The Pharmacology of Analgesic Agents
David J. Jones
Antonio Hernandez
Analgesia is the relief of pain and is achieved by removing the painful stimulus, blocking the generation of nerve impulses at sensory nerve endings, or reducing signal strength in pain pathways. Analgesia is achieved through the systemic administration of either narcotic or nonnarcotic analgesic agents. Anesthesia is the loss of all sensation with or without the loss of consciousness and can be achieved by blocking the generation of nerve impulses at sensory nerve endings or reducing signal strength in pain pathways. Anesthesia is achieved by either local application or systemic administration of anesthetic agents. Each of these groups of agents has as a therapeutic goal to reduce pain perception, which is the process whereby the brain integrates both sensory and emotional components of nociceptive or neuropathic input.
The process of nociception involves activation of specialized sensory receptors (nociceptors) in nerve endings by noxious insults such as inflammation or injury. A diverse array of chemical mediators, including prostaglandins, leukotrienes, bradykinin, adenosine triphosphate (ATP), histamine, acetylcholine, substance P, and serotonin, either directly or indirectly lower the threshold for activation of nociceptors on primary afferent neurons that terminate in the dorsal horn of the spinal cord. Synapses are formed with spinal neurons that project to supraspinal structures involved in processing and integrating nociceptive inputs resulting in the perception of pain.
A number of different neurotransmitters and neuropeptides, as well as ion channels, have been implicated in playing both direct and indirect roles in modulating signal strength in spinal and supraspinal nociceptive relay circuits (1,2). For example, glutamate released from primary sensory terminals activates both N-methyl D-aspartate (NMDA) and non-NMDA subtypes of receptors; substance P and calcitonin gene—related peptide (CGRP) are colocalized and presumably co-released from terminals of primary afferents; adenosine and protein kinase C appear to play a role in nociceptive signaling; endogenous opioid peptides modulate both ascending (pain perception) and descending (pain control) signaling in nociceptive relay circuits; norepinephrine and serotonin mediate one component of descending control of nociceptive relay circuits; glutamate is also responsible for excitatory conduction in brain pathways mediating nociception and awareness; gamma-aminobutyric acid (GABA) decreases neuronal signal strength by increasing inhibitory conduction in nociceptive pathways; and ion channels (Ca2+, Na+, K+) mediate axonal as well as synaptic conduction in nociceptive pathways resulting in both excitatory and inhibitory transmission. Given the complexity of nociceptive signaling circuits, antidepressants, anticonvulsants, neuroleptics, corticosteroids, NMDA receptor antagonists, central nervous system (CNS) stimulants, and α2 receptor agonists are employed in the management of pain syndromes with varying results. Frequently, these agents are used in combination, especially with the nonnarcotic and narcotic analgesics.
Based on the actions of these various mediators, analgesic agents either block or augment signaling, resulting in a decrease in pain perception. Of the large group of agents noted above, this chapter focuses on nonnarcotic and narcotic analgesic agents, which have as their primary clinical use the management of moderate and severe pain, respectively. Typically, these agents are the first line of treatment for pain syndromes and as such provide a backbone for combination therapy with secondary agents. As such, this chapter is intended to provide an overview of these two groups, rather than a detailed account of individual agents. Dosages and summary of usage are found in Chapters 83 and 65.
NONNARCOTIC ANALGESIC AGENTS
Nonnarcotic analgesics (aspirin, acetaminophen, propionic acid derivatives, and cyclooxygenase II [COX II] inhibitors) provide relief of low- to moderate-intensity pain such as headache, myalgia, arthralgia, and other pains arising from integumental structures. Antipyresis (fever reduction) is also a prominent action of the nonnarcotic analgesics. In contrast to acetaminophen, the salicylates, propionic acid derivatives, and selective COX II inhibitors all exhibit significant anti-inflammatory effects. In general, this group of agents is available over the counter and thus widely available for self-medication and also abuse (3). Consumption is driven by public who have become increasingly aware of managing their own health status as well as aggressive marketing campaigns by manufacturers of these products. As a result adverse reactions and interactions are occurring with increasing frequency, especially in the elderly population.
Salicylates and Propionic Acid Derivatives (Ibuprofen, Naproxen, Ketoprofen)
Acetylsalicylic acid (ASA), also known as aspirin, is absorbed rapidly following oral administration, partly from the stomach
but mostly from the upper small intestine. Appreciable concentrations are found in plasma in less than 30 minutes, with peak blood levels occurring within 1 to 2 hours. The rate and extent of absorption are determined by a number of factors, including the disintegration and dissolution rates of tablets, the pH at the mucosal surface (acid conditions favor salicylate absorption due to increasing the nonionized or uncharged fraction), and gastric emptying time.
but mostly from the upper small intestine. Appreciable concentrations are found in plasma in less than 30 minutes, with peak blood levels occurring within 1 to 2 hours. The rate and extent of absorption are determined by a number of factors, including the disintegration and dissolution rates of tablets, the pH at the mucosal surface (acid conditions favor salicylate absorption due to increasing the nonionized or uncharged fraction), and gastric emptying time.
Aspirin is rapidly hydrolyzed by tissue and blood esterases to acetic acid and salicylic acid (salicylate), with the salicylate being distributed throughout most body tissues. Eighty to ninety percent of the salicylate is bound to plasma proteins, especially albumin. In the liver, salicylate is either conjugated with glycine to form salicyluric acid, conjugated with glucuronic acid to form ester or ether glucuronides, or metabolized (1%) to gentisic acid. The plasma half-life of aspirin is 15 minutes and that of salicylate 2 to 3 hours in low doses and 15 to 30 hours at high or toxic doses (4). This dose-dependent elimination is a result of the limited availability of hepatic glycine to form salicyluric acid and the availability of glucuronic acid to form glucuronides. As a result, at these high concentrations, there is increased unchanged (free) salicylate in the urine. Renal excretion of free salicylate is highly variable and is pH dependent; as little as 5% free salicylate is excreted in acid urine, and up to 85% free salicylate is excreted in alkaline urine (5).
After oral administration, the propionic acid derivatives (the “profens,” e.g., ibuprofen, naproxen, ketoprofen) are rapidly absorbed; peak concentrations vary from 1 to 2 hours (halflife, 2 hours) for ibuprofen, 2 to 4 hours (half-life, 14 hours) for naproxen, and 1 to 2 hours (half-life, 2 hours) for ketoprofen. The propionic acid derivatives are extensively bound (99%) to plasma proteins, usually albumin. The concentration of the propionic acid derivatives may be higher in synovial fluid than in plasma. These agents are extensively metabolized in liver, with more than 90% of an ingested dose of the propionic acid derivatives excreted in the urine as water-soluble metabolites or their conjugates.
The analgesic and anti-inflammatory actions of both the salicylates and propionic acid derivatives are a direct result of their ability to inhibit prostaglandin synthesis. Intermediates in prostaglandin biosynthesis or prostaglandins themselves sensitize pain receptors to mediators such as kinins, histamine, 5-hydroxytryptamine (5-HT), and so forth, which are released as a result of tissue damage. Salicylates and the propionic acid derivatives decrease prostaglandin synthesis through inhibition of prostaglandin synthase I and II (COX I and II) at both central and peripheral sites (6). COX I is constitutive and expressed in most tissues, including those involved with kidney function and gastric cytoprotection. COX II is inducible and activated by cellular mediators released owing to tissue damage and in part is responsible for the inflammatory response. Although both COX I and COX II isoenzymes are inhibited by these agents, there is considerable variation in potency; naproxen is about 20 times more potent than aspirin or ibuprofen. In addition to inhibiting prostaglandin synthesis, both aspirin and the propionic acid derivatives inhibit the migration of polymorphonuclear leukocytes and macrophages into inflammation sites and stabilize lysosomal membranes (thus decreasing the release of mediators from granulocytes, basophils, and mast cells), furthering the antiinflammatory properties of these agents. Aspirin and the propionic acid derivatives effectively penetrate joint fluid to maintain an anti-inflammatory action that exceeds the plasma half-life (7).
Inhibition of prostaglandin synthesis also mediates the antipyretic actions of aspirin and the propionic acid derivatives (8). Bacterial pyrogens stimulate the production of interleukin-1 (IL-1) in macrophages, which in turn stimulates prostaglandin synthesis through activation of COX in or near the hypothalamus (9). The increase in prostaglandin E-2 (PGE2) elevates body temperature by interfering with hypothalamic temperature control mechanisms, which regulate heat production versus loss. By blocking prostaglandin synthesis, aspirin and the propionic acid derivatives facilitate heat loss and reduce elevated body temperature.
The antiplatelet effects of aspirin have received significant notoriety with regard to reducing heart attacks and stroke. Aspirin as ASA before deacetylation in the portal circulation irreversibly acetylates COX in platelets (presystemic inhibition), thus blocking the synthesis of thromboxane A2 and hence the role in platelet aggregation (10). Platelets are particularly sensitive to ASA’s effects because they do not synthesize new COX; the effects of a single dose can last 8 to 10 days (11). The propionic acid derivatives and salicylic acid do not irreversibly inhibit the COX, and thus, it has not been established if these agents produce similar benefits.
The widespread use of aspirin and the propionic acid derivatives provides significant documentation for the adverse side effects of these agents. The most prominent side effect is gastric irritation, and up to 40% of patients taking aspirin have been demonstrated to experience this problem (12). The propionic acid derivatives have significantly less gastric irritation, but this varies from one agent to another and one patient to another. Prostaglandins protect the gastric mucosa by reducing gastric acid production and release. Inhibition of COX I by these agents releases the prostaglandin inhibitory effect on gastric acid production leading to gastric irritation and potential gastric and duodenal ulcers. Coadministration of a prostaglandin analog (misoprostol) can reduce these side effects (13).
Prostaglandins also influence salt and water excretion by regulating renal blood flow and by direct effects on the renal tubules. Because both COX I and COX II are expressed in the kidney, enzyme inhibition can lead to side effects such as fluid retention and electrolyte imbalance (14). Patients with renal disease appear to be most sensitive to the salicylates and propionic acid derivatives such that a reversible decrease in GFR, interstitial nephritis, and nephrotic syndrome may occur. The potential for renal side effects is greater with agents that have a longer half-life, including agents that have greater selectivity for the COX II isoenzyme.
Children with chicken pox or flulike symptoms who take aspirin are up to 25 times more likely to acquire Reye’s syndrome (15). Reye’s syndrome is a deadly disease of children and teenagers occurring in the aftermath of a viral infection (especially the influenza virus) (16). Aspirin administration, in conjunction with viral illnesses, appears to damage hepatic mitochondrial membranes in genetically predisposed individuals, leading to liver injury and encephalopathy. A warning now appears on products containing aspirin in order to avoid the possible complications associated with Reye’s syndrome.
Hypersensitivity reactions to aspirin include asthma (8% to 20% of adult asthmatics) (17), skin rashes, angioedema, and potentially anaphylactoid reactions. Patients with asthma and nasal polyps may experience further sensitivity to aspirin, including bronchospasm and hypotension (18). An additional consideration with both acute and chronic use of aspirin is the prolongation of bleeding time. Although alterations in bleeding time have not been reported to occur with the propionic acid derivatives, hypersensitivity reactions have been reported (19).
Toxicity to aspirin has been well documented, largely because of the general availability of aspirin in household medicine cabinets. Mild aspirin toxicity or salicylism includes headache, dizziness, mental confusion, tinnitus, nausea, and vomiting. These symptoms are dose dependent and resolve with reducing the dosage (20). Severe aspirin toxicity is marked by hyperventilation due to increased CO2 production from salicylate-induced uncoupling of oxidative metabolism in skeletal muscle and direct stimulation of the medullary respiratory center. Respiratory alkalosis, which is compensated for by increased sodium and potassium bicarbonate excretion, leads to severe disturbances in acid-base balance proceeding to convulsions, coma, and, after a period of unconsciousness, death from respiratory failure. Treatment is symptomatic in mild cases of poisoning, but administration of intravenous fluids and correction of acid-base and electrolyte balance is required in more severe cases. Alkalinization of the urine will increase the excretion of free salicylate (21).
Because of their widespread use, drug interactions are not unusual with administration of aspirin and the propionic acid derivatives (22,23). In particular, any other agent that produces gastric irritation potentiates the effects of these drugs on the gastric mucosa. Oral anticoagulants are displaced from plasma protein—binding sites by these agents, which results in an increase in anticoagulant action. Among a number of drugs, combined administration with aspirin can lead to an increase in free versus bound tolbutamide, chlorpropamide, and phenytoin as a result of displacement from plasma protein—binding sites.
COX II Inhibitors
The selective COX II inhibitors have garnered much press lately based on the withdrawal of rofecoxib (Vioxx) from clinical use and the precautions for using celecoxib (Celebrex) (24). Along with valdecoxib (Bextra) and newer compounds such as etoricoxib, these compounds are second-generation nonsteroidal anti-inflammatory agents that demonstrate selectivity for the COX II enzyme (25). Based on selectivity for the COX II enzyme, the therapeutic advantage of these agents is a reduction in gastrointestinal side effects while producing anti-inflammatory effects comparable to the propionic acid derivatives (26,27). The use of the COX II inhibitors is indicated primarily in clinical settings where gastrointestinal side effects preclude the use of aspirin or the propionic acid derivatives (28). However, although the gastrointestinal side effect profile is reduced, contraindications such as allergy, aspirin-induced asthma, and compromised renal function still should be considered. Of greater concern is the risk of cardiovascular and cerebrovascular events with COX II inhibitors which have resulted in the worldwide withdrawal of rofecoxib and significant restrictive labeling for other agents in this class. Such warnings provide even greater concern for potential reactions and interactions when combined with over-the-counter agents such as the propionic acid derivatives administered for long periods of time (29).
Acetaminophen
Acetaminophen has analgesic and antipyretic actions, which are comparable to therapeutic doses of aspirin and the propionic acid derivatives. The mechanism whereby acetaminophen produces these actions is unclear because it demonstrates weak inhibition of COX I and COX II and also does not suppress inflammation. Studies suggest that the weak inhibition of COX and hence lack of anti-inflammatory action may be due to the presence of high concentrations of peroxides found at inflammatory sites which inactivate the acetaminophen molecule (30). The presence of a COX III isoenzyme that acetaminophen selectively inhibits or the production of reactive metabolites by the peroxidase function of COX II may further explain the lack of anti-inflammatory actions of acetaminophen (31,32).
Acetaminophen is rapidly and almost completely absorbed from the gastrointestinal tract. The concentration in plasma reaches a peak in 30 to 60 minutes, with a half-life in plasma of 2 to 3 hours. Unlike aspirin and the propionic acid derivatives, acetaminophen is relatively uniformly distributed throughout most body fluids; binding of the drug to plasma proteins is 20% to 50% (33). Practically, no acetaminophen is excreted unchanged, and the bulk is excreted after hepatic conjugation with glucuronate (about 60%) or sulfate (35%).
Acetaminophen is usually well tolerated though skin rash and other allergic reactions exhibited by localized areas of erythema or urticaria occur occasionally. The most serious toxic effect of acute overdosage of acetaminophen is a dosedependent, potentially fatal hepatic necrosis (34). Studies have demonstrated that as little as 4 g/d of acetaminophen (eight extra-strength tablets) can produce severe liver injury.
When toxic doses are ingested, the ability to glucuronidate acetaminophen is exceeded, shifting metabolism to the microsomal enzyme system (microsomal enzyme oxidizing system)-dependent N-hydroxylation to form N-acetyl-p-benzo-quinoneimine (NABQ) (30). NABQ usually reacts with
sulfhydryl groups in glutathione to form an inactive product. Because of the limited amount of hepatic glutathione, excess NABQ reacts with sulfhydryl groups in hepatic proteins, producing cellular damage and hepatic necrosis.
sulfhydryl groups in glutathione to form an inactive product. Because of the limited amount of hepatic glutathione, excess NABQ reacts with sulfhydryl groups in hepatic proteins, producing cellular damage and hepatic necrosis.
Early signs of liver toxicity include nausea, vomiting, and abdominal pain. Clinical signs of liver damage may become apparent within 2 to 4 days of ingestion of toxic doses (35). Regular consumption of ethanol can induce hepatic cytochrome P-450, leading to increased oxidative metabolism of acetaminophen to NABQ and lowering the threshold for acetaminophen-induced liver damage (36). Alcoholics may also exhibit hepatic glutathione depletion, which can further increase the severity of liver injury.
Treatment of overdosage with acetaminophen requires vigorous supportive therapy, including procedures to limit continued absorption of the drug such as induction of vomiting or gastric lavage, followed by oral administration of activated charcoal. Hemodialysis, if it can be initiated within the first 12 hours, has been advocated for all patients. Administration of sulfhydryl compounds, such as N
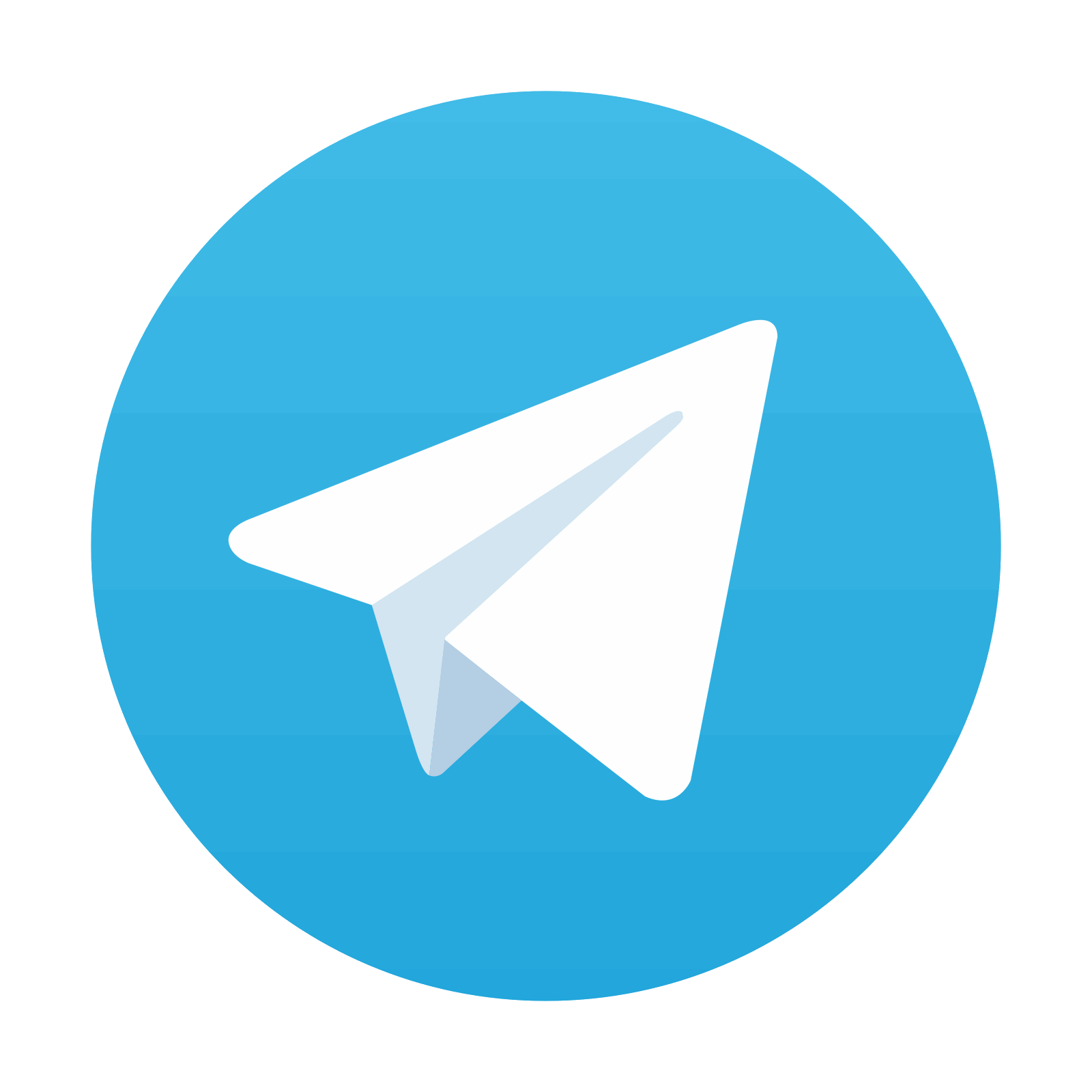
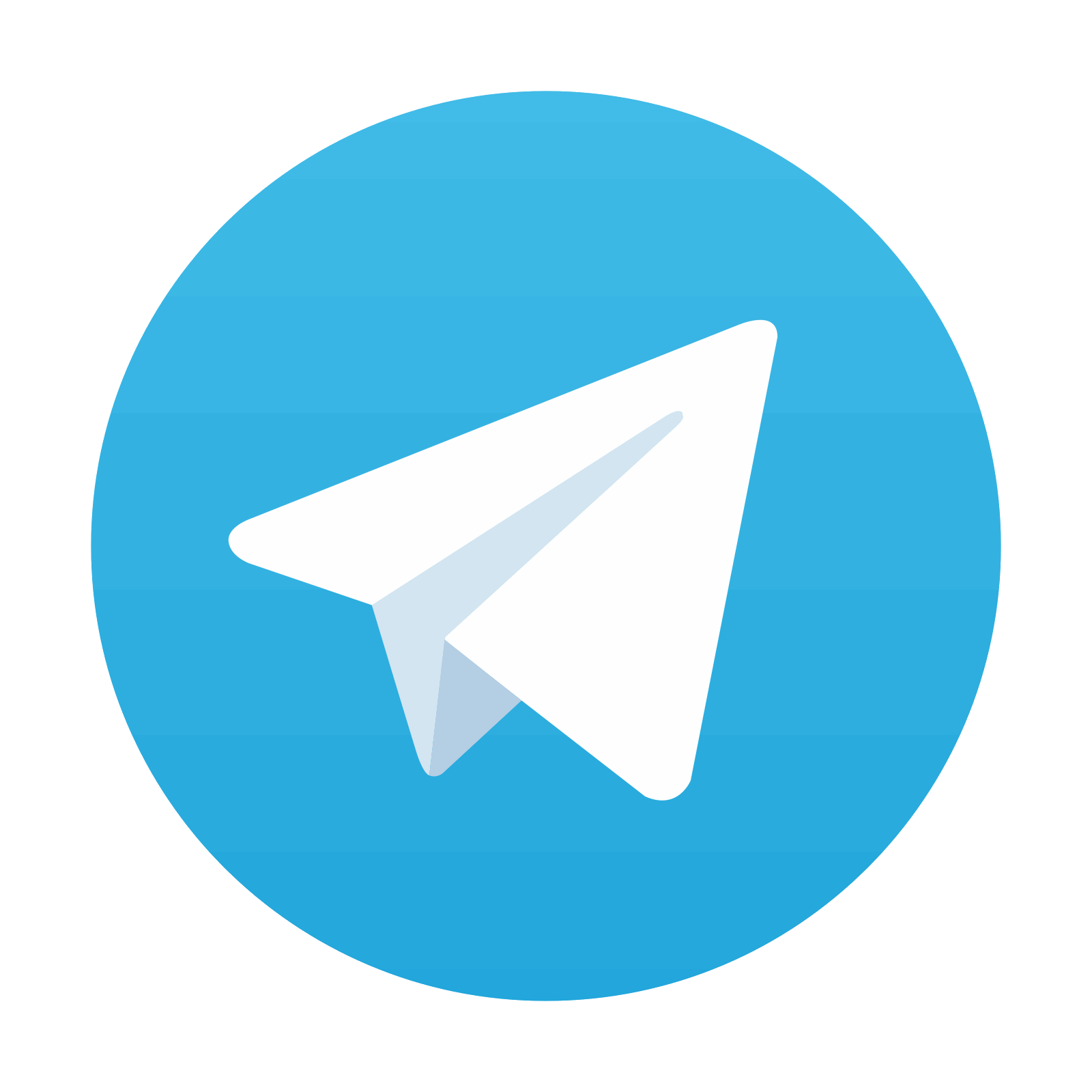
Stay updated, free articles. Join our Telegram channel

Full access? Get Clinical Tree
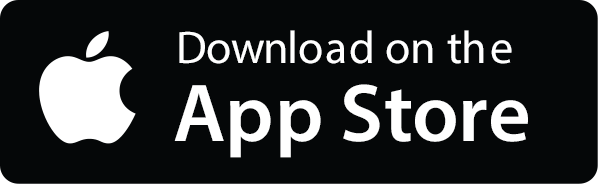
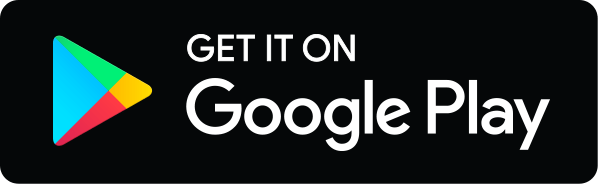
