The Lower Extremity
Perry L. Schoenecker
Margaret M. Rich
J. Eric Gordon
ROTATIONAL VARIATION
Definition.
Rotational profiles vary widely among healthy children (1, 2 and 3). Differences in appearance related to foot position during walking or running are most often just that, differences, not pathologic conditions. Foot position during walking is described by the direction of the foot relative to the body’s line of progression during the gait cycle (internal, external, or neutral). This is torsion. It results from the summation of several factors that include version of the bones, capsular pliability, and muscle control (1, 3, 4 and 5). Version is tilt or inclination within a bone, such as the relation of the femoral head/neck to the shaft of the femur. Contracture of a joint capsule may restrict rotation. Similarly, capsular laxity may allow a greater than normal arc of motion. Arthrosis or incongruity may also restrict motion. The balance between opposing muscle groups is also a determinant of foot position and may introduce a significant, dynamic component to the rotational profile. Age is another important variable because version, soft-tissue pliability, and muscle coordination change as the child matures (1, 2, 3, 4, 5, 6, 7 and 8).
Assessment.
Assessment of rotational alignment includes static and dynamic components. The static examination describes the available range of rotational motion. The dynamic examination displays the effect of various torsional forces at play during the walking cycle (Fig. 27-1). The static examination should be performed on a firm examining table, with the child in comfortable, loose clothing such as shorts or a diaper. Rotation is best assessed with the child in the prone position, keeping the pelvis flat and level on the examination table (3, 4 and 5). Flexion of the knee to 90 degrees allows the leg to be used like a goniometer relative to the thigh. Young children often will not allow examination other than on a parent’s lap. This is usually adequate although not as controlled as in the prone position. The arc of rotation may be more generous if measured with the hip flexed as in a sitting position (5).
Rotation of the leg laterally or medially is used to assess the degree of available hip rotation. When there is a greater degree of internal or medial rotation (outward movement of the leg in the prone position) than external or lateral rotation, a toed-in gait is more likely to be observed. Similarly, if there is greater external rotation than internal rotation, the gait pattern is usually toed-out. A greater ability to rotate the thigh internally rather than externally is frequently
referred to as anteversion. It should correctly be called antetorsion because hip rotation is the combined effect of version of the femur, joint mobility, and muscle function (1, 3, 5). True femoral torsion can be accurately assessed by noting the position of the leg while palpating the greater trochanter laterally.
referred to as anteversion. It should correctly be called antetorsion because hip rotation is the combined effect of version of the femur, joint mobility, and muscle function (1, 3, 5). True femoral torsion can be accurately assessed by noting the position of the leg while palpating the greater trochanter laterally.
Medial hip rotation is generally greater in girls than in boys (Fig. 27-2). Variability is greater in younger children. Static medial hip rotation averages 40 degrees in infants, but can range from 10 to 60 degrees. It increases slightly by the age of 10 years and then decreases gradually in adulthood. Lateral hip rotation is greater than medial rotation in infants; it averages 65 degrees (range, 45 to 90 degrees), compared to children over the age of 10 years who average 40 degrees (range, 20 to 55 degrees) (1, 2 and 3, 5, 9, 10). It increases slightly in adults.
Observation of the alignment of the axis of the hindfoot relative to the thigh, which is held in neutral rotation, determines the thigh-foot angle (TFA), as shown in Figure 27-1B. This relation also describes the contribution of the
leg segment or the degree of tibial torsion. Foot deformity, which may contribute to rotational abnormalities, can also be assessed easily in this position. Forefoot adduction or abduction and hindfoot varus or valgus is noted. Many young children have significant rotational laxity through the knee. Internal and external rotation of the tibia with the knee flexed demonstrates the degree of knee joint laxity. This may contribute to variation in foot position. The average TFA is 5 degrees internal in infants (range, -30 to +20 degrees) and increases to 10 degrees external by 8 years of age (range, -5 to +30 degrees) (1, 2 and 3, 5). TFA changes very little after 12 years of age.
leg segment or the degree of tibial torsion. Foot deformity, which may contribute to rotational abnormalities, can also be assessed easily in this position. Forefoot adduction or abduction and hindfoot varus or valgus is noted. Many young children have significant rotational laxity through the knee. Internal and external rotation of the tibia with the knee flexed demonstrates the degree of knee joint laxity. This may contribute to variation in foot position. The average TFA is 5 degrees internal in infants (range, -30 to +20 degrees) and increases to 10 degrees external by 8 years of age (range, -5 to +30 degrees) (1, 2 and 3, 5). TFA changes very little after 12 years of age.
Correlation between the static examination and the dynamic examination is important. The child’s walking pattern should be observed in an area large enough to allow comfortable and safe walking and running. The child should be able to walk alone (i.e., not holding someone’s hand). It is often helpful to observe the child in shoes as well as barefoot. Variations are common with change in speed or direction of walking. Foot and knee position should be observed over several cycles of gait. The relation of foot position to an imaginary line along the path being walked describes the foot-progression angle (FPA). Variability is considerable in children up to 5 years of age. An out-toed position predominates in older children. Once a mature gait pattern is established, usually by 5 years of age, FPA changes very little (1, 2, 3, 4, 5 and 6, 9, 10).
The child’s rotational profile includes the contribution of each of these components to the gait pattern. The FPA, TFA, and position of the knee relative to the body (femoral torsion), all contribute to the sum of rotational factors. Each area needs to be assessed along with its contribution, either positive (internal rotation) or negative (external rotation), to the overall gait pattern. Those children whose rotational profiles are beyond two standard deviations of the mean are considered abnormal according to Staheli’s criteria (3, 4) (Fig. 27-2).
Differential Diagnosis.
Infrequently, pathologic conditions will cause a rotational abnormality. Residual foot deformities,
disorders of the hip, and neuromuscular diseases are the most common causes of pathologic in-toeing or out-toeing. In-toeing may be caused by residual foot deformity from metatarsus adductus, clubfoot, or skewfoot (1, 4, 5). These conditions are discussed in detail elsewhere in this text. In-toeing, which is only apparent during swing phase, may be the result of overpull of the posterior tibial tendon, often seen in spastic hemiplegia (11, 12).
disorders of the hip, and neuromuscular diseases are the most common causes of pathologic in-toeing or out-toeing. In-toeing may be caused by residual foot deformity from metatarsus adductus, clubfoot, or skewfoot (1, 4, 5). These conditions are discussed in detail elsewhere in this text. In-toeing, which is only apparent during swing phase, may be the result of overpull of the posterior tibial tendon, often seen in spastic hemiplegia (11, 12).
Femoral antetorsion is often seen in spastic diplegia or quadriplegia. This may be a combination of excess femoral anteversion with contracture of the adductor and medial hamstring muscles (4, 5, 13). Excess valgus and pronation of the foot, which contribute to an out-toed foot progression, may also be seen (13). For some children with spasticity, extremes in rotational posture are a compensatory mechanism for limited hip, knee, or ankle motion. The combination of excess internal femoral rotation and external tibial rotation (malicious malalignment) (13, 14) is often observed in children with spasticity. Children with diplegia and quadriplegia often have heel cord tightness. To maintain foot contact with the floor, the calcaneus tends to rotate laterally beneath the talus, which allows the talar head to drop plantarward, producing a valgus deformity (15). If the peroneal tendons are also spastic, the forefoot will also be pulled into an abducted position creating a planovalgus deformity. If the child is unable to clear the foot during swing phase, the foot is repeatedly dragged along the floor, adding to the external torsional force applied to the foot. This produces a malalignment, with the foot externally rotated from the planovalgus deformity and the knee internally rotated from femoral antetorsion.
Pathologic out-toeing may result from the severe pes planovalgus often associated with external tibial torsion (16) (Fig. 27-3). This may be secondary to tarsal coalition, but may also be seen in adolescents with rigid flat feet without a coalition. It is unclear whether the out-toed position is an adaptation to a rigid flat foot, or whether the lack of foot flexibility promotes the development of external rotation. A rigid deformity may occur with or without a tarsal coalition. The combination of femoral retrotorsion, external tibial torsion, and pes planovalgus can also be seen, particularly in large adolescents, which produces a striking out-toed gait. Slipped capital femoral epiphysis (SCFE) should be considered in a differential diagnosis of out-toeing, particularly when the deviation is asymmetric or of recent onset (4, 17). Hip dysplasia can alter rotation, but its effect is highly variable (7). Asymmetric hip rotation may be apparent upon examination, but is not reliable in detecting hip dysplasia. In such instances, further evaluation with radiographic hip examination is warranted (3, 4). Coxa vara may also present as an out-toed gait.
Radiographic Evaluation.
It is recommended that any child who presents to an orthopaedic surgeon with concerns of a gait abnormality have an anteroposterior (AP) pelvis radiograph. Children older than 8 years with a recent change in gait or with complaints of hip or knee pain should also have a frog lateral radiograph of the hips (3, 4, 17). A cross-table lateral film should also be obtained to detect a minimally displaced SCFE. Although the incidence of otherwise occult pathology
is low, the consequence of a missed diagnosis, such as hip dysplasia or an SCFE, is significant for the child.
is low, the consequence of a missed diagnosis, such as hip dysplasia or an SCFE, is significant for the child.
It is not necessary to obtain serial radiographs of the hips or lower extremities to follow the course of normal rotational development (4, 5). Special views to quantify version of the femur or tibia are not indicated in the routine evaluation of rotation (3, 4 and 5). Three-dimensional imaging studies [computed tomography (CT) and magnetic resonance imaging (MRI)] are typically not indicated in the assessment or follow-up of rotational variations, but are more accurate in quantifying rotation than clinical or biplane radiography (18).
Natural History.
Most children brought in for concerns of intoeing or out-toeing are normal. Rotational profiles are highly variable, particularly in toddlers who have not mastered the basic skills needed for normal walking, which includes just about every child younger than 2 years and many of those who are 2 to 5 years of age (1, 2, 6, 10). Internal and external rotational variations should be considered just that, variations of normal, not pathologic conditions. The natural history of rotational variations is gradual normalization, usually accomplished by 6 to 7 years of age. There are no conclusive studies to show that any nonsurgical intervention speeds up or assures the normalization of gait (4, 5, 19, 20).
Internal tibial torsion is more common than external tibial torsion in toddlers (4, 5) (Fig. 27-4). It is often associated with physiologic bowlegs and decreases 1 to 2 years after the resolution of the bowing. Occasionally, it will persist into preadolescence. External tibial torsion is less common, but is more likely to persist through adolescence (4, 5).
The assessment of hip range of motion in newborns and infants is highly variable (2, 3, 5, 7). Most healthy infants have an external rotation contracture about the hip, which is likely a result of intrauterine positioning. This may not fully resolve until they become established walkers at 18 to 24 months of age. At that age, an in-toed gait may be more apparent. Lateral hip rotation gradually decreases as medial rotation increases. Paradoxically, anteversion in the femoral neck typically decreases from 30 degrees (range, 15 to 50 degrees) at birth to 20 degrees (range, 10 to 35 degrees) by 10 years of age (1, 2, 3, 4 and 5, 7, 8). A decrease in anteversion of the femoral neck would be expected to produce greater external rotation of the hip; however, changes in muscle balance and hip capsule pliability appear to have greater influence on gait. By 8 years of age, a child who toes in while walking, but has at least 20 degrees of hip external rotation, is within normal limits as defined by the mean plus or minus two standard deviations. Similarly, one who toes out while walking, but has at least 20 degrees of hip internal rotation, has motion within a normal range (4, 5, 10).
![]() FIGURE 27-4. A: Internal tibial torsion is often seen in toddlers in association with physiologic bowlegs and results in an in-toed gait. B: The TFA is best assessed in the prone position. |
Variations in rotation have not been linked directly to the risk of degenerative joint disease (9, 10, 21, 22, 23, 24, 25, 26 and 27). Several authors have tried to correlate the degree of femoral anteversion with the presence of osteoarthritis of the hip using postmortem studies or by preoperative CT scan. Most studies have shown a similar measure of anteversion in hips with and without arthritic changes (22, 23, 24, 25 and 26). One study measured anteversion in hips of patients undergoing proximal femoral osteotomy or total hip replacement. Patients with bilateral disease had an average 9 degrees greater anteversion than patients with healthy hips. Patients with unilateral arthritis averaged 4 degrees more anteversion in the arthritic hip than in the healthy hip (26). Some authors have demonstrated a relation of anteversion with degenerative changes in the knee, presumably from increased shear loads (27). Hip pain is not a typical complaint in children with increased femoral anteversion alone (1). Anterior knee pain may be associated with increased medial rotation of the femur, but not generally with patellofemoral changes (23, 27). Although some recent publications have suggested that torsional variations may increase the risk of osteoarthritis, anatomic studies have not conclusively shown a causal
relation between femoral anteversion and osteoarthritis. A subset of patients exists in whom the limits of tolerance in hip or knee range of motion is exceeded and for whom the risk for early degenerative changes may be increased (27, 28). In these patients, the alteration in rotation places increased stress across the hip or knee, which can promote the development of osteoarthritic changes. The precise limit of tolerance remains undefined. However, the presence of hip or knee pain should be considered as indications for osteotomy (29).
relation between femoral anteversion and osteoarthritis. A subset of patients exists in whom the limits of tolerance in hip or knee range of motion is exceeded and for whom the risk for early degenerative changes may be increased (27, 28). In these patients, the alteration in rotation places increased stress across the hip or knee, which can promote the development of osteoarthritic changes. The precise limit of tolerance remains undefined. However, the presence of hip or knee pain should be considered as indications for osteotomy (29).
Although the presence of excessive hip external rotation may augment posterior shear loads, an increased incidence of slipped epiphysis has not been found in patients with femoral retroversion without other contributory factors being present (17). Athletic ability does not correlate with the position assumed during normal walking, although some activities may be hindered by rotational variations, particularly hip or tibial external rotation (30, 31 and 32). High-performance sprinters, however, tend to adopt a toedin position during running regardless of their walking style (32). Functional impairments such as tripping, clumsiness, or lack of running ability, although often attributed to rotational differences, have not been shown to correlate with rotational profile (30).
There are some children with such extreme variation in rotation that the appearance of their gait is unacceptable to them or their parents. The natural history of rotational variation, the lack of evidence for musculoskeletal sequelae, and the absence of objective functional disability must be kept in mind and the family educated in this regard (1, 3, 4 and 5). The natural history of rotational variation should be clearly communicated to the parents and understood by them prior to any recommendation for active treatment.
Treatment.
The natural history of rotational variations is gradual normalization. No treatment is necessary in most children who present with concerns of in-toeing or out-toeing. The use of shoe modifications, orthotics, or positioning devices is ineffective (5, 17). Although these measures are not harmful, there is no data to show any positive effect. Their use reinforces the errant notion that in-toeing or out-toeing is an abnormal condition or disorder that requires treatment. The cost of orthopaedic shoes and orthotics can be considerable. Musclestrengthening exercises or activities may diminish the dynamic component that produces variation in gait; however, no studies have addressed this specific topic. Treatment options for foot and ankle abnormalities are covered in Chapter 29.
Rotational osteotomy may be considered for those otherwise healthy children who have persistent rotational abnormality into later childhood and adolescence and find the appearance of their gait or their function unacceptable (4, 5, 27, 33, 34). Gait may appear less awkward following osteotomy. Patients and their parents often express satisfaction with the change in appearance following osteotomy. Improvement in function is variable and less predictable. Those with intoeing typically note less tripping. Those with out-toeing are more likely to notice an improvement in running ability.
Pain, although rarely a complaint, may be improved following surgical treatment (27). Most often, this occurs in patients with malicious malalignment or the combination of femoral internal rotation with tibial external rotation (27, 35) (Fig. 27-5). These patients may experience knee symptoms preoperatively, likely related to the increased shear forces through the knee. This combination of rotational abnormalities has been associated with some risk of patellofemoral arthritis (36, 37).
The authors preferred technique for correcting rotational deformities of the tibia/fibula is a supramalleolar osteotomy of the tibia and at times also of the fibula (Figs. 27-6, 27-7, 27-8 and 27-9). A distal correcting osteotomy has less potential for serious neurovascular complication than does proximal tibial osteotomy (33, 38). The tibia should be cut 2 to 3 cm above the physis and parallel to the ankle. It is essential that the plane of the osteotomy be perpendicular to the longitudinal axis of the tibia (rotation of the distal on the proximal fragment through an oblique cut may introduce problematic angular deformity). For rotational deformities >30 degrees, the addition of a fibular osteotomy facilitates the ability to rotate the tibia without inadvertent translation of the distal fragment relative to the proximal tibia. The degree of correction is determined by aligning the foot and ankle, such that the second toe is in line with the tibial tubercle and the center of the patella. As the foot is dorsiflexed and knee bent, the foot should remain in line with the lateral thigh. This aligns the flexion-extension axes of the ankle, knee, and hip.
For fixation in younger patients, the authors prefer crossed K-wires supplemented with a long leg, bent knee cast. Two or three stainless steel wires of appropriate size are inserted; one from proximal medial to distal lateral and avoiding crossing the medial growth plate of the distal tibia and the second from distal medial to proximal lateral. A third wire is used if rotational stability does not seem adequate with two wires. A long leg bent knee cast is used for 6 weeks. Healing is usually sufficient at that time for pin removal and application of a short-leg weight-bearing cast for an additional 4 weeks.
In older (>8 to 9 years of age) and/or larger patients, a small-fragment T-plate or cloverleaf plate can be used for tibial fixation. The T-plate is placed anteriorly just proximal to the distal tibial growth plate. If a cloverleaf plate is used, it can be placed medially. A short-leg cast is worn for 5 weeks. Toetouch weight bearing is allowed. The short-leg cast is changed to a moon boot at 5 to 6 weeks and progressive weight bearing allowed. Plate removal later may be necessitated by the subcutaneous position of the plate.
Correction of excess femoral internal or external rotation is obtained by equalizing the degree of internal versus external rotation. The amount to be rotated can be estimated during the prone examination, comparing internal and external hip rotation, preoperatively (5). Femoral osteotomy may be performed proximally, distally, or midshaft depending on the surgeon’s preference and the type of fixation to be used (34, 39) (Figs. 27-10, 27-11, 27-12, 27-13, 27-14 and 27-15). Proximal femoral osteotomy is preferred if there is also varus, valgus, flexion, or extension deformity of the hip. Similarly, angular deformities about the knee are better addressed by a distal femoral osteotomy. A standard lateral approach to the femur is used, whether proximal or distal. In proximal osteotomy, a pediatric or adolescent blade plate is used for fixation. If a distal femoral osteotomy is performed in a skeletally immature patient, a small straight compression plate or 95-degree condylar (adolescent) blade plate may be used for fixation. The use of cast or orthotic immobilization is at the discretion of the surgeon. Alternatively, a medial approach can be used along with K-wire fixation (34). This latter technique should be reserved for smaller children and must be supplemented with a spica cast. The desired amount of rotational correction is typically that which achieves an equal amount of internal and external rotation.
![]() FIGURE 27-7. The incision for the tibial osteotomy is made along the anterior border of the tibia, extending proximally from the ankle crease 4 to 5 cm. |
Femoral Rotation Osteotomy Using an Intramedullary Nail (Figs. 27-10, 27-11, 27-12, 27-13, 27-14 and 27-15)
In children 9 years of age or older, intramedullary (IM) fixation can be used for either the tibia or the femur (39, 40). Locking screws or similar mechanisms are needed proximal and distal to the osteotomy because the osteotomy has no intrinsic stability. In the skeletally immature and in those with a relatively narrow medullary canal, the osteotomy is generally performed by a limited open technique. The proximal femur entry site is made lateral and distal to the tip of the greater trochanter. Great care is taken to avoid any dissection (including penetration) on the medial side of the trochanter. A recent modification in femoral nail design (proximally angulated 15 degrees) facilitates correct nail placement. This lateral trochanter entry site is necessary to avoid injury to the medial portion of the trochanteric growth plate and injury to the terminal branches of the medial circumflex artery in the trochanteric fossa. Neither coxa valga nor avascular necrosis has occurred in our early experience with this approach (40). Fixation with an IM rod does not allow for any concomitant correction of varus, valgus, flexion, or extension. However, IM fixation does allow for early weight bearing, a particular advantage if bilateral procedures are performed.
Increased femoral internal rotation and tibial external rotation may coexist, producing a rotational malalignment (35, 41) (Fig. 27-5). Knee pain, which is usually nonspecific, may be present. Patellar maltracking usually is not present. Because the deformities are complementary, foot progression may be normal, but the static examination will demonstrate the abnormality. Combined osteotomy of the femur and tibia may be necessary to correct symptomatic malrotation (4, 35, 41). Femoral rotation is corrected as the first step of the procedure. The foot is then aligned with a tibial osteotomy completing the correction. Satisfactory correction aligns the planes of motion of the hip, knee, and ankle. Correction of bilateral deformities can be performed as staged unilateral procedures 6 to 12 months apart.
Complications.
The risks and complications of in-toeing and out-toeing are related to its treatment, not its presence (3, 4 and 5, 17, 19, 20, 30). Because most patients who have surgical alteration of their rotation do so to effect a change in the appearance of their gait, the surgical procedure must accomplish the desired change in rotation. Functional change is noted in some children, but not consistently.
Complications of rotational osteotomy are the same as for other osteotomies. Problems related to nonunion, infection, blood loss, joint stiffness, scarring, and anesthesia are the most serious (33, 34 and 35, 38, 42). Distal tibial osteotomy has less risk of compartment syndrome and peroneal nerve injury than proximal osteotomy. Whether performed proximally or distally, the use of a blade plate for fixation of a femoral osteotomy may inadvertently produce undesirable frontal or sagittal plane deformity. Similarly, inadvertent deformity can be produced in the distal tibia if an oblique osteotomy is made, rather than a transverse cut, in performing a distal tibial osteotomy. The authors avoid crossing the distal tibial physis when using K-wires to fix a supramalleolar tibial osteotomy. We have observed distal tibial physeal growth arrest and deformity following K-wire penetration of the medial tibial physis. Asymmetric growth arrest can occur from periosteal stripping and injury to the lateral distal femoral physis. Injury to the greater trochanteric apophysis can produce valgus deformity in the proximal femur.
Angular deformity may be less problematic when a locked IM fixation device is used; however, adjustments in position can be difficult once the rod is locked. Use of a lateral entry point helps minimize the risk of avascular necrosis to the femoral head or disruption of normal growth of the proximal femur (40, 42). Recent changes in pediatric IM nail design permit safer entry through the lateral aspect of the greater trochanter, minimizing both of these complications (40). In the skeletally immature patient, use of this lateral entry site is imperative.
PHYSIOLOGIC BOWING AND GENU VARUM
Physiologic Bowing
Definition.
Infants and children frequently present to the orthopaedic surgeon for evaluation of bowing (43). Often, these children are early walkers, achieving independent ambulation before their first birthday (44, 45). The normal knee alignment at birth is 10 to 15 degrees of varus, which remodels to a neutral femoral-tibial alignment at approximately 14 months of age (1, 46, 47 and 48) (Fig. 27-16). Levine and Drennan (49) have defined physiologic bowing, radiographically, as more than 10 degrees of bilateral femoral-tibial varus is noted after the age of 18 months. These children will often have associated internal tibial torsion which results in a characteristic bowed, intoed gait.
Clinical Features.
Parents are concerned with both the cosmetic appearance of the bowing, in-toeing deformity, and the associated problems of excessive tripping. A family history of bowing is common (45, 50). Examination reveals bowing of both the lower extremities with in-toeing (Fig. 27-17). Although the bowing deformity is bilateral, it may be asymmetric. Despite their bowlegged, toed-in gait, these young children are characteristically very agile walkers. The child should be observed walking, both toward and away from the examiner, to assess the severity of the dynamic varus and associated internal rotation deformity. Internal rotation of the extremity permits contact of the foot with the floor as the child stands. This also maintains the body center over the midline as the child walks. The presence of a lateral thrust should be noted. This is a brief,
dynamic, lateral, knee movement that occurs during single-leg stance. It can represent a lateral subluxation or shifting of the femur on the depressed medial tibia or lateral ligamentous laxity. A lateral thrust is characteristic of pathologic bowing. The amount of varus deformity of the lower extremity can be measured with a goniometer, with the knees extended and patellae facing forward. The distance between the knees when the patient stands with the ankles together is noted. Photographs are helpful in documenting the deformity. Angular and rotational alignment and joint range of motion of the entire lower extremity are assessed. Medial-lateral knee joint laxity is not usually present in physiologic bowing.
dynamic, lateral, knee movement that occurs during single-leg stance. It can represent a lateral subluxation or shifting of the femur on the depressed medial tibia or lateral ligamentous laxity. A lateral thrust is characteristic of pathologic bowing. The amount of varus deformity of the lower extremity can be measured with a goniometer, with the knees extended and patellae facing forward. The distance between the knees when the patient stands with the ankles together is noted. Photographs are helpful in documenting the deformity. Angular and rotational alignment and joint range of motion of the entire lower extremity are assessed. Medial-lateral knee joint laxity is not usually present in physiologic bowing.
![]() FIGURE 27-17. Examination of the child with physiologic bowing shows symmetric bowing throughout the tibia and internal tibial torsion which is often more noticeable with walking. |
In younger children, those <18 months of age, radiographs can document the degree and location of the varus deformity, but usually will not distinguish between physiologic bowing and early Blount disease. Radiographs are an essential part of the evaluation in the older child (over 18 months of age). Radiographs are also indicated for those with more pronounced deformities (clinical femoral-tibial angle >20 degrees), when a lateral thrust is observed, if the child is of short stature (below fifth percentile), or if a metabolic bone disease is suspected (Fig. 27-18). The radiograph is taken with the patient standing, if possible, and the patellae pointing straight ahead. The relative degree of varus deformity is noted by observing the shaft-to-shaft angle of the femur and tibia (51, 52). More importantly, the distribution of bowing deformity is noted (53). When physiologic, the bowing occurs throughout the distal femur, proximal tibia, and distal tibia. In contrast, in early Blount disease, the varus deformity is more focally limited to the proximal tibia.
Differential Diagnosis.
The differential diagnosis of bowing in the young child includes physiologic bowing and pathologic bowing (Table 27.1). Pathologic bowing occurs in infantile tibia vara (Blount disease), metabolic bone disease, skeletal dysplasias (Fig. 27-18B-D), and focal fibrocartilaginous dysplasia. The clinical and radiographic features
associated with metabolic bone disease or skeletal dysplasia readily differentiate these pathologic conditions from physiologic bowing. Most often, the necessary differentiation is between physiologic bowing and infantile Blount disease. If the bowing is physiologic, the entire lower extremity will appear to be bowed. If the varus results from a relatively greater deformity of the proximal tibia, infantile tibia vara or Blount disease may be present (54, 55 and 56). Children with either physiologic bowing or Blount disease usually are early walkers and typically present for evaluation at 15 to 18 months of age. Often, there is a positive family history of bowing deformity (in siblings, parents, uncles, and aunts) (45, 54). Physiologic bowing and Blount disease are two points within the same spectrum, with Blount disease being the pathologic result of unresolved infantile bowing (51, 54). Frequently, one extremity will have physiologic bowing, with Blount disease affecting the contralateral tibia.
associated with metabolic bone disease or skeletal dysplasia readily differentiate these pathologic conditions from physiologic bowing. Most often, the necessary differentiation is between physiologic bowing and infantile Blount disease. If the bowing is physiologic, the entire lower extremity will appear to be bowed. If the varus results from a relatively greater deformity of the proximal tibia, infantile tibia vara or Blount disease may be present (54, 55 and 56). Children with either physiologic bowing or Blount disease usually are early walkers and typically present for evaluation at 15 to 18 months of age. Often, there is a positive family history of bowing deformity (in siblings, parents, uncles, and aunts) (45, 54). Physiologic bowing and Blount disease are two points within the same spectrum, with Blount disease being the pathologic result of unresolved infantile bowing (51, 54). Frequently, one extremity will have physiologic bowing, with Blount disease affecting the contralateral tibia.
TABLE 27.1 Differential Diagnosis of Bowed Legs | ||||||||||||||||||||
---|---|---|---|---|---|---|---|---|---|---|---|---|---|---|---|---|---|---|---|---|
|
Radiographic Evaluation.
Radiographic distinction between physiologic bowing and Blount disease is not obvious in very young children. The Langenskiöld changes, diagnostic of Blount disease, are not always distinct before 2 to 3 years of age (47, 50, 51, 53, 56). Measuring the metaphyseal-diaphyseal (MD) angle of both the proximal tibia and distal femur helps to identify the location and relative severity of varus deformity (51, 55, 56). Although an absolute MD angle is not diagnostic, it does serve as an early guide in differentiating Langenskiöld stage I infantile Blount disease from physiologic bowing (44, 49, 50, 53) (Fig. 27-19). Measurement can be affected by limb position (56). A study of the proximal tibial MD angle in patients with bowing (physiologic bowing or Blount disease) identified two distinct populations with considerable overlap (54, 55) (Fig. 27-20). On the basis of this study by Feldman and Schoenecker, when the MD angle is 10 degrees or less, there is a 95% probability that the diagnosis is physiologic bowing. Conversely, if the MD angle is 16 degrees or more, there is a 95% probability that Blount disease is present. Bowen et al. (52) noted that all children with a tibial MD angle >16 degrees showed progression of the varus deformity. For those patients with an MD angle between 10 and 16 degrees, follow-up for at least 1 to 2 years is necessary to determine whether the bowing resolves (physiologic bowing) or progresses (Blount disease).
The ratio of the distal femoral MD angle relative to that of the proximal tibia can be helpful in differentiating physiologic bowing from early Blount disease. This is measured on the AP lower extremity x-ray film by constructing a distal femoral MD angle similar to that measured in the proximal tibia. This angle represents the contribution of varus in the distal femur to the overall measure of femoral-tibial varus in the limb. The distal femoral MD angle is divided by the proximal tibial MD angle. This quotient represents the proportion of varus found in the femur relative to the tibia. A ratio of >1 suggests that the bowing is physiologic, that is the femur contributes as much as the proximal tibia to the varus, and resolution is expected to occur (55). A ratio of <1 indicates that the bowing is predominantly within the tibia and is more likely to evolve into Blount disease.
Bowing can be associated with metabolic bone disease or skeletal dysplasia (Fig. 27-18C,D). Rickets [usually X-linked hypophosphatemic (XLH) rickets] is the most common metabolic bone disease to present as a bowed leg deformity in a toddler (57, 58). Nutritional rickets should be suspected if the child was breast-fed and did not receive supplemental vitamin D. Infants presenting with rickets are short; the measured
height is often below the tenth percentile. Radiographs of infants with bowing deformity secondary to rickets should not be mistaken for physiologic bowing. In patients with XLH, the physis appears abnormally wide, and the metaphysis is flared and curves like a trumpet around the physis. Similar changes are found within all growth plates. Bone density will be diminished overall, with thinning of the diaphyseal and metaphyseal cortices. The severity of changes in bone morphology and the degree of osteomalacia is variable. The diagnosis of XLH is made by analyzing calcium and phosphate in serum and in urine (57, 58). Patients suspected of having rickets should be referred to an endocrinologist for a thorough metabolic workup.
height is often below the tenth percentile. Radiographs of infants with bowing deformity secondary to rickets should not be mistaken for physiologic bowing. In patients with XLH, the physis appears abnormally wide, and the metaphysis is flared and curves like a trumpet around the physis. Similar changes are found within all growth plates. Bone density will be diminished overall, with thinning of the diaphyseal and metaphyseal cortices. The severity of changes in bone morphology and the degree of osteomalacia is variable. The diagnosis of XLH is made by analyzing calcium and phosphate in serum and in urine (57, 58). Patients suspected of having rickets should be referred to an endocrinologist for a thorough metabolic workup.
The child who presents with bowing deformity in association with a skeletal dysplasia will be short, typically below the 5th percentile. The radiographic changes for each skeletal dysplasia varies with the site of involvement which may be principally epiphyseal, physeal, metaphyseal, or diaphyseal or involve multiple sites and may also involve the spine. Achondroplasia, the most common of the skeletal dysplasias, typically presents with bowing deformity. On a standing AP x-ray, characteristic findings include a knee centered varus deformity with an elongated fibula. Patients with pseudoachondroplasia may present with a varus deformity in association with ligamentous laxity. Metaphyseal chondrodysplasia (Schmid’s or McKusick’s type) typically presents with persistent bowing and short stature in an otherwise normal-appearing child (Fig. 27-16D). While the radiographic changes about the physis and metaphysis include flaring and widening of the medial metaphysis, the changes are distinct from those of osteomalacia and bone density will appear normal (see Chapter 8).
Focal fibrocartilaginous dysplasia is a very rare, but progressive, unilateral, focal deformity that can occur either in the proximal tibial metaphysis or in the distal femoral metaphysis (59, 60, 61, 62, 63, 64 and 65). The clinical presentation is similar to unilateral infantile Blount disease. The lesion consists of a focus of fibroblastic and cartilaginous tissue, usually below the insertion of the pes anserinus on the tibia (Fig. 27-21). This produces an acute angulation below the metaphysis. The diagnosis is made radiographically. A characteristic indentation is noted in the medial cortex at the junction of the metaphysis and diaphysis, and a focal varus deformity is associated with it. The lesion is radiolucent and often well circumscribed, often with a rim of reactive bone. The physis and epiphysis are normal. An identical process has also been observed in a corresponding location in the distal medial femur (63). Some authors have reported spontaneous improvement in angulation. Simple curettage is recommended for persistent lesions or those in unusual locations (64).
Natural History of Physiologic Bowing.
Parents should be informed that spontaneous correction of physiologic bowing is anticipated as predicted by Salenius and Vankka and Sabharwal et al. (1, 46, 47) (Fig. 27-16). Bowing, although present in infants, is often not noticed until the child begins standing. The bowing resolves, typically by 2 years of age, and physiologic valgus develops between 3 and 4 years of age (Fig. 27-22). Nonoperative treatment (orthotics, shoe modification, or nighttime splinting) is both unnecessary and ineffective. In mild deformities, the bowing and its associated internal tibial torsion predictably resolve; follow-up visits may not be necessary. For those patients with more pronounced or persistent deformities, follow-up visits are scheduled at 3- to 6-month intervals. Resolution or progression of the varus that occurs with growth can be documented by subsequent physical examination. Serial photos can be compared with the initial image to determine the degree of resolution or progression of the bowing deformity. Follow-up standing AP radiographs should be obtained if the varus does not appear improved on clinical appearance or by serial goniometric measurement. Uncommonly, varus may persist into late childhood without progressive physeal changes. Varus which does not resolve warrants continued follow-up and may require treatment before skeletal maturity with growth modulation.
Infantile Blount Disease
Definition.
In 1937, Walter Blount (65) published his classic review of tibia vara or osteochondrosis deformans of the proximal tibia, noting the progressive course of both the varus
clinical deformity and the correlative radiographic pathology. The distal femur is typically normal, but occasionally a valgus deformity will develop later (52, 53, 66). Both the clinical and radiographic pathology described by Blount and histopathologic changes described by Langenskiöld (44, 65, 67, 68, 69 and 70) (Fig. 27-19) were felt to be secondary to a disruption of normal growth of cartilage and bone. These progressive changes are caused by excessive focal pressure on the proximal medial tibial growth plate and adjacent bone from chronic abnormal weight bearing. Avascular necrosis of bone in Blount disease has not been observed (69). Progression of this developmental, pathologic tibia vara can be corrected with treatment (65, 67, 68, 69 and 70).
clinical deformity and the correlative radiographic pathology. The distal femur is typically normal, but occasionally a valgus deformity will develop later (52, 53, 66). Both the clinical and radiographic pathology described by Blount and histopathologic changes described by Langenskiöld (44, 65, 67, 68, 69 and 70) (Fig. 27-19) were felt to be secondary to a disruption of normal growth of cartilage and bone. These progressive changes are caused by excessive focal pressure on the proximal medial tibial growth plate and adjacent bone from chronic abnormal weight bearing. Avascular necrosis of bone in Blount disease has not been observed (69). Progression of this developmental, pathologic tibia vara can be corrected with treatment (65, 67, 68, 69 and 70).
Etiology.
Early on, infants with physiologic bowed leg deformity cannot be clearly distinguished from those with infantile Blount disease. Like physiologic bowing, infantile Blount disease is usually bilateral (48, 53, 54). When unilateral infantile Blount disease is noted, often the contralateral extremity is bowed physiologically. These children typically are early walkers (<10 months of age) and often are overweight (>95th percentile). As with physiologic bowing, there may be a family history of bowing deformity.
Cook et al. (71), using finite element analysis, calculated that the compressive force resultant from weight-bearing stresses on a bowed leg was sufficient to produce a disturbance in growth. Obesity increases the potential for growth disturbance. In Blount disease, the focal pathologic changes in the proximal medial tibial growth plate cause varus to progress. This chronic growth disturbance results from disorganization of the physis and the osteochondrosis of the medial proximal tibial physis and adjacent epiphysis and metaphysis as described by Blount (44, 48, 65, 67, 68, 69 and 70, 72). The proximal medial tibia fails to grow normally, and tibia vara of increasing severity develops. This results in shortening of the extremity and, if left untreated, ultimately results in depression of the medial tibial condyle and intra-articular deformity.
Pathoanatomy and Radiographic Features.
In 1952, Langenskiöld identified six distinct radiographic stages of development of the proximal tibia depicting the natural progression of untreated infantile Blount disease (44, 70) (Fig. 27-19). The
stage and the age at which it occurs have prognostic significance (48, 50, 51 and 52, 73). In stages I and II, the irregular metaphyseal ossification changes are often indistinguishable from physiologic bowing. These changes may be reversible. Clear-cut stage I or stage II changes of Blount disease are typically not apparent until the patient is 2 to 21/2 years of age. Stage III shows definite deformity in the proximal tibial physis, often with some fragmentation. Stage IV lesions can be associated with early bar formation across the deformed physis, as it assumes a vertical orientation (44, 70). These physeal bars are often difficult to detect. There is profound disruption of the physeal cartilage and abnormal growth in the adjacent bone as stage V develops, usually in children older than 8 years. Eventually, this process results in severe depression of the articular surface and stage VI disease (74). Consistent positioning of the lower extremities is important to detect subtle changes in the physis. Standing AP radiographs with the patellae facing forward are recommended for serial evaluations.
stage and the age at which it occurs have prognostic significance (48, 50, 51 and 52, 73). In stages I and II, the irregular metaphyseal ossification changes are often indistinguishable from physiologic bowing. These changes may be reversible. Clear-cut stage I or stage II changes of Blount disease are typically not apparent until the patient is 2 to 21/2 years of age. Stage III shows definite deformity in the proximal tibial physis, often with some fragmentation. Stage IV lesions can be associated with early bar formation across the deformed physis, as it assumes a vertical orientation (44, 70). These physeal bars are often difficult to detect. There is profound disruption of the physeal cartilage and abnormal growth in the adjacent bone as stage V develops, usually in children older than 8 years. Eventually, this process results in severe depression of the articular surface and stage VI disease (74). Consistent positioning of the lower extremities is important to detect subtle changes in the physis. Standing AP radiographs with the patellae facing forward are recommended for serial evaluations.
In North America, advanced Langenskiöld stages often occur at a much younger age than in Finland. All of Langenskiöld’s patients were Caucasians from Finland, whereas a large proportion of patients in the United States are African American. North American children typically experience more rapid progression with more severe, irreversible changes at an earlier age than their European counterparts. Stage IV changes are often seen in 4- to 5-year-old children in the United States compared to 7- to 8-year-old children in Finland. The greater incidence and severity of disease has been attributed to a higher proportion of overweight children in North America (80).
The distal femur in infantile Blount disease is usually normal. Although distal femoral varus does not occur in infantile Blount disease, valgus does occasionally occur in children with more advanced tibial changes. It may be a response to the asymmetric load across the knee, allowing relative overgrowth of the distal medial femur (52, 66). More often, the valgus appearance of the femur is a consequence of severe tibial bowing and relative abduction of the hip, which creates the illusion of valgus at the distal femur.
Nonoperative Treatment.
Brace treatment should be considered either in all patients <3 years of age with unilateral Blount disease changes (Langenskiöld stages I, II) or in patients older than 18 months who have persistent bowing and risk factors for Blount disease. A tibial MD angle of >16 degrees is a radiographic sign of significant risk for Blount disease (49, 54, 55). A sign of relative risk is an MD angle between 10 and 16 degrees along with the clinical appearance of a varus deformity or progression of varus. Additional risk factors include obesity, ligamentous instability, or the presence of a lateral thrust, any of which may potentiate a varus deformity (51, 54). Improvement in the tibial MD angle should be apparent within 12 months of brace treatment.
Studies have demonstrated that brace treatment can correct both the varus deformity and the pathologic proximal-medial tibial growth disturbance (75, 76 and 77). In these three reports, 79 extremities were treated using a brace. The best results were obtained with unilateral deformity, where brace treatment was successful in 22 of 23 patients. In contrast, brace treatment was less successful for treating bilateral deformities, with only 18 of 28 patients noted to be successfully treated. Compliance was much more difficult to achieve for the child with bilateral deformity, as is understandable. It is also less effective in obese patients. Bracing should not be initiated after 3 years of age, nor should brace treatment be continued if Langenskiöld stage III changes develop (75, 76 and 77).
We have successfully treated unilateral Blount disease with a modified knee-ankle-foot orthosis (KAFO). The knee is held in extension with a single medial upright KAFO. Thigh and calf cuffs and a varus-correcting lateral knee pad provide three-point fixation. It is worn for 23 of 24 hours (50, 51, 75, 76 and 77) (Fig. 27.23). The locked KAFO counteracts the pathologic medial compressive forces, allowing resumption of more normal growth and correction of the genu varum. In those patients who are compliant, the clinical appearance of bowing typically improves over the ensuing months; however, the pathologic radiographic changes at the proximal medial tibial metaphysis, physis, and epiphysis are slower to remodel. Brace treatment is continued until the bony changes in the proximal medial tibia resolve; typically, this takes 11/2 to 2 years of brace treatment (75, 76 and 77). Brace treatment will be successful if by 4 years of age the mechanical axis of the lower extremity passes through the center of the knee and the depression of the medial epiphysis resolves. The radiographic appearance of the medial epiphysis and metaphysis should normalize by 5 years of age. If satisfactory correction does not occur, surgical correction should be recommended.
Operative Treatment.
Children older than 21/2 to 3 years with worsening stage II or evolving stage III Blount disease, who are either noncompliant or not good candidates for brace treatment because of obesity or bilateral involvement, should be treated surgically with proximal tibia and fibular varus-correcting osteotomy. Alternatively, surgical treatment with growth modulation has been shown to be effective in selected cases. Early surgery to realign the leg by 4 to 5 years of age is necessary to prevent progression to stage IV disease, when the early formation of a physeal bar can occur. Surgical treatment unloads the medial compartment of the knee and facilitates the growth of the proximal medial physis. Restoration of normal growth in the medial tibial physis is less likely to occur if surgery is delayed such as until 5 years of age (78).
Young patients and/or those with less than stage IV Langenskiold changes may be considered for growth modulation treatment (79). Growth modulation is best accomplished in this age patient with the placement of a small-fragment (typically two-hole) plate extraperiosteally across the proximal lateral tibial physis. The plate is secured by cortical screws. This construct impedes lateral physeal growth. Over time, correction will occur. Frequent follow-up visits are essential to monitor correction. This is assessed by measuring the mechanical axis of the involved lower extremity. With correction of the varus deformity, the mechanical axis will shift from its previous location medial to the center of the knee to a more lateral position. A mechanical axis slightly lateral to the center of the knee joint is desirable. The medial growth plate often
continues to grow more slowly than the lateral growth plate. Slight overcorrection is desirable to compensate for the often occurring and variable differential growth of the proximal tibial physis. Continued follow-up is mandatory to assure that correction of varus is maintained and/or to address recurrent deformity. Repeat application of the plate may be needed to correct mild recurrent deformity.
continues to grow more slowly than the lateral growth plate. Slight overcorrection is desirable to compensate for the often occurring and variable differential growth of the proximal tibial physis. Continued follow-up is mandatory to assure that correction of varus is maintained and/or to address recurrent deformity. Repeat application of the plate may be needed to correct mild recurrent deformity.
Osteotomy is preferred for more severe deformity or if close, reliable follow up cannot be assured (Figs. 27-24, 27-25, 27-26, 27-27, 27-28, 27-29, 27-30, 27-31 and 27-32). The proximal tibial osteotomy is performed with attention to both the known inherent risks and the need for obtaining adequate correction of the deformity (78, 80, 81). The fibula is osteotomized through a separate lateral incision, taking care to avoid injury to the deep motor branches of the peroneal nerve (38, 82). The tibial osteotomy can be accomplished in a variety of methods (48, 51, 68, 78, 82, 83, 84, 85 and 86). A straight transverse osteotomy allows for necessary correction of frontal, sagittal, and rotational deformity. The fragments are stabilized with smooth K-wires (Fig. 27-24C,D). Alternatively, Price et al. have effectively utilized monolateral external fixation to stabilize the tibial osteotomy (86). A slight “overcorrection” into valgus with or without translation of the distal fragment laterally is desirable (48, 50, 51, 82). This places the mechanical axis of the leg within the lateral compartment of the knee, unloading the medial proximal tibia. The mechanical axis of the leg can be assessed intraoperatively using the bovie cord. The cord is stretched from the center of the hip and across the center of the ankle with the leg resting on a radiolucent table. The leg should not be held, but simply allowed to rest on the table. The wire within the bovie cord, which is visible on the C-arm, is used to verify the position of the mechanical axis (Fig. 27-24E,F). This simple technique provides a reproducible method to verify that the mechanical axis has actually been transferred lateral to the center of the knee joint (82). Alternatively, an intraoperative AP x-ray film of the entire lower extremity can be taken to assess the correction obtained. If a unilateral deformity is present, clinical comparison to the normal extremity is helpful in determining whether adequate correction has been obtained. A subcutaneous fasciotomy of the anterior compartment is performed prior to wound closure. Suction drains are routinely used.
Postoperatively, the extremity is immobilized in a non-weight-bearing, long-leg, bent-knee cast. Alternatively, a spica cast is used for the child with relatively short, fat extremities. On occasion, a KAFO is used following cast removal if the preoperative deformity was severe and associated with ligamentous laxity. Following corrective osteotomy, the pathologic changes in the proximal medial tibia must be carefully monitored. These bony changes are not always reversible, and further progression in their development may be associated with a recurrence of deformity (44, 67, 69, 70). It is essential to document that valgus alignment has been obtained and maintained by the use of long cassette radiographs (hip to floor) and comparison of serial examinations until skeletal maturity (Fig. 27-24G).
Complications.
Neurovascular complications are the principle risks associated with a proximal tibial osteotomy (38, 80, 87, 88 and 89). A careful subperiosteal exposure minimizes direct nerve and vessel trauma. Prophylactic limited fasciotomy and the use of drains help avoid increased compartment pressure (38, 82, 89). If a compartment syndrome is suspected postoperatively, immediate fasciotomy should be performed. Based on our past clinical experience, an acute traction or impingement injury to the peroneal nerve may also occur (38). Prompt surgical release of any peroneal nerve compression has typically resulted in a satisfactory outcome.
Worsening Varus Deformity and Physeal Bar Formation.
Recurrent or worsening varus deformity with persistent pathologic changes can occur despite restorative osteotomy at a young age (44, 50, 51). The risk for recurrent deformity is present in some children as early as Langenskiöld stage III and is much more common in those with stage IV disease, marked obesity (>95th percentile weight), or ligamentous laxity (48, 50, 51) (Fig. 27-33). If patients present with advanced pathologic changes or with recurrent deformity following brace or surgical treatment, an MRI or CT scan should be utilized to search for the presence of a physeal bar within the distorted proximal medial tibia. Identification of a bar may be difficult because of the serpentine course of this abnormal physis, which takes on a vertical slope as the deformity worsens. Early bridging across the physis typically occurs at the inferior aspect of the vertical limb of the distorted medial physis (Fig. 27-33B).
Progressive varus deformity may occur without an identifiable bony bar (Langenskiöld III or early IV) (48). This occurs rather subtly because of a decreased growth rate of the proximal medial tibial physis relative to the lateral physis and can occur any time prior to skeletal maturity. As the physis transitions from a normal horizontal orientation to a more vertical position, this differential growth becomes more evident. Careful observation is needed to detect this change early in the course of treatment. Premature closure of the medial tibial physis frequently occurs because of persistent deficient proximal medial physeal growth. If this occurs, the varus deformity can often be controlled with growth modulation, using an eight-plate or hemiepiphyseal staples (48, 79, 90, 91). If additional tibial physeal growth is anticipated, the internal fixation is removed after a slight overcorrection is obtained. Careful follow-up including radiographic assessment of recurrent varus deformity and/or leg-length inequality are mandatory until skeletal maturity is reached.
Operative Treatment.
If an osseous bar is identified, restorative surgery is indicated. Left untreated, progressive bar formation will result in complete arrest of the medial physis (Langenskiöld stage VI). Physeal bar resection in conjunction with a varus-correcting osteotomy is most effective if the patient is young (under 10 years), the bar is relatively small, and the patient is not excessively overweight (48, 51, 70, 92, 93) (see Figs. 27-37, 27-38 and 27-39). The proximal tibia is approached through an anteromedial longitudinal incision and the physeal bar is resected first. Often the bar is not discrete, but is a collection of several small punctate foci of bone that coalesce and function as a tether. The medial edge of the normal physis is often difficult to locate. Excision of the bony bridge is done cautiously, preserving as much normal physeal tissue as possible. However, resection must be complete so that an intact physeal line coursing 180 degrees from the posteromedial to the anteromedial cortical edge of the tibia is visible. The C-arm can be helpful in monitoring the procedure. Methylmethacrylate (Cranioplast) is used to fill the void to inhibit the formation of a recurrent osseous tether. Additionally, the existing varus deformity should be surgically corrected, typically with varus-correcting osteotomies of the tibia and fibula (Fig. 27-33C). Alternatively, milder varus deformity can be corrected with growth modulation utilizing either a temporary lateral hemiepiphyseal staple or eightplate in conjunction with the medial epiphysiolysis.
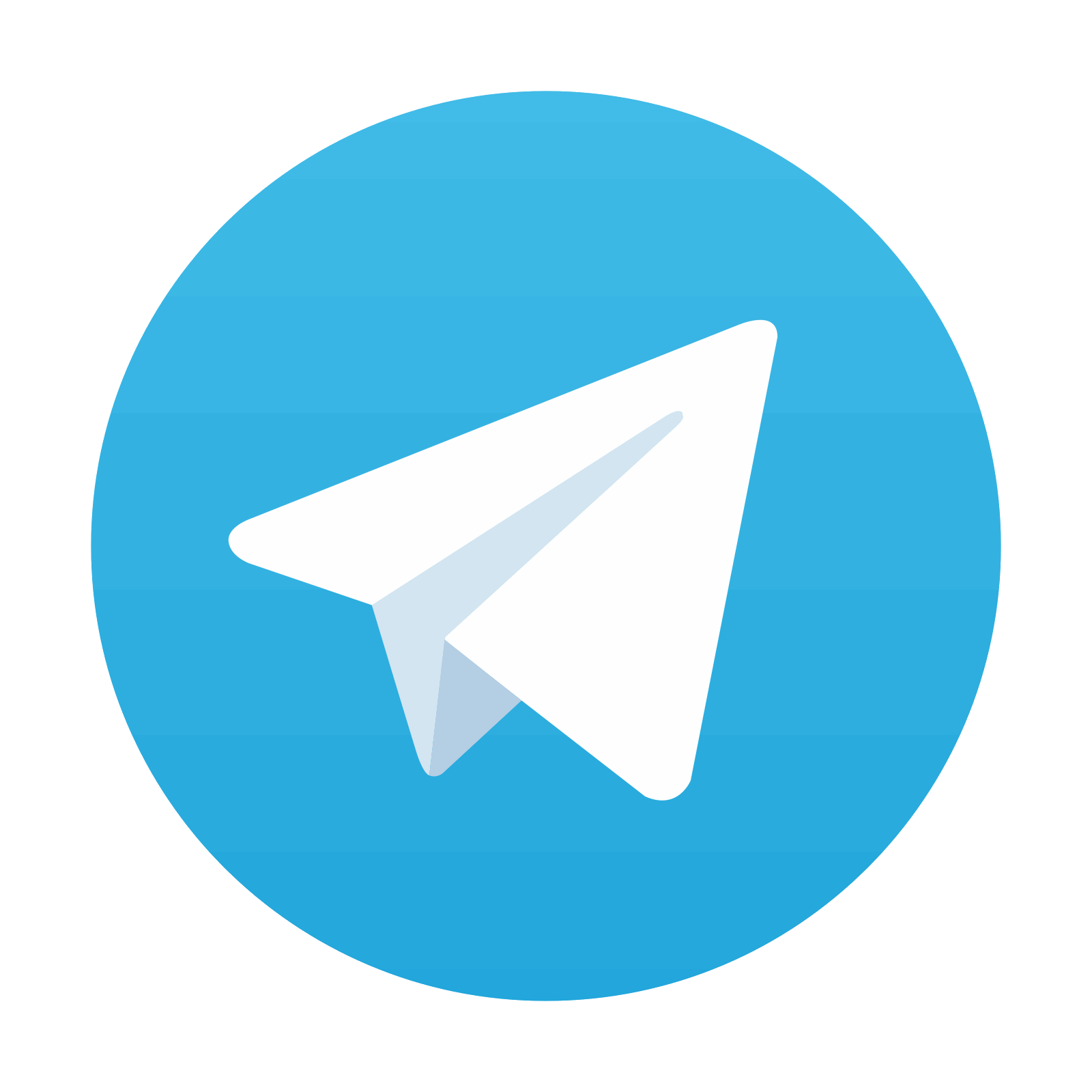
Stay updated, free articles. Join our Telegram channel

Full access? Get Clinical Tree
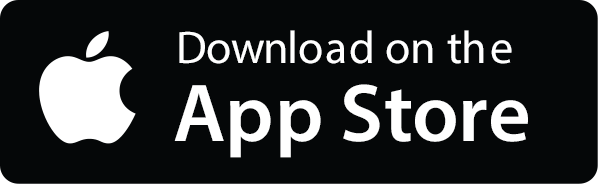
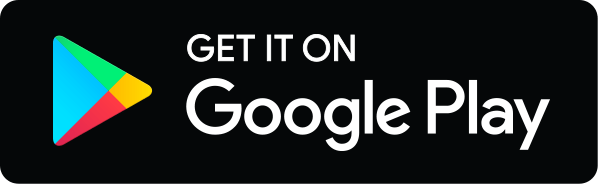
