Fig. 24.1
Meniscus vascularity. During the gestational period, the whole meniscus is vascular. After birth, the inner part starts to lose its vascularity due to endostatin accumulation. Around the 11 years of age, the inner part becomes avascular (Reprinted from Springer Cellular and Molecular Bioengineering, Sanchez-Adams and Athanasiou [57], figure 3, with kind permission from Springer Science and Business Media)
During the gestational period, the developing menisci are highly cellular in the beginning and the nucleus–cytoplasm ratio is high. In time, these cells mature and the nucleus–cytoplasm ratio declines. While the number of cells decreases, their collagen production increases. Collagen fibers begin to orient in a circumferential manner. Locomotion and postnatal loading of the knee joint are thought to have important roles in the development of orientation of collagen fibers [8, 17].
Anatomy
Medial and lateral menisci which are located between femoral and tibial articular surfaces resemble a wedge in coronal plane. The two menisci are not completely identical. The medial meniscus has a crescent shape, anterior of which is wider when compared with its posterior part. However, the lateral meniscus is more circular, and its wideness remains the same throughout its anterior and posterior parts. The medial meniscus covers 60 % of the medial tibial plateau, whereas the lateral one covers 80 % of the lateral tibial plateau. Peripheral parts of the menisci are thick, convex, and continuous with the joint capsule. Their inner parts have thin, free ends. The upper surfaces of the menisci are concave which ease their articulation with the corresponding convex femoral articular surfaces. Their lower surfaces that attach to the tibia are flat. The congruency of the shapes of the menisci with their corresponding upper and lower articular surfaces diminish the friction emerging during joint motion [8, 17, 20, 57].
The anterior horns of both of the menisci are attached to each other via transverse intermeniscal ligament (Fig. 24.2). The anterior and posterior horns are attached to the tibia via insertional ligaments. The insertion site of the anterior horn of the medial meniscus is in the anterior intercondylar fossa which is just anterior to the anterior cruciate ligament (ACL). The posterior fibers of the anterior horn blend with the transverse meniscal ligament. The posterior horn is attached to the posterior intercondylar fossa which is situated between the posterior cruciate ligament (PCL) and the insertion site of the posterior horn of the lateral meniscus. The anterior horn of the lateral meniscus is attached close to the ACL, anterior to the tibial spines. The insertion sites of the anterior and posterior horns of the lateral meniscus are closer to each other when compared with that of the medial meniscus. The peripheral part of the medial meniscus is attached to the joint capsule via meniscotibial (coronary) ligaments. Also, the middle part of the medial meniscus is firmly attached to the deep part of medial collateral ligament. As a result of these anatomic characteristics, the mobility of the medial meniscus is limited. Unlikely, the lateral meniscus is not in relation with the lateral collateral ligament. In addition, there is the popliteal hiatus which is situated in the posterolateral part of the meniscus and constitutes one third of its posterior part with the popliteus tendon passing through and has no relation with the capsule. When compared with the medial meniscus, the lateral one is more mobile [11, 17, 20].
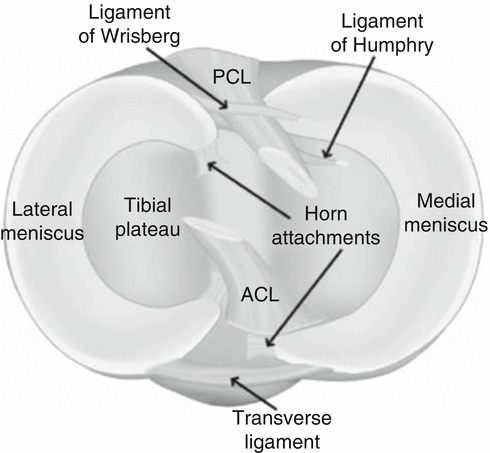
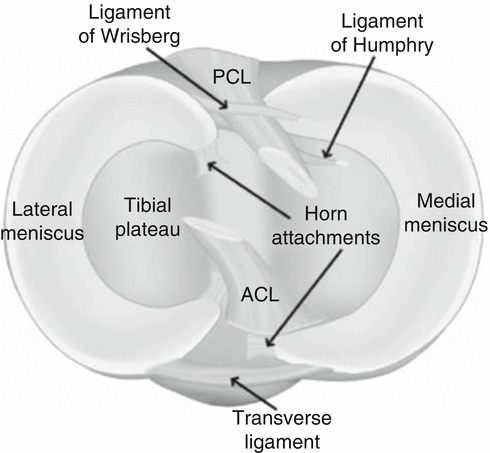
Fig. 24.2
The schematic axial showing the medial and lateral menisci. Anterior horns of the two menisci are attached to each other via the transverse meniscal ligament. The posterior horn of the lateral meniscus is attached to the lateral part of the medial femoral condyle via Humphrey and Wrisberg ligaments (Reprinted from Springer Cellular and Molecular Bioengineering, Sanchez-Adams and Athanasiou [57], figure 2 with kind permission from Springer Science and Business Media)
The posterior horn of the lateral meniscus is attached to the lateral part of the medial femoral condyle via anterior (Humphrey) and posterior (Wrisberg) meniscofemoral ligaments. The anterior meniscofemoral ligament passes anterior to PCL, and posterior to it, there is the posterior meniscofemoral ligament. The attachment site at the medial femoral condyle is in close proximity with the attachment site of PCL. As a result of studies conducted on cadaveric knees, a consensus has not been reached about the incidence and number of meniscofemoral ligaments. Though their function is not absolutely ascertained, it is suggested that they restrict the mobility of the lateral meniscus by pulling its posterior horn both anteriorly and medially throughout the range of motion [20, 22, 24].
The developmental variations of the lateral meniscus are more abundant than that of the medial meniscus. Discoid meniscus is more commonly observed in the lateral meniscus. In the past, discoid meniscus was thought to be formed as a result of the cessation of development; however it was not possible to show that the meniscus possessed a discoid shape at any time during its development [8, 50].
Vascular Supply
The menisci of an adult knee are relatively avascular structures, and their vascular pattern is strongly related to its healing capacity. According to their vascularization, adult meniscus is divided into three zones. The outermost one third of it is regarded as the “red–red zone” which has an abundant blood supply. The middle one third part, named as the “red–white zone,” has a scarce vascularization when compared with the red–red zone. The innermost one third is the avascular “white–white zone” (Fig. 24.3). When compared to its body, the insertion sites have richer vascularization [37].
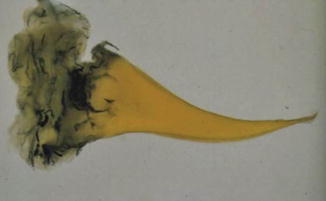
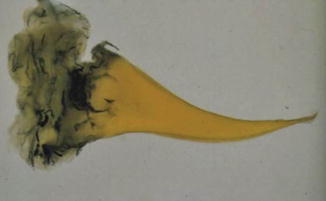
Fig. 24.3
Blood supply to adult’s meniscus. Vascularization of the outer part of the meniscus from the perimeniscal plexus is observed (Reprinted from Arthroscopy: The Journal of Arthroscopic and Related Surgery, Stärke et al. [61], with permission from Elsevier)
Medial and lateral and superior and inferior geniculate arteries branching from the popliteal artery form a perimeniscal capillary plexus which supplies the peripheral parts of the menisci. Both medial and lateral menisci have synovial folds over the peripheral parts of both femoral and tibial sides which possess short terminal vessels. Only the popliteal hiatus part of the lateral meniscus is devoid of this blood supply [9, 11, 21, 48].
Along with a few terminal branches of medial and lateral geniculate arteries, middle geniculate artery contributes to the vascularization of the menisci via the synovial folds of the anterior and posterior horns. These synovial vessels enter the tissue directly passing through the insertional ligaments and constitute short, terminal, endoligamentous vessels supplying the horns [7, 11].
The vascularization of the rest of the meniscal tissue is provided by either diffusion from the synovial fluid or mechanical pumping. Histological studies performed utilizing scanning electron microscopy revealed that there were canal-like structures opening to the surface of the menisci. The function of these canal systems has not been identified yet; nevertheless it is suggested that they had roles in the regulation of the hydrostatic pressure of the menisci and facilitation of liquid flow under loading conditions, providing supply to the avascular parts from the synovial fluid and blood vessels [1, 5].
In order to obtain diffusion from the synovial fluid, the menisci should be subjected to cyclic loading and stress relaxation provided by body weight and muscle force. During infancy since the menisci could not be exposed to loading conditions, it was claimed that diffusion from the synovial fluid did not contribute to their vascularization which was only supplied by blood vessels. Following the beginning of bipedal walking or after the muscles start to exert sufficient amount of forces on the menisci, the vascularization pattern changes. The menisci whole of which are supplied by blood vessels are replaced by adult-type menisci whose inner parts are avascular [21, 48].
Neuroanatomy of the Meniscus
Posterior articular branch of tibial nerve is responsible for most of the innervation of the meniscus. Medial articular branch of saphenous nerve also contributes to the innervation of the medial meniscus [15]. The larger nerves have a circumferential pattern in the perimeniscal tissue accompanying blood vessels. The smaller nerves or single axons have a radial course toward the outer one third of the meniscus. When compared with its middle part, the outer part of meniscal body is richer in nervous tissue, whereas the inner one third is devoid of it. Both the anterior and posterior horns possess more abundant neural tissue than the rest of the meniscus. As well as its vascularization, the majority of the nerves are observed in the horns and its periphery. The innervations of the medial and lateral menisci are alike [9, 15, 42].
Mechanoreceptors are specialized receptors that convert physical stimulus such as tension, compression, change of position, and acceleration to electrical neural stimulus. Mechanoreceptors are most commonly found in the horns of the menisci with the posterior horn having more than that of the anterior horn. In the horns and the periphery of the meniscus, three types of mechanoreceptors were identified. Type I (Ruffini) mechanoreceptors are responsible for the sensation of static joint positioning and changes in pressure. These are slowly adapting receptors with low threshold for activation. Type II (Pacini) mechanoreceptors are rapidly adapting receptors with also low threshold for activation which sense changes in tension and acceleration. Type III (Golgi) mechanoreceptors are slowly adapting receptors with high threshold for activation which are activated when the extremes of range of joint motion are achieved and initiate a protective reflex inhibition [1, 7, 9, 17, 21].
The proprioceptive information supplied from the menisci reaches the central nervous system which may contribute to the anteroposterior translation of femur over tibial plateau. In addition to the mechanical characteristics of menisci, their proprioceptive features may also have some effects in the knee joint stability. The proprioception of the knee joint was shown to decrease significantly in case of isolated meniscal injuries [1, 21, 30].
Histological and Biochemical Features of the Meniscus
The meniscal tissue which is fibrocartilage in nature is composed mainly of extracellular matrix and relatively scarce and scattered cells. There are three types of cells within the meniscal tissue. The cells located at the peripheral part are oval- or spindle-shaped cells which resemble fibroblasts morphologically. They possess thin, long, cytoplasmic processes which are used to communicate with other cells and extracellular matrix. These cells have abundant cytoplasm which is rich in granular endoplasmic reticulum and Golgi apparatus. They do not have pericellular matrix; instead, the collagen fibers of the extracellular matrix (which are mainly type I but to a lesser extent types III and V) surround these fibroblast-like cells. The cells of the inner menisci are more oval in shape and are surrounded by pericellular matrix. Owing to their resemblance to chondrocytes, they are called fibrochondrocytes or chondrocyte-like cells. The extracellular matrix around them contains mostly type II collagen, besides much less but significant amounts of type I collagen and glycosaminoglycan more than the outer menisci. Considering the fact that this part of the meniscus contains type II collagen and aggregan which is a proteoglycan, it resembles hyaline articular cartilage. The third type of cells is the ones located at the superficial part of the meniscus which is spindle shaped similar to the fibroblast-like cells but flatter than them and does not possess cytoplasmic processes. They are considered as progenitor cells with certain capabilities [25, 28, 37, 54].
Healthy meniscal tissue is composed of water (70–75 %), collagen (20–22 %), glycosaminoglycan (0.6–0.8 %), and deoxyribonucleic acid (DNA) (0.10–0.12 %) [28]. The collagen constituting meniscus is 90 % type I collagen and to a much lesser extent type II, III, V, and VI collagens. Type I and II collagens form the fibrillar skeleton of the meniscus. The functions of type III and V collagen are not clearly identified. However, type VI collagen is a matrix glycoprotein and responsible for the stabilization of the collagen skeleton and persistence of the attachment of fibrochondrocytes to matrix [54].
The orientation of collagen fibers is related to the mechanical properties of the menisci and their tearing patterns. The histological evaluation of meniscal sections revealed that it had three distinct layers. The superficial layer which exists both on the tibial and femoral surfaces is composed of a thin collagen fiber network whose fibers are approximately 30 nm in diameter. Underneath this reticular layer, there is a lamellar layer which also exists both on the femoral and tibial sides. The collagen fibers of the lamellar layer are radially oriented at the outer parts of the anterior and posterior horns; at the inner parts, they intersect at various angles. The main part of meniscal tissue is located between the two lamellar layers. The orientation of collagen fibers in this central layer is circumferential in the whole menisci. In addition, there are few radial fibers which connect the circumferential collagen fibers to each other (Fig. 24.4) [49, 54].
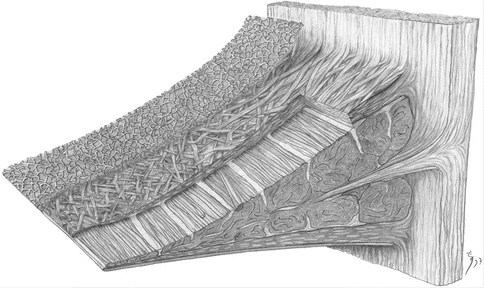
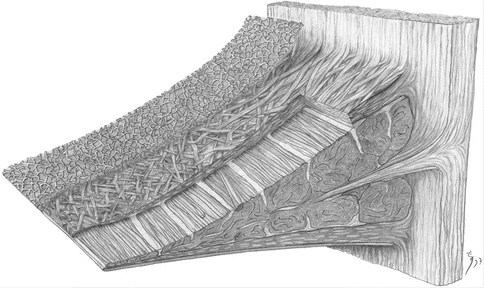
Fig. 24.4
Synoptic drawing of a meniscal section showing the superficial and lamellar layers both on the tibial and femoral surfaces and in between the central main layer. In the central layer, circumferentially oriented collagen fiber bundles are observed. A few radial fibers also intersect them (Reprinted from Springer-Verlag Anatomy and Embryology, Petersen and Tillmann [49], figure 5, with kind permission from Springer Science and Business Media)
When the knee joint is subjected to axial loading, the menisci are compressed and pushed away from the center of the joint, which in turn leads to increased tensioning in their outer parts, named as “hoop stresses.” The tensioning is very well tolerated by the circumferentially oriented collagen fibers; however radial fibers are not durable enough. This phenomenon is considered in the etiology of longitudinal meniscal tears [49, 54].
The proteoglycans which are among the components of the organic part of noncollagen meniscal tissue are composed of a core protein and one or several glycosaminoglycan (GAG) chains attached to it. The amount of GAG of a meniscus is eight times that of articular cartilage [2]. The GAGs found in the meniscal tissue are chondroitin 6-sulfate (40 %), chondroitin 4-sulfate (20 %), dermatan sulfate (20 %), keratin sulfate (15 %), and hyaluronate (3 %) [28]. GAGs are negatively charged hydrophilic molecules; because of this property, the meniscal tissue is resistant to compressive stresses. The collagen network and GAGs of the menisci constitute a porous, permeable, solid matrix which is essential for the preservation of the hydrostatic pressure of tissue. In loading conditions, there is an influx of fluid into the tissue followed by the deformation of solid matrix which is responsible for the shock-absorbing property of the meniscus. The meniscal regions having the most abundant GAG content are subjected to the highest loading conditions [7, 17, 28, 54]. Aggregan is the main proteoglycan of the meniscal tissue and responsible for the viscoelastic, compressive properties. Decorin, biglycan, and fibromodulin are other proteoglycans found in low amounts in the meniscal tissue [17, 54, 58].
Matrix glycoproteins have a role in adhesion and connect cells to the components of extracellular matrix. They contribute to the structural and mechanical strength of the tissue. Type VI collagen, fibronectin, and thrombospondin are among the glycoproteins found in the meniscal tissue. Elastin is a noncollagen fibrillar protein found in the matrix of the meniscus which has a role in regaining the shape following deformation due to loading [1, 7, 17, 37, 54].
Biomechanical and Functional Properties of the Meniscus
The biomechanical features of the menisci are related to their anatomic and viscoelastic material characteristics which make them possess several important functions in the joint. Load bearing, shock absorption, and joint lubrication are among the functions of menisci. They also provide support to joint stability and have roles in proprioception [32, 54, 59].
The two incongruent joint surfaces, the distal femur and the proximal tibia, become congruent to each other as a result of meniscal function. They also have a role in load sharing. By means of menisci, the tibiofemoral contact area enlarges which in turn decreases the contact stress during loading. Since the menisci move together with the femur and tibia throughout the range of motion, they provide congruency of the tibiofemoral joint and participate in load sharing. This dynamic congruency also contributes to the joint stability and lubrication [68].
During loading, the menisci transmit 70 % of the load in the lateral compartment and 50 % in the medial compartment. In extension, they transmit 50 % of the load, whereas in 90° of flexion, they transmit 85 % of it and protect the articular cartilage against compressive stresses. The menisci are exposed to compressive, tensile, and shearing forces. By means of circumferential collagen fibers and the insertion characteristics of the anterior and posterior horns, vertical compressive stresses are converted to horizontal hoop stresses which prevent them from extrusion (Fig. 24.5). The tensile properties of the menisci facilitate load bearing and shock absorption functions [6, 37, 68].
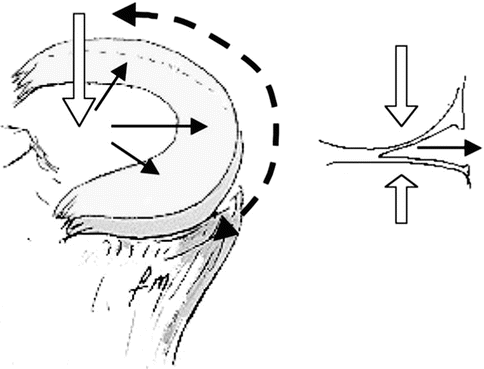
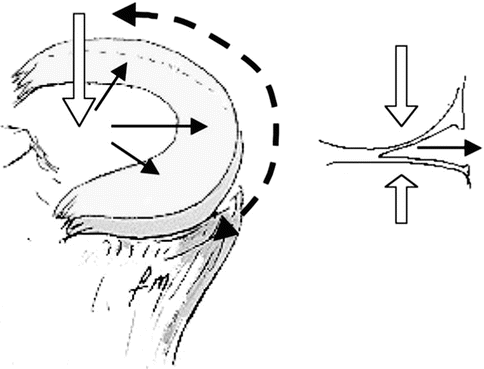
Fig. 24.5
A compressive force (white arrow) is converted a radially directed force (black arrows), which is taken up as hoop stresses (dashed arrow) within the meniscus (Reprinted from Boyd and Myers [6], with permission from Elsevier)
As well as other biological tissues, the menisci respond to loading in two steps. The first step is mechanical or elastic respond to loading. Compression of solid matrix and fluids inside the tissues leads to hydrostatic pressure increases. The second step is the efflux of liquids which is time dependent. Morphological changes which are biomechanically referred as “creep” occur in the meniscus facilitating the increase in the congruency and contact area between two corresponding joint surfaces. Efflux of liquid lowers the hydrostatic pressure of the meniscus which is called stress relaxation. The flow continues until a balance is reached between the applied pressure, hydrostatic pressure of the matrix, and tensile forces exerted by the collagen fibers [1]. When compared to the articular cartilage, its compressive stiffness and permeability are lower which is related to its shock absorption properties [32]. Though the exact mechanism of joint lubrication is not identified, it is considered to be related with the efflux of liquids from the meniscal tissue into the joint space. The menisci contribute to the nutrition of articular cartilage by pushing the synovial fluid against cartilage surfaces and protecting the synovial film layer over the articular cartilage [17, 32, 68].
It was demonstrated that under loading conditions, the anterior horn of the medial meniscus moved a mean of 7.1 mm, and the posterior horn 3.9 and 3.6 mm in the mediolateral direction with knee flexion; on the other hand the anterior horn of the lateral meniscus moved 9.5 mm, and the posterior horn 5.6 and 3.7 mm in the mediolateral direction. The results of this study showed that the lateral meniscus was more mobile than the medial one and the anterior horns were more mobile than the posterior ones. The mobility of the menisci throughout the range of motion increases the conformity of joint surfaces and protects them from injuries [68]. The higher incidence of meniscal tears occurring in the posteromedial part of the medial meniscus is explained by the fact that this part is more stagnant [6].
Partial or complete loss of the meniscus increases point loading which leads to early degeneration due to alterations of mechanical vectors. In a study conducted on cadaveric knees which evaluated the tibiofemoral contact mechanics, medial contact area and medial peak contact stress values were measured in the knees with intact meniscus or 50 %, 75 %, or total meniscectomized knees at extension and 30 and 60° of flexion. In case of an intact meniscus, the largest medial contact area was observed at extension; with increasing flexion, medial contact area decreased. The comparison with the intact meniscus revealed that medial contact area decreased 20 % in the 50 % meniscectomized knees, 35 % in the 75 % meniscectomized knees, and 54 % in total meniscectomized knees. Medial peak contact stress increased 43 % in the 50 % meniscectomized knees, 95 % in the 75 % meniscectomized knees, and 136 % in total meniscectomized knees [34]. The type of meniscal tears is also important. The radial tears reaching the periphery of meniscus do not necessarily change the volume; however the ability to withstand hoop stresses is influenced which alters the functional capability of the meniscus [6].
Another important function of the meniscus is to contribute to the joint stability. The medial meniscus with its wedge shape limits the posterior movement of medial femoral condyle over tibial plateau which has limited influence on knee joint stability under normal circumstances. However, in knees with especially ruptured anterior cruciate ligaments, the medial meniscus provides important contributions to joint stability. With limiting anterior translation of tibia, the meniscus behaves as a secondary stabilizer of the knee joint in this plane [6, 20, 32, 54].
The menisci provide proprioceptive feedback with regard to the position sense of the joint via mechanoreceptors located in their structure. Besides the mechanical support to the stability of the knee joint, by means of proprioceptive reflex arc, the menisci also have functional contributions [21, 32, 54].
The Pathophysiology of Meniscal Injuries
The meniscal tears of young population generally occur as a result of sports injuries. In these patients, the mechanism of injury is mostly exposure to rotational torque to flexed knee under loading conditions. In this patient population, meniscal tears are frequently accompanied by anterior cruciate ligament ruptures or osteochondral injuries [20, 40].
Degenerative meniscal tears become more common with aging. The water content of degenerative meniscal tissue is increased, whereas cell count, collagen, and GAG contents are decreased. Mucinous type of degeneration is observed in the aging meniscus with a decrease in its elasticity and an increase in its friability which makes it vulnerable to tearing. Degenerated meniscus can be torn even with a minor trauma [20, 28].
Meniscal tears are classified as partial or complete tears according to their depth. Complete tears are further classified as stabile and unstable tears. Meniscal tears are also classified according to the type of tear: vertical/longitudinal, radial/transverse, or horizontal/complex tears. Radial tears of the posteromedial part of the medial meniscus are the most common. On the other hand, acute anterior cruciate ligament tears are generally accompanied by vertical longitudinal tears of the lateral meniscus. Degenerative complex tears are most commonly observed in the posterior horn [50].
Healing of the Meniscus
The healing capacity of meniscal tissue is limited. The healing rates of meniscal tears reported to have a range from 63 to 91 % in the literature [10]. Division of meniscal tissue according to its vascularization is relevant with its healing potential. The tears in the red–red zone which has the most abundant blood supply have the highest healing potential. The tears of the red–white zone have relatively good prognosis. Since the white–white zone is avascular, tears in this zone do not heal spontaneously. Even if they are surgically treated, healing would be suboptimal [37, 40].
As is the case in healing processes all around the body, inflammatory cells and mediators should reach the injured zone of the meniscus. Inflammatory cells form fibrin clots in the tears of the highly vascular other zone. This is the first step of healing. The fibrin clot forms a skeleton for the formation of matrix and is also chemotactic for the other mediators which will participate in the healing process. The vessels originating from the perimeniscal capillaries proliferate into the fibrin clot. Meanwhile undifferentiated mesenchymal cells also proliferate. The lesion is filled with a fibrovascular scar tissue which holds the wound edges together and is in continuation with the neighboring meniscal tissue. In time, this tissue maturates to assume a normal fibrocartilage appearance [11, 50]. Nevertheless the newly formed healing tissue is not as durable enough as the healthy meniscal tissue [6].
Based on the understanding of the fact that the more the vascularization is, the better the healing, certain methods began to be applied in order to increase the vascularity of the avascular zone to treat the meniscal tears in this zone. These methods include trephination (forming channels connecting the avascular inner zone to the vascular outer zone), synovial abrasion, pediculated synovial grafts, rasping of the meniscus, exogenous fibrin clot, and bone marrow stimulation techniques [7, 14].
Today studies are being conducted on the utilization of growth factors and cytokines which control the mitogenic behavior of cells and their differentiation and also function as signaling molecules in order to augment the meniscal healing and regeneration. Proinflammatory cytokines such as tumor necrosis factor-alpha (TNF-α) or interleukin-1 (IL-1) were shown to have negative effect on meniscal healing [26, 62]. IL-1 stimulates the synthesis of nitric oxide (NO) and matrix metalloproteinases (MMP) which were shown to decrease the shear strength of repair and suppress cell accumulation and proliferation, therefore adversely affecting the meniscal repair process in vitro [26, 43, 51]. However, the inhibitory effects of IL-1 were shown to be antagonized by dynamic loading in in vitro conditions [43]. On the contrary, the growth factors including insulin-like growth factor (IGF), fibroblast growth factor (FGF), and platelet-derived growth factor (PDGF) have been shown to influence positively the proliferation of the cells of especially the avascular region of the menisci and the formation of new extracellular matrix [63–65]. Nevertheless, for today it seems that the introduction of these basic science findings into the clinical era necessitates further studies.
Utilization of platelet-rich plasma (PRP) has been proposed to augment meniscal healing along with a widespread effort to make it infiltrate into many fields of medicine; but despite the positive results obtained from a few experimental studies, controlled clinical trials favoring its use are lacking [29, 62, 67].
Treatment of Meniscal Tears
The evaluation of the location, type, and length of meniscal tear is essential to decide the proper treatment method. Before intervening with the meniscus, its upper and lower surfaces should be examined thoroughly arthroscopically. Partial tears which do not intersect the whole thickness of the meniscus or tears shorter than 8 mm seldom cause mechanical symptoms and have higher spontaneous healing potentials. Thus, in case of such stable tear, partial meniscectomy is not indicated; instead it should be treated conservatively [6].
Almost until 25–30 years ago, the choice of treatment in meniscal tears was mostly open total meniscectomy. Long-term deleterious effects of total meniscectomies have been shown; this type of treatment increased osteoarthritis risk by 14-fold [55]. In a cadaveric study performed in human knees, tibiofemoral contact mechanics was evaluated following serial meniscectomies. The results of this study revealed that the decrease in the contact area and the increase in the peak contact pressure were similar both in the segmental and total meniscectomized knees. This is because segmental meniscectomy disrupts the continuity of its peripheral part that contains circumferentially oriented collagen fibers resisting hoop stresses [34]. The current concept of treating meniscal tears includes the preservation of meniscal tissue as much as possible. Open procedures are replaced by arthroscopic interventions which are more favorable.
< div class='tao-gold-member'>
Only gold members can continue reading. Log In or Register a > to continue
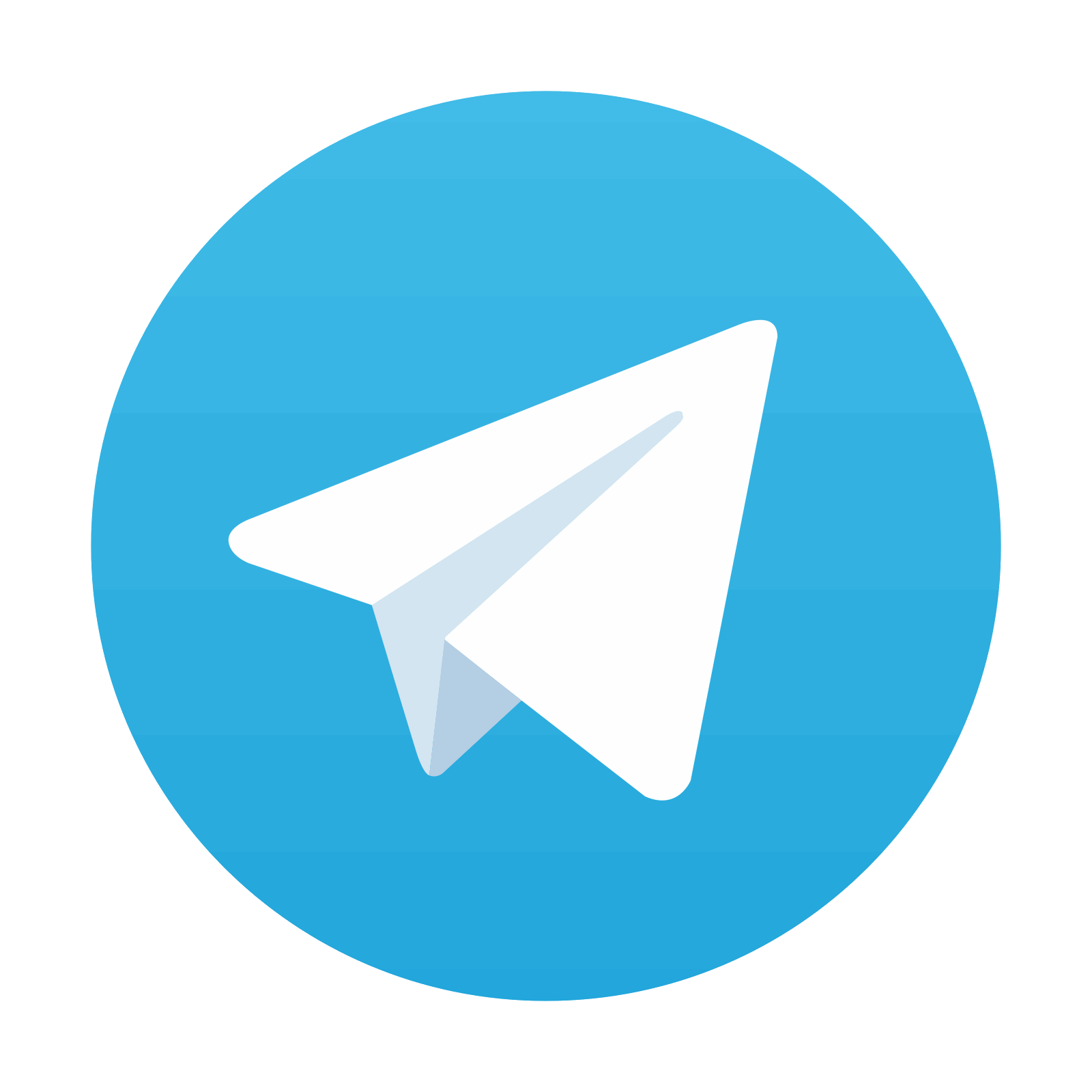
Stay updated, free articles. Join our Telegram channel

Full access? Get Clinical Tree
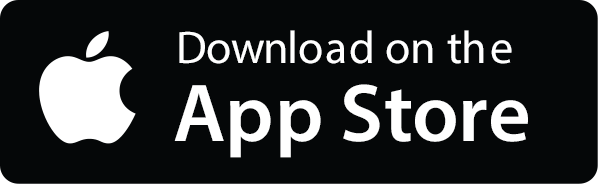
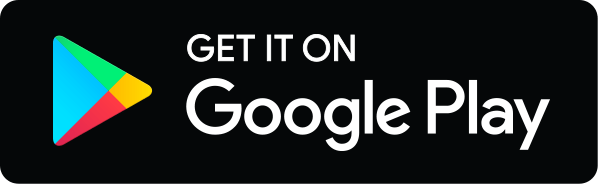