Chapter 23 Thoracic and Costovertebral Subluxation Syndromes
After reading this chapter you should be able to answer the following questions:
Question 1 | Why do the traditional movement descriptions of flexion, extension, lateral flexion, and rotation need to be modified to describe segmental spinal motion? |
Question 2 | How does altered motion in thoracic motion segments accompanying aging lead to degenerative changes in the thoracic spine? |
Question 3 | What is the clinical significance of the transition area of spinous process rotation that accompanies lateral flexion? |
Comparatively little is known about the neurologic, biomechanical, and physiologic relationships of this region of the spine. This is peculiar when one realizes that the thoracic spine houses the sympathetic nervous system and spinal dysfunction has been implicated in the development of somatovisceral reflex dysfunction, visceral dysfunction, and pain. What is known about thoracic physiology and biomechanics has been largely extrapolated from known functions of the cervical and lumbar spines. It would appear that our knowledge of spinal function has been driven by the need to understand the causes and treatment of spinal pain and therefore the lumbar spine. Secondarily, the cervical spine has captured most of our attention. Much of the literature relating to the thoracic spine is devoted to pathologic conditions and conditions that with a few exceptions have little to do with the clinical problems that are seen on a daily basis by the chiropractic clinician.
Clinical experience, however, demonstrates that pain syndromes and disorders of the thoracic spine and rib cage that do manifest themselves are quite frightening to the patient and produce anxiety about internal diseases that may or may not be relevant. Pain, pathology, and degenerative changes in this region of the spine have been shown to relate to postural changes, including scoliosis and kyphosis, autonomic and visceral dysfunction or pathologic condition, aberrant spinal static or dynamic function, or aberrant costovertebral function.1–3 Aberrant static and dynamic function is related to dysfunction of the soft tissues, including ligaments, muscles, and discs. Joint function is regulated by sensorimotor reflex feedback loops.
1. The locomotor system as a whole, as expressed in movement dynamics
2. Static body posture and our adaptation to gravity
3. The vertebral column and its function as an organ system
4. The cardiovascular and respiratory systems
5. The visceral elements and reflex responses
6. The cervical spine and its postural reflex regulation and direct muscle attachments
7. The lumbar spine, its postural regulation, and direct muscle attachments
8. The functional interaction of the thoracic spine and rib cage
Clinically Relevant Anatomy
The dorsal vertebrae are intermediate in size between the cervical and lumbar vertebrae, and they increase in size from above downward, a structural accommodation thought to relate to the increased demands of weight bearing.4 The typical thoracic vertebra is so named because of the nature of rib attachments. The second to the eighth thoracic vertebrae are considered typical and contain two pairs of demifacets. One is located on the superior posterolateral aspect of the body and one on the inferior aspect directly below, forming the articulation with the head of the ribs on each side. Viewed from the superior aspect the typical vertebra shows a heart-shaped body with relative equal anterior-to-posterior dimensions; the spinal canal is round (Figure 23-1). The left side of the vertebral body sometimes has an impression formed by the aorta, which lies along the vertebral bodies and discs. From the lateral perspective, the vertebral body is slightly thicker on the posterior than the anterior. This vertebral shape functions in forming the primary normal thoracic kyphosis. The superior and inferior disc end plates should appear parallel on radiographs. The pedicles are directed backward from the body, and the inferior intervertebral notches are large and deeper than in other regions of the spine. This results in small but adequate intervertebral foramina that are circular in form (Figure 23-2).
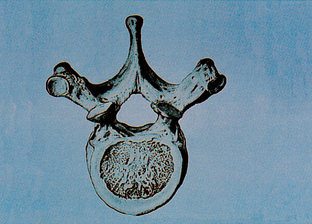
Figure 23-1 Superior view of typical thoracic vertebra.
(From Clemente C. Anatomy: a regional atlas of the human body. 4th ed. Baltimore: Lippincott Williams & Wilkins; 1997.)
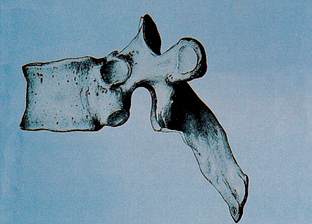
Figure 23-2 Lateral view of typical thoracic vertebra, wedge-shaped vertebral body.
(From Clemente C. Anatomy: a regional atlas of the human body. 4th ed. Baltimore: Lippincott Williams & Wilkins; 1997.)
The laminae are broad and thick, and they overlap one another like tiles on a roof. They give rise to the inferior and superior articular processes. The superior articular processes each have an oval hyaline cartilage–covered facet that is flat and faces posteriorly, slightly superiorly, and laterally. The inferior articular processes have on the anterior surface an oval hyaline cartilage articular facet that is slightly concave transversely and faces anteriorly, inferiorly, and medially. The superior and inferior facets overlap like shingles. When viewed laterally, the joints create an angle from horizontal reported to be from 60 degrees in the upper thoracic region up to 80 to 90 degrees in the lower thoracic region.5(See Figure 23-2.)
The thorax, or chest cage, is an osseous cartilaginous structure whose principal function is to protect the principal organs of respiration and circulation. The rib cage is an important functional component of respiration, and Wyke6 showed that by freezing the costovertebral joints the neurologic stimulus for respiration was suspended and respiration ceased. This fact may explain the benefit perceived by patients with chronic lung problems and asthma after adjustive care.
The spinous process of the first thoracic vertebra is thick and may be almost as long as the vertebra prominens (spinous of C7), and during flexion of the neck, it also can appear to be quite prominent. This structure makes it an excellent contact for rotational and lateral flexion adjustments for fixations/subluxations between C7-T1 and T1-2. A thumb contact, commonly called a TM (thumb move), which is described later in this chapter, should concern itself with the thoracic vertebral shape, different patient head alignment, and thrust direction during positioning to specifically affect C7-T1 or T1-2. The head position and direction of thrust also change when we consider the holding elements involved, such as the direction of the muscle force. These factors are important for success and comfort in adjusting this region. As in all areas of the spine, the anatomy and the holding elements should be considered when applying an adjustment. This review does not deal with all the adjustments in the area; rather, special attention is drawn to areas that have been shown to produce particular difficulty for clinicians in efforts to develop efficiency, specificity, and ease of adjusting. The first thoracic vertebra is such an area. It has a complete facet for the first rib and a demifacet on the inferior of the body for the articulation of the second rib. The presence of a cervical rib may complicate pain syndromes involving the upper ribs and thoracic vertebrae but provides no particular problem for treatment and, in many cases, is present for years without symptoms. Thoracic outlet problems are common and clinically are often caused by irritation coming from the multiple pain-sensitive structures related to not only the vertebral facet joints but the rib attachments and the increased number of ligaments and muscles of this region. Muscle forces that act on these joints take their attachments from the cervical spine and occiput as well as from the thoracic spine and ribs. Correction should consider the related fixations in these regions of the spine and the interaction of the muscles. Further discussion of the cervical thoracic junction is presented in Chapter 22.
The inferior thoracic vertebrae, T9-12, are also considered atypical, particularly T11 and T12, which display characteristics of lumbar vertebrae. The ninth thoracic may or may not have an inferior demifacet, whereas the tenth usually has only one demifacet, often more laterally placed on the pedicle. The eleventh vertebra is more similar in size to a lumbar vertebra, and the articular facets for the ribs are larger and are located on the pedicle, which is also larger and stronger. The spinous process is short and nearly horizontal. The transverse processes of T11-12 are short and have no articulation with the rib. The twelfth thoracic vertebra differs from the other thoracic vertebrae in that the superior facets have a coronal facing, that of a thoracic vertebra, whereas the inferior facets have saggital facing, that of a lumbar vertebra. The biomechanical demands placed on this vertebra are unique and often necessitate special attention so that symptoms may be controlled. The thoracolumbar region exhibits the highest torsional stiffness and the highest frequency of fracture.4 The transverse process is shorter, containing tubercles similar to mammillary or accessory processes formed by the attachments of muscles. These anatomic and biomechanical factors make this vertebra an important transitional vertebra for spinal curves and suggest that the optimal function of the spine occurs when the curves statically and dynamically make their transition at this level. Treatment should endeavor to restore or maintain this ideal function.
The presence of the anteroposterior (AP) curves in the spine gives it more resistance to weight-bearing forces, providing a springlike effect and reducing vibration. The thoracic kyphosis, unlike the lumbar spinal lordosis, ensures that in the neutral posture the facets carry little if any weight. Posture is such that when standing, we are inclined slightly forward. The result is that mild activity of posterior muscles, the erector spinae, maintains our posture. During unsupported sitting, there is slightly more activity of the posterior muscles.7,8 This muscle also extends up the rib cage and helps posturally stabilize the spine and rib cage as a unit. During extension, the articulating facets form a close-packed system that limits motion.
The anterior compartment of the thoracic motion segment is formed by the vertebral bodies, the intervertebral disc, and longitudinal ligaments. The anterior ligament is a strong band made up of several layers, stretching from occiput to sacrum, narrower but thicker in the thoracic region, and serves to limit extension (Figure 23-3). The posterior longitudinal ligament up the posterior vertebra bodies is also thick, widens at the discal region, and narrows at the vertebral body. The axis of motion of the thoracic segment being anterior to this ligament allows it to limit flexion and translation.
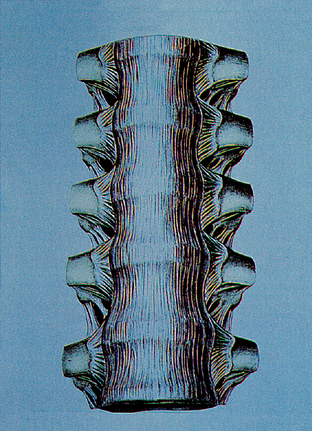
Figure 23-3 Anterior view of typical thoracic vertebra, anterior ligaments.
(From Clemente C. Anatomy: a regional atlas of the human body. 4th ed. Baltimore: Lippincott Williams & Wilkins; 1997.)
The posterior compartment is formed by the vertebral arch, the transverse processes, and the zygapophyseal joints. The articular capsules are important structures in this posterior compartment because they are pain-sensitive structures and are called on to limit the movement of the thoracic motion segments in all ranges. They are attached to the lateral margins of the articular processes and are reinforced by the ligamenta flava that connect the vertebral lamina (Figure 23-4). The latter ligaments limit flexion and, to some degree, lateral flexion and rotation, and are composed largely of yellow elastic fibers. In degenerative change, the ligament may bulge and cause irritation to the nerve root.
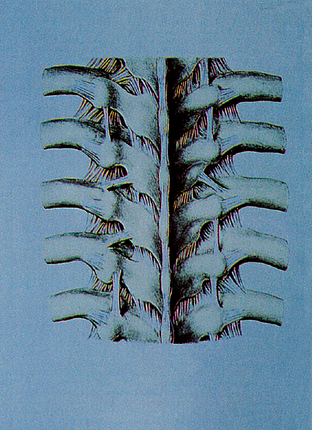
Figure 23-4 Posterior view of typical thoracic vertebra.
(From Clemente C. Anatomy: a regional atlas of the human body. 4th ed. Baltimore: Lippincott Williams & Wilkins; 1997.)
The intrinsic elements of the thoracic spine, the discs, ligaments, and vertebral structure provide the intrinsic stability that is significant up to a few kilograms.4 As in other areas of the spine, Nachemson9 showed that the nucleus exerts hydrostatic pressure that acts like a coil spring, separating the vertebrae and producing a preload stretch on the anterior and posterior compartment ligaments. Removal of the posterior ligaments, particularly the elastic ligamenta nuchae, demonstrates that the ligaments also exert compressive force or prestress on the disc, which provides for protection and enhancement of motion. As previously discussed, the rib cage also produces resistance to motion, adding to thoracic stiffness twofold and load-bearing capacity threefold (Figure 23-5).10 All of these factors are probably why disc lesions and facet problems are less frequent in the thoracic spine. The interspinous and supraspinous ligaments limit flexion and are well developed in the thoracic region. Tenderness of the supraspinous in the mid-thorax, a common clinical finding, would seem to demonstrate that this region of the spine is frequently under flexion strain. Degenerative change in the anterior aspect of the dorsal vertebrae is also common, which may relate to increased compressive forces or at least to excessive anterior mobility of the body.
Biomechanics of the Thoracic Spine
Static Concepts
Gray’s Anatomy11 holds that the cervical curve starts at C2 and ends at T2, the thoracic curve starts at T2 and extends to T12, and the lumbar curve starts at T12 and ends at L5. The normal apex of the thoracic curve is T7. The spine was normally seen to have a very slight lateral curve convexity to the right, thought to be caused by muscular development related to dominant right-handedness. Schmorl and Junghanns12 reported that it was normal for 80% of spines to have a mild physiologic scoliosis, left in the cervical thoracic region and right in the thoracic and lumbar regions, whereas 20% of spines showed the reverse of this curve. These curves begin developing at 6 years of age; they were thought to be related to greater strength of right thoracic musculature caused by dominant right-handedness. It is interesting to note, however, that Figure 51 of their text, depicting these curves in a preparation by Virchow, is more representative of the four opposed rotational scolioses seen as normal and presented in chiropractic literature by Carver,13 Beatty,14 and Homewood.15
The Four Opposed Rotational Scolioses (Carver)
1. Right lower lumbar curve extending to L3; 3 transitional vertebrae
2. Left lumbar thoracic curve; apex at T12 and termination at T7-8, the transitional vertebrae
3. Right thoracic cervical curve; apex at T4, transition at C7-T1
4. Left cervical scoliosis, apex at C4, with a counteraction at C1-2, C1 right, C2 left
Carver’s opinion was that the apex of the thoracic kyphosis was at T7-8, with the normal curve extending from T2-12. There appears to be some general agreement in the literature that the basic asymmetry of the body as well as dominant handedness produces mild rotational curves in the spine that develop in early childhood. A left cervical curve and a right thoracic curve may by all reports be a normal functional compensation for right-handed individuals and may account in part for the common low right shoulder seen in right-handed individuals. It is surprising how often in clinical practice one encounters a spinally healthy individual with the mild curves Carver considered as optimal—the four opposed rotational scoliosis. The key factor in a functional spine seems to be symmetry of mobility and balanced compensation rather than strictly alignment. The most important clinical factors in assessing the statics of the spine would be (1) the curves should be smooth and even, and each individual vertebra should participate uniformly in the configuration; (2) the apex and transitions of the curves should be at the levels just stated; and (3) the spine should fall so that the center of gravity passes through the center of the body of C7 and the center of the body of T12 with only a mild deviation (scoliosis) presented on postural analysis. Dynamic motion should be equal to each side, and the curve should be uniform; that is, each motion segment should participate smoothly and somewhat equally, reaching the end point of the motion with uniform rhythm.
Dynamics of the Thoracic Spine
The thoracic spine is the longest region of the spine but the least mobile. This lack of mobility is caused primarily by the fact that the height of the disc is approximately 20% to 25% that of the height of the thoracic vertebral body, a ratio that is the lowest in the spine. Additionally, the rib cage with the double posterior and anterior costosternal attachments limits rotation and lateral flexion. When the sternum is removed, the stiffness of the thoracic spine imparted by the rib cage is negated.4 These movements are slightly less limited in the lower thoracic region, where the elasticity of the costal cartilages allows for a slightly greater degree of mobility in certain ranges. The thoracic vertebral facet facings limit flexion and extension to some degree but do little to impede rotation and lateral flexion of the thoracic segment, which is mainly limited by the ribs. During extension the facets are seen to make contact, which limits movement along with the anterior longitudinal ligament. All motion in the thoracic spine is accompanied by movement in the ribs, which at times amounts to only a few millimeters of translational motion. Thoracic biomechanical dysfunctions, whether vertebral or costovertebral, are intimately related because of the osseous structural arrangement and the muscular attachments. When correcting dysfunction, concern for static alignment, joint motion, and the extrinsic forces that block the movement are of importance. White and Panjabi16 emphasized six degrees of freedom in the thoracic motion segment similar to the findings in the lumbar spine: (1) anterior/posterior motion or translation along the Z axis; (2) lateral to medial motion around the X axis; and (3) superior to inferior motion around the Y axis. The traditional descriptors flexion, extension, lateral flexion, and rotation do not conform to the actual movements in the spine because all movements are combined or coupled movements. The primary coupled motion is easily detected on a motion study plain film radiograph, and it is well understood that lateral flexion is combined with rotation and vice versa. It is important to note that tertiary motions in translation, flexion or extension, or motion along the Y axis also accompany these main motions. Clinicians often believe that when the tertiary motion is lost in a motion segment (joint blockage or subluxation), the neurologic reflex changes that take place are the most significant to the patient and explain some of the dramatic results obtained by the dynamic adjustive thrust when evaluation demonstrates little static or dynamic change other than the restoration of the paraphysiologic or tertiary motion. Panjabi et al.17 showed that the average intervertebral translation motion was 1 mm in the sagittal plain. This amount of motion can be felt only through the end feel challenge method and clinically can be appreciated as altered after a dynamic adjustive correction. At times the palpable restoration of motion may represent restoration of tertiary motion, paraphysiologic motion, or translatory motion that may prove sometimes to be the same motions.
The axis of motion for rotation and lateral flexion is located in the center of the body of the vertebra and under normal circumstances very little shearing or translatory motion of the disc takes place. The axis of motion for flexion-extension is located in the center of the disc of the vertebra below and does not allow or require much shearing or translation. Clinically, anterior disc lipping and degeneration are common radiographic findings in middle age.18,19 One cause of these pathologic changes is probably a shift of the axis of motion posteriorly and laterally caused by alteration in muscular activity that results in hypermobility of the anterior body during movement with resultant increased shearing force to the disc and stress and strain to all of the intrinsic structures. The alteration of movement or function produces a change in structure, resulting in the degenerative changes commonly seen in the anterior body of the thoracic spine and the osteophytic changes at the costotransverse joints.
The musculature of the thoracic spine is dealt with in detail in most anatomy texts. The action is usually described on a kinesiologic basis with such descriptions as extensors/rotators or lateral flexors of the spine. For the clinician involved in spinal correction, the specific attachment of these muscles and their individual actions and effects on the motion and biomechanics of the individual vertebra are important. Some of the discussion in this area must be theoretical at this stage because of limited experimental research and technology to validate our opinions; however, speculation based on structure, function, and observation is an important first stage. The Quiring and Warfel series20,21 on musculature is a very good reference source for the clinician to use for the details of origin, insertion, nerve supply, and motor points. The detailed drawings allow us to speculate on the biomechanical action these muscles exert on the individual motion segment.
The rhomboids arise from the ligamentum nuchae, the spinous process of C7, and the spinous processes of T1-5. They insert into the medial border of the scapula. In the prone position, elevation of the scapula and palpation of these muscles as well as of the trapezius can help determine if hypertonicity is present in these more superficial muscles or the deep muscles of the back.
The main muscles that control thoracic biomechanics are the trunk muscles, the erector spinae, and the transversospinal group (Figure 23-6).
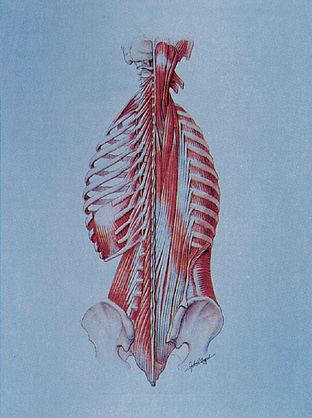
Figure 23-6 Erector spinae and transversospinal muscles.
(From Seeley RR, Stephens TD, Tate P. Anatomy and physiology. 3rd ed. St. Louis: Mosby; 1995.)
The erector spinae group is made up of the (1) iliocostalis, (2) longissimus, and (3) spinalis.
2. Longissimus Portion
a). Thoracic portion origin of transverse processes of lumbar spine and the lumbodorsal fascia; insertion into the transverse processes of the lower 9 or 10 ribs
b). Cervicis portion originates from upper fourth to fifth thoracic transverse processes with an insertion into the transverse processes of C2-6
c). Capitus portion originates from transverse processes of the upper four to five thoracic and articular processes of lower three to four cervical vertebrae; insertion is into the posterior margin of the mastoid of the occiput
3. Spinalis Portion
a). Thoracic portion arises from spinous processes T11-12, L1-2; insertion is the fourth through the eighth thoracic spinous processes
b). Cervicis portion arises from spinous processes of T1-2, C7, and ligamentum nuchae; insertion is spinous processes of C2-4
c). Capitus portion originates from transverse processes of the upper six to seven thoracic vertebrae and articular pillars of C4-7 cervical vertebrae. Insertion is between inferior and superior nuchal lines on the occiput. It should be noted by the clinician at this point that much of the attachment of these muscles is devoted to attachments to the ribs. These attachments help to stabilize the head of the rib into the thoracic transverse processes.
Transversospinal Muscles
Semispinalis capitus has its origin from the transverse processes of T1-6 and C7 as well as articular pillar C4-6 and the insertion into the occipital line between superior and inferior nuchal line. Multifidus is a segmental muscle not as well developed in the thoracic region as in the lumbar spine or the cervical spine. The origin is from the spinous process of the vertebra above; the insertion is into the transverse processes of vertebra below and extending as far down as three to four segments. The rotational components of the thoracic segments are further supported by rotatory muscles, which lie deep to the multifidus in pairs and are present only in the thoracic spine. The origin is from the transverse process of one vertebra and the insertion is into the lamina of the vertebra above. Segmental motion is further supported by the interspinales muscles and intertransversarii muscles, which are very small or even nonexistent in some thoracic spines. Clinically these latter muscles may have little significance.
Kinematics of the Thoracic Spine
Kapandji22 reports movement of the spine as a whole. His diagrams demonstrate 45 degrees of flexion-extension, 20 degrees of lateral flexion to each side (total of 40 degrees), and 35 degrees of rotation to each side (total of 70 degrees). Gregerson and Lucas’s study23 of normal medical students using Steinmann’s surgical pins inserted into the spinous processes showed an average segmental rotation of 6 degrees. They showed a slight difference between sitting and standing, with sitting showing less motion in the lower thoracic vertebrae. They reported a total range of motion similar to the figures suggested by Kapanji22(37 degrees to one side, or a total of 74 degrees of rotation). In this same study, rotation during gait was observed. During normal walking, T7-8 was seen to stay relatively stable and formed the transition point for total body rotation developed by the pelvis, opposed to the counter-rotation seen in the shoulders with normal arm swing. With each step, the gross rotation motion of the body at the sacrum is approximately 8 degrees, which reduces to 0 degree at T7-8. The arm swing and shoulder motion produce an opposite rotation of 6 degrees at T2. Therefore T7-8 would show the most rotation segmentally during normal walking (approximately 2.5 degrees). The segmental rotation at T11-12 and T1-2 during walking amounts to about 0.5 degree. These biomechanical observations would lend logical support to the concept that T7 should be the optimal apex of the thoracic kyphosis. The most definitive study of the biomechanics and the range of segmental motion in the thoracic region comes from White and Panjabi24(Table 23-1).
Flexion-Extension Range of Motion: Sagittal Plane Rotation
Median figures are 4 degrees of motion for the upper thoracic segments, 6 degrees for the mid-thoracic segments, and 12 degrees of motion each for the T11, T12, and L1 segments. White and Panjabi25 suggested that the instantaneous axis of motion for flexion is located in the anterior one third of the superior aspect of the vertebral body of the vertebra below; for extension, the location is in the anterior one third of the inferior aspect of the vertebra of the superior motion segment (Figure 23-7).
Lateral Flexion Range of Motion: Frontal Plane of Motion
As in other areas of the spine, lateral flexion is accompanied by a coupled motion; the main coupled motion is rotation in the sagittal plane. Lateral motion totals approximately 52 degrees to each side with intersegmental movement of approximately 5 to 6 degrees in the upper segments and 8 to 9 degrees in the lower segments. During lateral flexion the instantaneous axis of motion is located in the body of the vertebra below. Lateral flexion on plain film radiographs demonstrates that the primary coupled motion, generally in the upper thoracic vertebrae, is similar to a cervical motion segment in that the spinous processes rotate to the convexity (type 2 motion). In the lower thoracic vertebrae, the normal behavior is similar to that of a lumbar segment; the spinous processes rotate to the concavity (type 1 motion). My own clinical observations of coupling patterns suggest that the optimal or normal pattern for the upper thoracic segment is a type 2 pattern and for the lower thoracic segment a type 1 pattern, the transition point being T6-7. The typing of motion patterns was developed in the lumbar spine and reported by Grice,26,27 Cassidy,28 and Gitelman.29 These observations can be clinically produced by a radiographic study that places the seated patient with the dorsal spine against the bucky and laterally flexioning the patient, keeping the spine in contact with the bucky. Deviations from this pattern are common and in my opinion are clinically significant on a long-term biomechanical basis. White and Panjabi4 discuss the disagreement about coupling patterns in the thoracic region and suggest that the complexity of the motion along with variation in techniques of study may be the reason. They go on to observe that abnormal coupling patterns are seen in scoliotic deformities.
Rotation Range of Motion: Motion in the Horizontal Plane
The rotation range of motion for the whole thoracic spine is 41 degrees. Each vertebral segment in the upper half of the thoracic spine moves approximately 4 degrees to each side with a total of 8 to 9 degrees, whereas the lower three thoracic segments move only about 2 degrees to each side.4
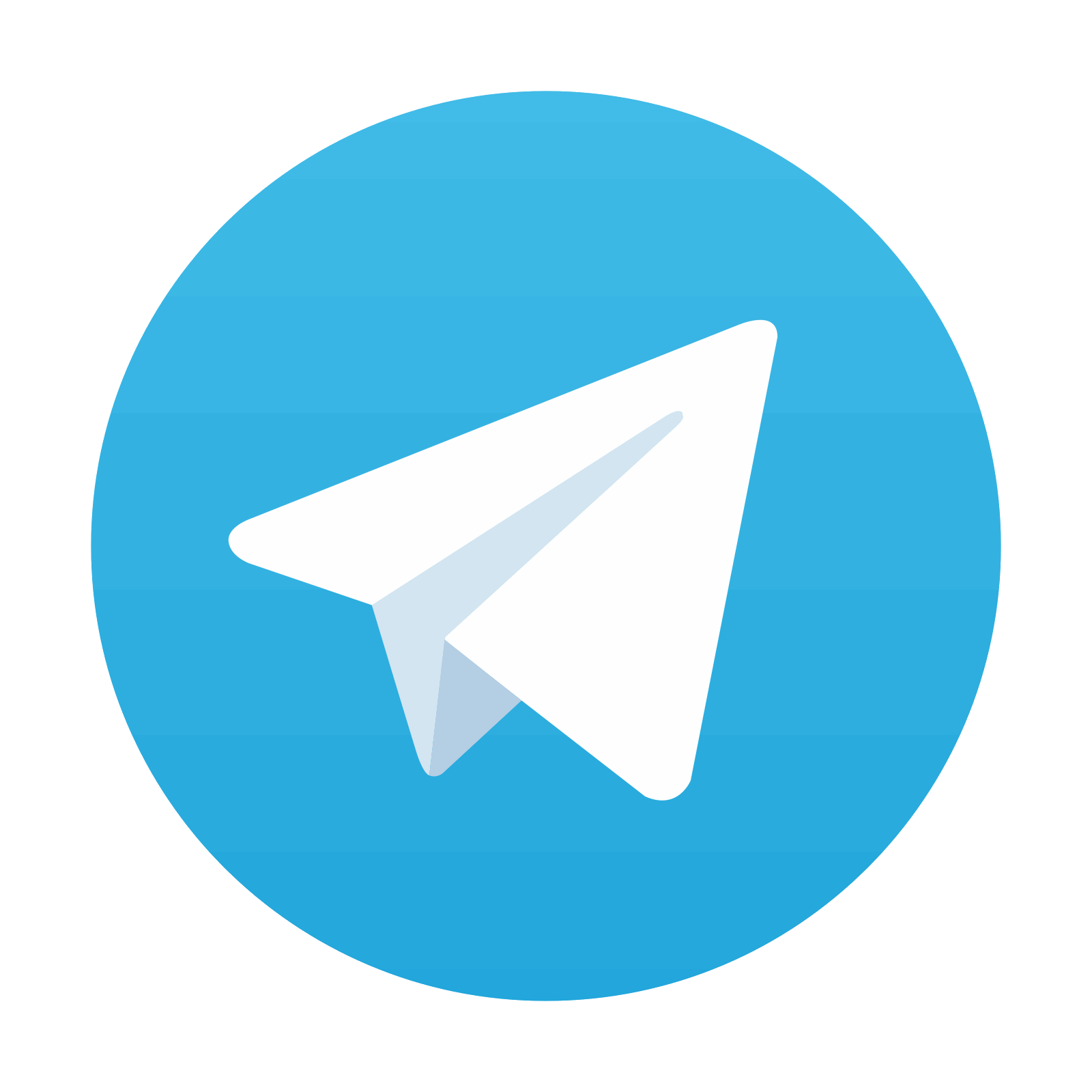
Stay updated, free articles. Join our Telegram channel

Full access? Get Clinical Tree
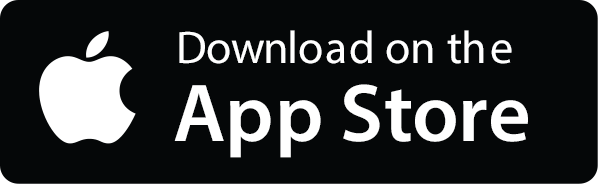
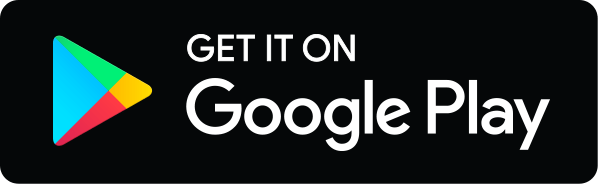