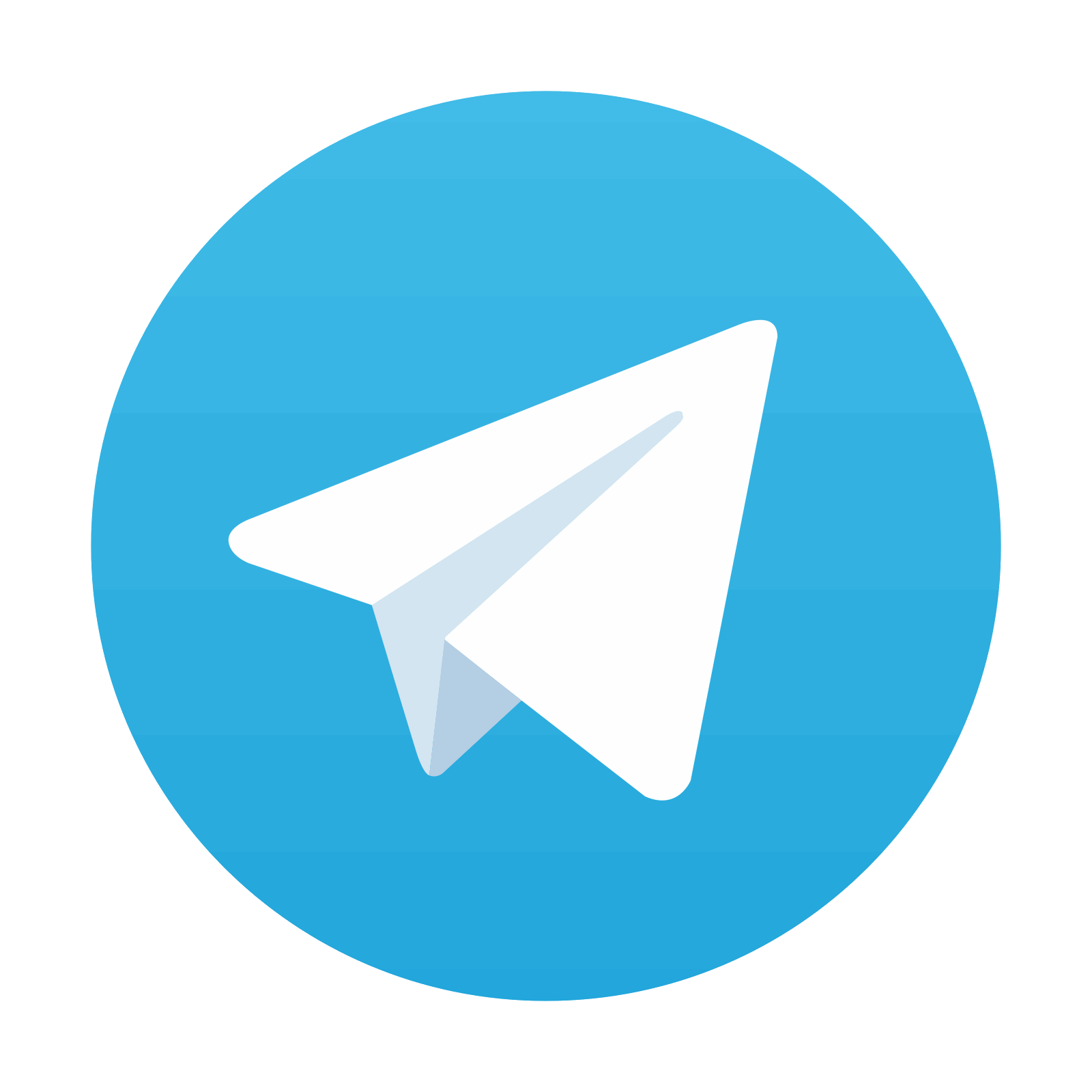
Stay updated, free articles. Join our Telegram channel

Full access? Get Clinical Tree
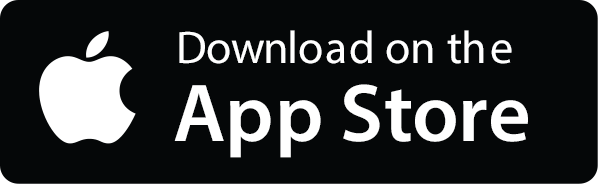
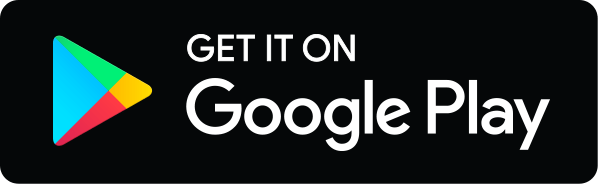