Chapter Ten Thoracic spine
Clinical anatomy and biomechanics
The biomechanics of the thoracic spine have been largely under-investigated, compared to the cervical or lumbar spines. This is probably due to the difficulties associated with the methodology of studying the movement analysis of the region, or the relatively low prevalence of thoracic pain in clinical practice (Edmondston & Singer, 1997). Considering the ex vivo studies of White (1969), Panjabi et al (1976a) and Panjabi et al (1981), Lee (1993) proposed a ‘clinical model’ of thoracic spine mechanics (Edmondston & Singer, 1997). This model was later updated (Lee, 2003, p. 44) after considering the findings of the in vivo study of Willems et al (1996).
In this model the mechanics of the spine are described considering the relative flexibility between the spinal column and the thoracic cage (Lee, 2003). The articulation of the superior costo-vertebral joint does not develop until about the age of 13 years (Dutton, 2004, p. 1243). Also, the ossification of the head and tubercle of the rib does not appear until puberty, and unites with the shaft of the rib soon after the 20th year (Warwick & Williams, 1973, p. 254). The delayed ossification allows for increased mobility of the thoracic spine relative to the rib cage, especially rotation and side-flexion (Dutton, 2004, p. 1243).
As the thoracic spine matures the superior costo-vertebral joint limits the degree of the rotation in all planes, while in old age, the ossification of the costal cartilages decreases the pliability of the relative flexibility of the thorax (Lee, 2003, p. 44).
Considering the above, there are three conditions for the spine. The first is the ‘mobile thorax’ where the rib cage is more mobile than the thoracic spine. The second is that of the ‘stiff thorax’ where the rib cage is less mobile than the thoracic spine and the third is that of same relative flexibility between the rib cage and thoracic spine (Lee, 2003, pp. 44–46). Furthermore, the anatomical differences between the regions of the thoracic spine dictate the differences in the biomechanical behaviour. Therefore, the following regions will be examined separately when this is necessary:
• The vertebro-manubrial region: T1 and T2; the first and second ribs and the manubrium
• The vertebro-sternal region: T3 to T7; the third to seventh ribs and the sternum
• The vertebro-chondral region: T8 to T10; the eighth to tenth ribs
• The thoraco-lumbar region: T11 and T12 and the eleventh and twelfth ribs.
Flexion extension
Flexion and extension are relatively plane motions coupled with only slight axial rotation (Willems et al, 1996). The range of motion of flexion and extension at each motion segment increases in the cephalocaudad direction (White & Panjabi, 1978; Willems et al, 1996). Flexion is coupled with anterior translation and some compression. Furthermore, axial compression produced flexion rotation and anterior translation of the superior vertebra (Panjabi et al, 1976a). During flexion at the vertebro-sternal region, the superior vertebra flexes and translates anteriorly relative to the inferior vertebrae. The inferior facets of the superior vertebra glide supero-anteriorly to the inferior one (Lee, 2003, p. 44).
The ribs rotate anteriorly along the axis of the neck of the rib, owing to the curved artricular surfaces of the costo-transverse joints (Warwick & Williams, 1973, p 418-419), with the anterior aspect of the rib travelling inferiorly and the posterior superiorly. Considering the ‘mobile thorax’, the ribs will continue to travel in this direction when the vertebral movement is exhausted, resulting in a coupling of superior glide and anterior roll of the tubercle at the costo-transverse joint (Lee, 2003, p. 45). Conversely, in the ‘stiff thorax’ the movement of the ribs is exhausted first while the vertebrae continue to flex. This results in an inferior glide coupled with posterior roll of the tubercle of the rib at the costo-transverse joint. For the condition of ‘same relative flexibility’ of the thoracic spine and the rib cage there is no apparent movement between the vertebrae and the ribs (Lee, 2003, p. 46).
Extension follows a similar pattern to flexion and is coupled with posterior translation and slight distraction (Panjabi et al, 1976a). This slight distraction could be due to the resistance exerted by the approximation of the facet joints during extension that allow less motion compared to the lumbar region (Oxland et al, 1992). However, in the experimental model of Panjabi et al (1976a) when posterior translation was initially induced, the coupling changed to that of slight compression. Furthermore, the study of Panjabi et al (1976a) has shown that axial distraction produces extension rotation and posterior translation.
Lee (2003) proposes that, during extension, the ribs rotate posteriorly along the axis of the neck of the rib so that the anterior part of the rib travels superiorly and the posterior inferiorly. Similarly, for the ‘mobile thorax’ the ribs continue to travel in this direction after the vertebral motion is exhausted, leading to an inferior glide coupled with a posterior roll of the tubercle at the costo-transverse joints (Lee, 2003).
For the ‘stiff thorax’ the movement of the ribs terminates prior to the vertebral ones leading to superior glide, coupled to an anterior roll of the tubercle relative to the transverse process at the costo-transverse joint. Equally for the condition of ‘equal relative flexibility’ there is no apparent movement between the vertebrae and the ribs (Lee, 2003).
At the vertebro-chondral region, the facet joints and the costo-transverse joints are more flattened and face obliquely downwards medially and backwards (Warwick & Williams, 1973, p. 418–419). The ninth often fails to form joints with the tenth rib and the tenth transverse process may not present a facet for the tubercle of the tenth rib (Warwick & Williams, 1973, p. 238) Therefore, there is minimal conjunct rotation of the ribs, with minimal motion at the costo-vertebral joint (Lee, 2003, p. 54). At the vertebro-chondral region, the zygapophyseal glide superiorly during flexion and inferiorly during extension (Lee, 2003, p. 54).
At the vertebro-manubrial region, owing to the reduced relative mobility of the first two ribs, the movement pattern is similar to the ‘stiff thorax’ pattern on the thoraco-sternal region (Lee, 2003, p. 55). At the thoraco-lumbar region, during flexion, the inferior facets of the moving vertebra translate upwards on the superior facets of the inferior vertebra, resisted only by the joint capsule. During extension, the superior facets translate inferiorly towards the superior facet of the inferior vertebra. The extension is limited by the facet joints (Oxland et al, 1992). The motion of the 11th and 12th ribs is a pure spin (Lee, 2003, p. 55).
Rotation and side-flexion
Regarding rotation and side-flexion of the thoracic spine the picture is somewhat less clear. The recent review of Sizer et al (2007) has revealed that ‘there is poor agreement among 3-D biomechanical studies that identified coupling behaviour of the thoracic spine’. From the eight studies that Sizer et al (2007) reviewed (Gregersen & Lucas, 1967; Oxland et al, 1992; Panjabi et al, 1976a; Scholten & Veldhuizen, 1985; Schultz et al, 1973; Stewart et al, 1995; Theodoridis & Ruston, 2002; Willems et al, 1996) two were in vitro (Oxland et al, 1992; Panjabi et al, 1976a;), three were in vivo (Gregersen & Lucas, 1967; Theodoridis & Ruston, 2002; Willems et al, 1996) and two (Schultz et al, 1973; Scholten & Veldhuizen, 1985) were mathematical model analyses (Sizer et al., 2007).
Six of the above studies (Gregersen & Lucas, 1967; Oxland et al, 1992; Panjabi et al, 1976a; Scholten & Veldhuizen, 1985; Schultz et al, 1973; Willems et al, 1996) examined the thoracic motion coupling using vertebral movement initiation, while two studies (Stewart et al, 1995; Theodoridis & Ruston, 2002) examined the coupling of the thoracic spine initiated by upper extremity motion (Sizer et al, 2007).
The costo-vertebral joints and the costo-transverse joints were dissected only in the study of Oxland et al (1992) and were not represented in the studies of Schultz et al (1973) and Scholten and Veldhuizen (1985). However, Oxland et al (1992) examined only the thoraco-lumbar junction kinematics. None of the studies examined the kinematics of the costo-vertebral and costo-transverse joints.
When rotation was the initiating movement in the in vitro study of Panjabi et al (1976a) and Panjabi et al (1976b), these authors reported an ipsilateral coupling of side-flexion with left rotation and a contralateral with right rotation for all the tested motion segments. Furthermore, there was a translation to the right on the coronal plane with rotation to the left and translation to the left with right rotation. A consistently ipsilateral coupling of side-flexion with rotation initiation was also identified in the mathematical model analysis study of Schultz et al (1973) and in the in vitro study of Oxland et al (1992) for the T11–T12 and T12–L1 motion segments. Conversely, the in vivo study of Willems et al (1996) revealed variable coupling patterns with the contralateral coupling pattern being more frequent in the upper thoracic segments (T1 to T4) and ipsilateral being more frequent in the rest of the segments.
When side-flexion was the initiating movement, Panjabi et al (1976a) and Panjabi et al (1976b) reported a contralateral coupling of rotation and ipsilateral pattern of translation in the coronal plane. The coupling pattern remained the same when coronal plane translation was the initiating movement. Willems et al (1996) again reported variable coupling behaviour with 47% of their observations of the upper segments (T1–T4) to be of ipsilateral coupling. 83% of T4–T8 segments and 68% of the T8–T12 segments showed an ipsilateral coupling. The remainder of their observations were of contralateral coupling of rotation. The studies of Gregersen and Lucas (1967), Schultz et al (1973) and Scholten and Veldhuizen (1985) have all reported ipsilateral coupling patterns, while Oxland et al (1992) reported no identifiable coupling pattern of rotation with side-flexion for the T11–T12 and T12–L1 segments.
The two studies (Stewart et al, 1995; Theodoridis & Ruston, 2002) that examined the coupling behaviour of the thoracic motion with upper extremity elevation, reported variable results. However, the ipsilateral coupling of rotation and side-flexion was more dominant in the studies of Stewart et al (1995) and Theodoridis and Ruston (2002). Several reasons can be responsible for the variability of the findings of the three-dimensional biomechanical studies reported above. Although the in vitro studies may have the greatest degree of accuracy, by removing the effect of posture and muscular control, they cannot be easily extrapolated in clinical practice (Sizer et al, 2007).
Further, the preparation of the cadaveric specimens of the in vitro studies involves the resection of the whole or part of the ribs or thoracic cage, ligaments, muscles and costo-vertebral joints, which increases the neutral zone and range of motion of the thoracic spine and destabilizes the thoracic spine (Andriacchi et al, 1974; Oda et al, 1996, 2002; Panjabi et al, 1981).
The age of the subjects may also play a role. For their study, Stewart et al (1995) recruited young individuals with no severe kyphosis and found no significant pattern of coupled motion, while Theodoridis and Ruston (2002) recruited older subjects and found a predominantly ipsilateral coupling of side-flexion and rotation. Thoracic disc degeneration and disc space narrowing is common after the third decade of life. Disc degeneration will increase the flexion and rigidity of the thorax and lead to reduction of lateral flexion motion earlier and, to a greater extent, any other direction (Edmondston & Singer, 1997). The study of Scholten & Veldhuizen (1985) was the only one that examined the coupling movements of the thoracic spine in flexion and found them to be strongly ipsilateral. Perhaps the latter can explain the differences between the studies of Stewart et al (1995) and Theodoridis and Ruston (2002).
It is worth noting that the studies of Gregersen and Lucas (1967), Schultz et al (1973), Panjabi et al (1976a), Panjabi et al (1976b), Oxland et al (1992) and Willems et al (1996) investigated the coupling motions with the spine in its ‘neutral’ position. This may lead to variability since there is no standardized ‘neutral’ position amongst the investigators, especially in the in vivo studies (Sizer et al, 2007).
Contrary to in vitro, in vivo investigations examine the spinal motion in the presence of the extrinsic and intrinsic factors associated with the in vivo state (Willems et al, 1996) and therefore are clinically applicable (Sizer et al, 2007). However, in vivo investigations lead to challenges in controlling a number of factors such as postural control tissue adaptation, anatomical and circadian variability within and between subjects (Sizer et al, 2007). Furthermore, by its nature, the in vivo study allows measuring of motion in only a few specific segments (Gregersen & Lucas, 1967) or measuring of motion of the spine in space rather than measuring motion of a specific motion segment (Lee, 2003, p. 49). Furthermore, as Sizer et al (2007) points out, all the in vivo studies investigated the motion of healthy subjects. Therefore, their findings might not be representative of the spinal motion of symptomatic individuals since patients with spinal pain present with an altered pattern of muscle co-activation, increased muscle activity and maladaptive postural patterns (Dankaerts et al, 2006).
As described above, side-flexion in the thoracic spine in the in vitro study of Panjabi et al (1976a) reported contralateral rotation coupling while the in vivo studies of Gregersen and Lucas (1967) and Willems et al (1996) and the mathematical model analyses of Schultz et al (1973) and Scholten and Veldhuizen (1985) reported ipsilateral rotation coupling. Lee (2003, pp 50–51) proposes a model that accommodates for these findings.
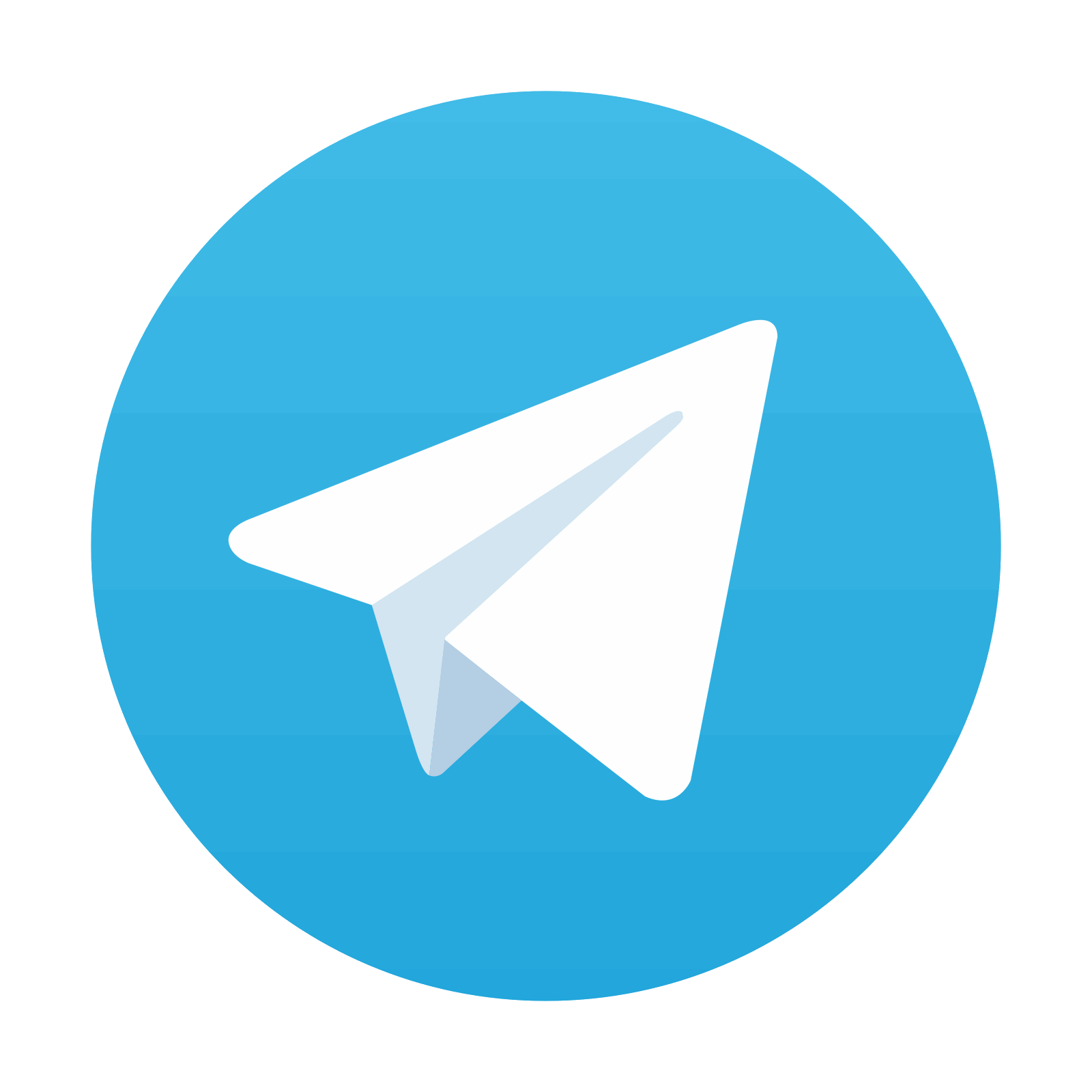
Stay updated, free articles. Join our Telegram channel

Full access? Get Clinical Tree
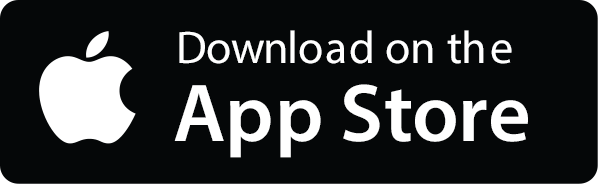
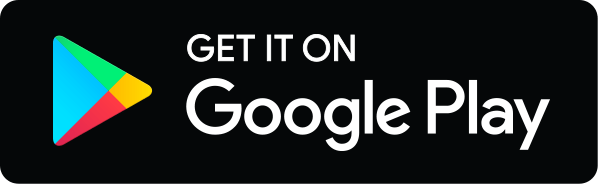