This means, for example, that towards the ‘late’ stages of rehabilitation it does not necessarily matter whether a rugby player has sustained an injured ACL, PCL or lateral meniscus – if the player wishes to return to competitive rugby the knee still has to perform in a multi-directional agility-biased sport with the same frequent rapid deceleration-acceleration cycles, as well as tolerating high impact forces with every foot-strike when running and jumping or as a result of collisions with opponents or the ground, regardless of the initial type of knee injury. As such, a major premise of this chapter’s overall rehabilitation philosophy is that range-of-motion (ROM) restrictions and rehabilitation strength training modifications specific to a unique knee injury type and its associated healing constraints are typically discontinued in ≤ 12 weeks post-injury or post-surgery, after which all tibiofemoral soft tissue injuries can often then be guided through rehabilitation according to the same progression criteria towards the same sport-specific functional drills and goals. Table 21.2 shows typical knee mechanisms of injury and common clinical diagnostic tests.
Table 21.2 Mechanism of injury, incidence of injury and common clinical diagnostic tests
Early stage rehabilitation
The early rehabilitation phase following injury or surgical reconstruction of the knee ligaments or menisci is initially concerned with the resolution of inflammation, the reduction and removal of swelling, and regaining full activation of the inhibited muscles, principally the quadriceps. Use of the rest, ice, compression, elevation (RICE) principle and how it helps in the resolution of inflammation has been discussed elsewhere within this book. With all knee injuries it is critical that the injured structures are allowed time to recover from the trauma they have received as a result of the initial injury or as a result of any surgical intervention. It is important that in all but the most minor of injuries, the patient’s level of weight-bearing during gait and functional activities in the first 72 hours post-injury is ideally pain-free or at least causes minimal pain. The use of crutches and partial weight bearing (PWB) is often critical to achieving this. In simplest terms, if the knee-injured patient presents with any antalgic gait or ‘limp’, crutches should be used for as long as is necessary to temporarily unload and protect sensitised healing tissues. Crutches should not be discontinued until a patient can demonstrate full active knee extension ROM (i.e. there is no ‘quadriceps lag’). Table 21.3 illustrates more injury-specific weight-bearing guidelines. Minimising quadriceps inhibition and atrophy is of critical importance in the management of any knee injury, and this is covered below.
Table 21.3 Weight-bearing, range-of-motion and exercise rehabilitation guidelines
Knee rehabilitation concerns and concepts
There are several clinical concerns and concepts regarding the safe and expedient rehabilitation of any knee-injured patient, especially in the initial rehabilitation period (≤ 12 weeks post-injury/post-surgery). Specific questions that address these clinical concerns and concepts include:
- How to manage quadriceps muscle atrophy and overcome its inhibition?
- Should a surgical reconstruction be performed?
- Should a knee brace be used during rehabilitation?
- What are the rehabilitation strength training and joint loading considerations when treating the knee-injured patient?
- Does a surgical reconstruction prevent knee osteoarthrosis (OA)?
Quadriceps inhibition and muscle atrophy
Persistent quadriceps weakness is associated specifically with ACL injury (Palmieri-Smith et al. 2008) but also knee injuries in general, and presents a major rehabilitation challenge for patients and clinicians alike. Quadriceps strength deficits can still easily exceed 20% at 6 months after ACL-R, a time when many athletes are cleared to return to activity (Keays et al. 2007). The magnitude of quadriceps weakness can lessen with time, but most research suggests that quadriceps strength deficits following knee joint injury persist for many months or years following injury or surgery (Shelbourne and Nitz 1990; Snyder-Mackler et al. 1995; Natri et al. 1996; Holder-Powell and Rutherford 1999; Risberg et al. 1999b, 2007; Mikkelsen et al. 2000; Feller and Webster 2003), illustrating the long-term nature of this problem. As the quadriceps is critical to dynamic joint stability, weakness of this muscle group is related to poor functional outcomes and may contribute to the early onset of knee OA (Palmieri-Smith et al. 2008).
There is evidence to suggest that arthrogenic muscle inhibition (AMI) and perhaps muscle atrophy are primarily responsible for the decrements in quadriceps strength following knee injury (Palmieri-Smith et al. 2008). As such, AMI in the form of voluntary activation failure is hypothesised to result from reflex activity in which altered sensory information from the injured knee joint leads to a diminished motor drive to its surrounding muscles. If clinicians can be effective in combating AMI early in the rehabilitation process, they will minimise the resulting strength deficits and diminish muscle atrophy, which should lead to a more complete more effective rehabilitation, and likely will result in quicker patient recovery and return to sport. The question is how can this muscle inhibition be addressed? In order to remove AMI, it will need an alteration in the inhibitory processes causing it. This can be achieved by one of two strategies: altering sensory feedback that signals something is wrong with the knee, or modifying the motor drive to the muscles.
Removing arthrogenic muscle inhibition: Altering sensory feedback
Arthrogenic muscle inhibition can be caused by increased abnormal sensory activity caused by joint effusion, or by a lack of normal sensory feedback due to the loss of mechanoreceptors subsequent to an ACL or other ligamentous rupture. So, one approach to removing AMI would be blocking/modifying the sensory signals responsible for initiating the inhibitory process. This might be achieved by removing abnormal sensory stimuli, minimising pain, or sending signals to the central nervous system (CNS) that may modify any pre-synaptic pathways contributing to AMI.
The most obvious method to reduce a joint effusion is aspiration of the joint. Though not without risks, it has been shown to be effective in significantly reducing AMI (Fahrer et al. 1988). Other modalities can also be used to reduce effusion such as compression, electrical muscle stimulation and elevation, but their effectiveness in reducing AMI is yet to be evaluated. Application of ice has been shown to decrease nerve conductance velocity and so slow the discharge of mechanoreceptors. Also following the application of ice, quadriceps activity has been found to be facilitated (Hopkins et al. 2002). Hopkins et al. (2002) also found that the application of transcutaneous electrical nerve stimulation (TENS) decreased AMI through the reduction of pre-synaptic inhibition. Stokes et al. (1985) found the effect of TENS was maximised when used whilst the patient exercised.
Removing arthrogenic muscle inhibition: Improving motor drive
Rather than blocking or modifying abnormal sensory feedback, an alternative approach to targeting AMI is to activate the inhibited motorneurons. As AMI prevents active recruitment of the quadriceps, electrical muscle stimulation (EMS) would allow for direct recruitment of motorneurons. Moreover, EMS has also been shown to preferentially activate type II muscle fibres compared with voluntary training of a similar intensity (Binder-Macleod et al. 1995). Research has also shown quadriceps EMS in combination with active quadriceps exercise has a superior effect than exercise or EMS alone (Snyder-Mackler et al. 1991).
Electromyography (EMG) and other forms of biofeedback may also be a useful adjunct to active exercise in overcoming AMI. Though the evidence is limited, Maitland et al. (1999) present a case study showing positive findings. Eccentric exercises have the potential to overload the muscle in a manner greater than that of concentric exercise. Gelber et al (2000) found positive results with the addition of specific eccentric exercise programmes, and that by using a graduated programme any issue with delayed onset muscle soreness (DOMS) was avoided.
Should a surgical reconstruction be performed
When an isolated ACL injury is present there is a great deal of evidence demonstrating that it is possible for an ACL-deficient (ACL-D) patient to return to high level physical activities following sufficient exercise rehabilitation (Herrington 2004; Neuman et al. 2008). However, there is no single subjective or objective predictor as to whether an ACL-D patient should or should not undergo reconstructive surgery (Herrington and Fowler 2006), and the amount of anterior knee laxity measured by anterior tibial displacement does not predict whether or not a patient will be able to perform high level physical activities (Clark 2001; Herrington 2004). Therefore, it seems at present the best way to decide on whether a patient should undergo ACL-R surgery is whether there are persistent repetitive episodes of giving way resulting in major functional disability. In other words, how a patient performs functionally having undergone an intensive rehabilitation period. However, if an ACL injury co-exists with a major tear of the MCL or LCL, then it is generally accepted that an ACL-R should be performed (Halinen et al. 2006; Shelbourne and Porter 1992).
There is also substantial evidence that a patient who is PCL-deficient (PCL-D) can perform high-level physical activities or return to sports participation with minimal functional limitation regardless of the amount of posterior laxity present (Fontbote et al. 2005; Shelbourne et al. 1999). Therefore, it seems the best way to decide whether a patient should undergo PCL-reconstruction (PCL-R) surgery is whether there are persistent episodes of giving way and knee pain or swelling. Of consideration as to whether a PCL-R is performed is the integrity of the remaining tibiofemoral capsuloligamentous structures. If there is significant post-injury laxity in any of the other main tibiofemoral ligaments (i.e. ACL, LCL, MCL) or the posterior-lateral complex (PLC) or posterior-medial complex (PMC), then a PCL-R is recommended (LaPrade and Wentorf 2002). A PCL-R is also recommended for the PCL-D knee if the injury has resulted in a bony avulsion of either of the PCL attachments (Wilk et al. 1999).
The MCL has a greater ability to heal than the other knee ligaments, with excellent tensile strength and overall functional outcome, even after a Grade III sprain, and its is generally now recommend that non-surgical treatment and management of acute isolated MCL injuries occurs (Halinen et al. 2006). Even in instances where a combined MCL-ACL injury exists, the majority opinion is that the MCL is treated conservatively whilst an ACL-R is performed (Halinen et al. 2006; Noyes and Barber-Westin 1995). Although the LCL heals more slowly than the MCL, Grade I and II LCL tears are treated conservatively where these injuries typically present as isolated LCL trauma (Meislin 1996). Since Grade III LCL tears rarely present without combined trauma to the PLC or PCL, surgical reconstruction of the LCL and any additionally damaged adjacent capsuloligamentous structures is recommended (Noyes and Barber-Westin 2007).
Surgical repair of the menisci is often recommended to relieve related symptoms, restore knee kinematics to as near normal as possible, and restore the patient’s function, although outcomes vary considerably (Brindle et al. 2001). Multiple variables influence the decision to carry out an operative repair including the patient’s age, health, lifestyle, willingness to undergo surgery, likely post-operative adherence with rehabilitation, and the location of a tear (Brindle et al. 2001; Lee et al. 2002). Healing of the menisci is influenced by the degree of vascular penetration, with only 25–30% of the menisci outer substance possessing a viable blood supply (Brindle et al. 2001). Consequently, the middle and inner thirds of the menisci substance possess a poor blood supply and are unlikely to heal easily, if at all (Brindle et al. 2001). The alternative to meniscal repair, partial meniscectomy, frequently causes altered knee kinematics and an earlier onset of tibiofemoral OA (Lohmander et al. 2007), and so meniscal repair is preferred wherever possible (Lee et al. 2002). However, partial meniscectomy is usually performed if the tear is in the inner one-third of the meniscus substance, if the tear has major tissue fragmentation or degeneration, or for tears where the edges cannot be approximated (McLaughlin and Noyes 1993).
Use of a knee brace during rehabilitation
The use of a knee brace following knee joint injury or surgery can depend on the the type of tissue injured or the surgical procedure that has been performed (Table 21.3). Research demonstrates there is no significant difference in clinical or functional outcome in braced versus non-braced ACL-R patients (McDevitt et al. 2004; Risberg et al. 1999a). As such, there appears to be no need to use a knee brace after isolated ACL-R. In contrast to ACL injury, routine use of a knee brace after PCL injury can be essential, although specific recommendations vary for the PCL-D and PCL-R knee.
For the PCL-D knee which is to be managed non-operatively, recommendations can differ according to whether there is a partial or complete tear of the PCL (Margheritini et al. 2002). Posterior shear forces begin to dramatically increase at approximately 60° knee flexion during closed kinetic chain (CKC) knee exercise such as the double-leg bodyweight (BW) squat (Figure 21.1), BW wall-squat (Figure 21.2), horizontal double-leg press (Figure 21.3), dumb-bell single-leg squat (Figure 21.4), and horizontal single-leg press (Figure 21.5). So for the PCL-D knee with a partial tear of the ligament substance it would appear sensible to wear a long-leg hinged knee brace locked to allow 0–50° knee flexion for the first two weeks post-injury. This will protect the healing ligament from excessive tensile loads in the early stages after injury whilst permitting a functional ROM during gait, since the posterior tibial shear forces during level walking remain at relatively low loads (Shelburne et al. 2004). This ROM will also permit selected muscle strengthening exercises. The brace can then be discarded after four weeks (Margheritini et al. 2002).
Figure 21.1 Double-leg bodyweight squat. The white arrow represents how the body’s centre-of-mass can theoretically induce more relative hip, knee and ankle joint compression than joint shear. When the lower limb is extended, the body’s centre-of-mass loads the lower limb along its longitudinal axis, inducing significant hip, knee and ankle joint compression. Photograph © Copyright Nicholas Clark. Reproduced with permission.
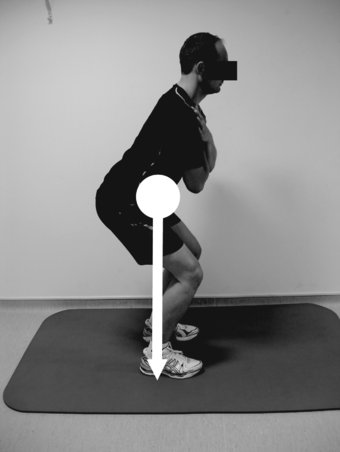
Figure 21.2 Bodyweight wall-squat. Photograph © Copyright Nicholas Clark. Reproduced with permission.
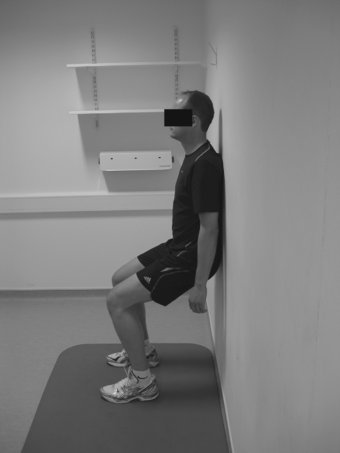
Figure 21.3 Horizontal double-leg press. White arrow represents how the leg press sled’s approximate point-of-application and line-of-action can theoretically induce more relative hip, knee and ankle joint compression than joint shear. When the lower limb is extended, the lower limb is predominantly loaded along its longitudinal axis, inducing significant hip, knee and ankle joint compression. Photograph © Copyright Nicholas Clark. Reproduced with permission.
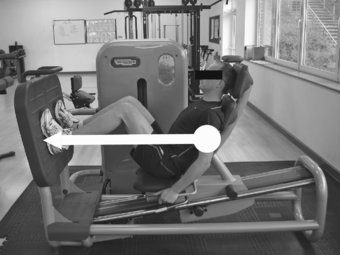
Figure 21.4 Single-leg dumb-bell squat. Photograph © Copyright Nicholas Clark. Reproduced with permission.
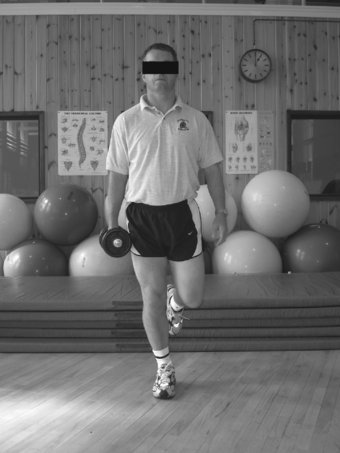
Figure 21.5 Horizontal single-leg press. White arrow represents how the leg press sled’s approximate point-of-application and line-of-action can theoretically induce more relative hip, knee and ankle joint compression than joint shear.When the lower limb is extended, the lower limb is predominantly loaded along its longitudinal axis, inducing significant hip, knee and ankle joint compression.Notice inflatable cushions under the right foot in an effort to minimise ‘cheating’ with the right leg. Photograph © Copyright Nicholas Clark. Reproduced with permission
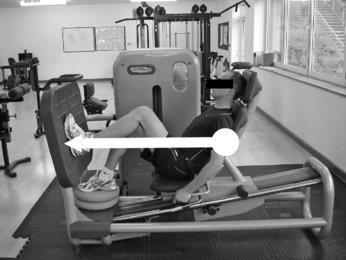
For the PCL-D knee with a complete tear of the ligament substance, a long-leg hinged knee brace locked at 0° knee flexion during ambulation is considered necessary for four weeks (Harner and Hoher, 1998). This is because the injury mechanism causing a complete tear of the PCL involves greater forces than those for a partial tear, and so it is most likely that there will be injury to the knee’s PLC or PMC also (Margheritini et al. 2002). During this time-frame, the brace can be regularly unlocked to allow 50–60° knee flexion for controlled ROM and muscle strengthening exercises (Noyes and Barber-Westin 2007). For the PCL-R knee the patient can wear a hinged knee brace locked to allow 0–50° knee flexion for the first eight weeks post-surgery. The brace is limited to 50–60° knee flexion for active ROM activities for eight weeks because this is the time-frame thought necessary for minimal soft tissue graft integration with the bone tunnel interface to occur (Harner and Hoher 1998).
Following Isolated MCL injury, a long-leg knee brace with a robust medial hinge is recommended. For the first week post-injury the brace is locked at 0° at rest and for gait and ADLs (Yoshiya et al. 2005), being unlocked frequently for passive ROM (PROM) and active ROM (AROM) exercises every day (Indelicato 1995). From week two onwards the brace can be unlocked as symptoms allow up to 90° knee flexion (Reider et al. 1993), being discarded at three to six weeks post-injury or when 90° pain-free knee flexion is achieved (Reider et al. 1993; Lind et al. 2009). Some clinicians recommend that the patient also wear the brace at night for three weeks post-injury (Reider et al. 1993).
During single-leg stance in uninjured subjects, LCL tensile forces are normally up to 8.5 times higher than MCL tensile forces (Shelburne et al. 2005), ranging from 25% to 60% BW (Schipplein and Andriacchi 1991; Shelburne et al., 2005). As such, the LCL is clearly loaded far more than the MCL, and so a long-leg hinged knee brace is considered essential even for Grade I tears (Meislin 1996). For the first seven days after isolated LCL injury, the brace is locked at 0° at rest and for gait and ADLs, being regularly unlocked for PROM and AROM exercises up to six times per day. As for isolated MCL injuries, from week two onwards the brace can be unlocked as symptoms allow up to 90° knee flexion, being discarded at three to six weeks post-injury or when 90° pain-free knee flexion is achieved and there is no quadriceps lag. If an LCL-reconstruction (LCL-R) is performed with or without a PLC-reconstruction (PLC-R), the brace is worn for at least eight weeks (Noyes and Barber-Westin 2007).
Joint loading considerations in knee rehabilitation strength training
There has been a commonly held belief that open kinetic chain (OKC) exercises such as the single-leg resisted knee extension (Figure 21.6) generate ‘excessive’ anterior tibial shear forces and ACL graft tensile loads. The term ‘excessive’ refers to the concern that OKC quadriceps strength training (i.e. resisted knee extension) induces large anterior tibial shear forces which, in turn, impose large tensile forces and load on a new ACL-R that are of sufficient magnitude to damage the healing graft-fixation complex (Fitzgerald, 1997). Although this concern is historically grounded with the best intentions for the patient, recent research demonstrates it no longer has a solid foundation in clinical practice, and so normal lower limb OKC and CKC muscle strength levels are illustrated in Table 21.4 and Table 21.5, respectively. Between-limb differences in muscle strength should be no greater than 10% as determind by normative data obtained from uninjured subjects (Daniel et al. 1982; Greenberger and Paterno 1995; Ostenberg et al. 1998; Petschnig et al. 1998; Sapega 1990).
Figure 21.6 Single-leg dynamic resisted knee extension. Curved arrow represents how the mass of the lower leg and ankle-weight can theoretically induce more relative knee joint rotation than knee joint compression. This is because when the knee is extended, the ankle-weight externally loads the lower leg perpendicular to its long axis (straight arrow). Photograph © Copyright Nicholas Clark. Reproduced with permission.
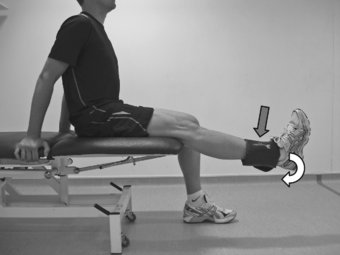
Table 21.4 Open kinetic chain quadriceps muscle strength levels
Table 21.5 Closed kinetic chain lower limb muscle strength levels
Anterior tibial shear forces are considered representative of ACL tensile forces (Escamilla et al. 1998) and vary considerably according to the type of exercise performed (Table 21.6). A resisted knee extension against a load of between 2kg and 39kg generates mean peak anterior tibial shear forces of between 50 Newtons (N) (Isaac et al. 2005) and 250N (Escamilla et al. 1998), which is equivalent to 200–250N generated by a BW forward step-up (Isaac et al. 2005). It is also significantly less than the 300–410N generated by just walking (Shelburne et al. 2004, 2005). So, based on these studies, it is difficult to reconcile why many ACL-R patients are encouraged to walk and climb stairs as soon as possible post-surgery but forbidden to perform resisted knee extensions, since walking actually generates higher anterior tibial shear forces (Table 21.6). Furthermore, as the vast majority of popular cruciate ligament reconstruction graft tissues fail at > 1900N (Table 21.7), and popular graft fixation methods for ACL-R fail at ≥ 500N post-surgery (Table 21.8), it is also clear that the 50–250N generated by sub-maximal resisted knee extensions are of insufficient magnitude to damage a properly applied graft and fixation method within correctly placed bone tunnels.
Table 21.6 Rank comparison of mean peak tibiofemoral anterior shear forces during selected strength training exercises17
Table 21.7 Mean ultimate load of cruciate ligament reconstruction
Human graft tissues | ||
Graft type | Reference | Mean ultimate load (N) |
Anterior cruciate ligament | Woo et al. (1991) | 2160 |
Posterior cruciate Ligament | Race & Amis (1994) | 4000 |
B-PT-B 7mm wide untwisted | Cooper et al. (1993) | 2238 |
B-PT-B 10mm wide untwisted | Cooper et al. (1993) | 3057 |
Noyes et al. (1984)a | 2900 | |
Staubli et al. (1999) | 1965 | |
Wilson et al. (1999) | 1784 | |
B-PT-B 10mm wide twisted | Cooper et al. (1993) | 2542 |
B-PT-B 15mm wide untwisted | Cooper et al. (1993) | 4389 |
Noyes et al. (1984)b | 2734 | |
B-PT-B 15mm wide twisted | Cooper et al. (1993) | 3397 |
QT 10mm wide untwisted | Staubli et al. (1999) | 2170 |
Single-strand semitendinosus | Hamner et al. (1999) | 1060 |
Noyes et al. (1984) | 1216 | |
Double-strand semitendinosus | Hamner et al. (1999) | 2330 |
Wilson et al. (1999) | 2422 | |
Single-strand gracilis | Hamner et al. (1999) | 837 |
Noyes et al. (1984) | 838 | |
Double-strand gracilis | Hamner et al. (1999) | 1550 |
Iliotibial band | Noyes et al. (1984) | 769 |
Quadruple-strandc unbraided | Hamner et al. (1999) | 4140 |
Kim et al. (2003) | 3000 | |
Millett et al. (2003) | 3404 | |
Quadruple-strandc braided | Kim et al. (2003) | 2215 |
Millett et al. (2003) | 2223 | |
Table © Copyright Nicholas Clark. Reproduced with permission. N = Newtons; aCentral third of patellar tendon; bMiddle third of patellar tendon B-PT-B = bone-patellar tendon-bone; QT = quadriceps tendon cRefers to four-strand composite graft formed by double-strand hamstring + double-strand gracilis graft |
Table 21.8 Mean ultimate load of common cruciate ligament reconstruction
Graft fixation methods | ||
Graft fixation method | Reference | Mean ultimate load (N) |
Paired 4mm – 5mm diameter lag screws | Campbell et al (2007)a | 762 |
Gupta et al. (2009)a | 638 | |
7mm diameter interference screw | Adam et al. (2004)b | 536 |
Kohn and Rose (1994)a | 461 | |
Pena et al. (1996)a | 640 | |
Steiner et al. (1994)a | 588 | |
9mm diameter interference screw | Camillieri et al. (2004)a | 497 |
Gerich et al. (1997)a | 678 | |
Honl et al. (2002)a | 637 | |
Kitamura et al. (2003)b | 835 | |
Kohn and Rose (1994)a | 550 | |
Kurosaka et al. (1987)a | 476 | |
Steiner et al. (1994)a | 674 | |
Zantop et al. (2004)b | 702 | |
Endobutton | Ahmad et al. (2004)b | 864 |
Honl et al. (2002)a | 572 | |
Kitamura et al. (2003)b | 580 | |
Rowden et al. (1997)a | 612 | |
Scheffler et al. (2002)a | 505 | |
Staple | Gerich et al. (1997)a | 588 |
Magen et al. (1999)b | 705 | |
Suture | Campbell et al (2007)a | 582 |
Honl et al. (2002)a | 507 | |
Steiner et al. (1994)a | 573 | |
Washer | Magen et al. (1999)b | 930 |
Scheffler et al. (2002)a | 554 | |
Cross-pin | Ahmad et al. (2004)b | 737 |
Zantop et al. (2004)b | 639 | |
MEAN of means | – | 493.5 |
Table © Copyright Nicholas Clark. Reproduced with permission. | ||
aHuman cadaver study; | ||
bAnimal study |
With regard to the clinical application of OKC and CKC strength training after ACL-R, five research groups have studied this (Bynum et al. 1995; Mikkelsen et al. 2000; Morrissey et al. 2000; Beynnon et al. 2005; Perry et al. 2005a). These five clinical research studies collectively clearly demonstrate no significant difference in anterior tibial displacement between patients performing CKC-biased quadriceps strength training or mixed CKC+OKC quadriceps strength training. Furthermore, ACL-R and ACL-D patients who performed the mixed CKC+OKC quadriceps strength training programmes consistently demonstrated significantly greater quadriceps strength versus the CKC-biased strength training patients when tested at follow-up (Mikkelsen et al. 2000; Tagesson et al. 2008). These studies all demonstrate that the clinical application of resisted OKC knee extensions with ACL-injured patients does not cause an increase in knee laxity as defined by anterior tibial displacement.
Tibiofemoral posterior shear forces during ADL, exercise and sport are frequently significantly higher than tibiofemoral anterior shear forces (Table 21.9). As such, exercise rehabilitation for the PCL-D or PCL-R knee requires greater care and slower progression than for the ACL-injured knee. This is because the normal posterior shear forces that are generated during physical activities are consistently of a greater magnitude than the lower limits of the mean ultimate load for common PCL graft fixation methods approximated at 500N (Table 21.8). Consequently, there is a high risk of damage to the PCL-R graft fixation site if exercise rehabilitation is progressed too aggressively or too quickly post-surgery. As outlined earlier, six to eight weeks is considered to be a sufficient period for initial ‘graft protection’ since this is the time-frame currently thought necessary for minimal fixation site healing and soft-tissue graft incorporation to occur at the bone tunnel interface (Harner and Hoher 1998).
Table 21.9 Rank comparison of mean peak tibiofemoral posterior shear forces during selected strength training exercises27
With regard to OKC and CKC quadriceps strength training exercises, because posterior shear forces appear to rapidly increase beyond 500N at angles greater than 50–60° of knee flexion (Table 21.9), exercises such as, for example, resisted knee extensions, single-leg-press and BW wall-squats should be limited between 0–50° knee flexion. After eight weeks, knee flexion ROM can be progressively increased in a controlled manner (Wilk et al. 1999). The authors’ preferred method of cautiously increasing ROM during controlled strength training exercises following PCL-R is by 15° knee flexion per week (Table 21.3).
With regard to OKC hamstring strength training exercise such as the prone hamstring curl (Figure 21.7a), some clinicians recommend complete abstinence from such exercises for eight weeks or more after PCL-R surgery (Wilk et al. 1999). This is due to the large posterior shear forces that can be generated during ‘maximum-effort’ resisted knee flexion exercises (Table 21.9). However, because tibiofemoral posterior shear forces do not approach 500N until well beyond 20–30° knee flexion during OKC resisted knee flexion exercises (Toutoungi et al. 2000), it is safe for the PCL-R patient to perform sub-maximal resisted isometric hamstring exercises within the limits of pain from 0–20° knee flexion (Figure 21.7b). This may deter progressive hamstring weakness secondary to the surgical trauma induced by harvesting the hamstring tendons.
Figure 21.7 (a) Single-leg dynamic resisted hamstring curl. Arrow represents how, when the knee is extended, the ankle-weight externally loads the lower leg perpendicular to its long axis. (b) Outer-range resisted isometric hamstring curl: maximum 20° knee flexion. Photograph © Copyright Nicholas Clark. Reproduced with permission.
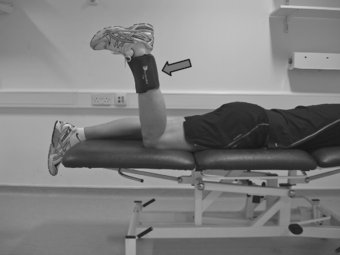
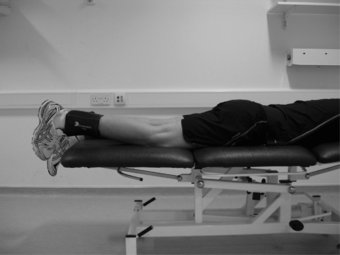
Mediolateral tibiofemoral joint shear forces during OKC and CKC rehabilitation strength training exercises are very low compared to anterior and posterior shear forces, being just 5% BW for OKC quadriceps exercise (Kaufman et al. 1991) and 12% BW for double-leg squats (Hattin et al. 1989). Since frontal plane knee alignment should be relatively neutral during these dynamic rehabilitation exercises with very little valgus rotation of the tibiofemoral joint, such exercises are clearly safe for all MCL injuries when performed within the limits of pain. Because it is normal for the knee to undergo some varus-valgus oscillation during dynamic CKC function (Kirtley 2006), the authors teach patients to maintain the tibial tubercle inbetween the first and third toes during the eccentric and concentric phases of CKC rehabilitation strength training exercises, thereby controlling excessive varus-valgus knee motion.
During standing single-leg CKC exercise LCL tensile forces dramatically increase from 60% BW in uninjured subjects up to 88% in knee injured patients (Schipplein and Andriacchi 1991; Shelburne et al. 2005). This is because it is normal for the lateral femoral condyle to ‘lift-off’ the lateral tibial plateau so that more compressive load is borne through the medial tibiofemoral joint and greater tensile loads are imposed on the lateral tibiofemoral joint (Schipplein and Andriacchi 1991). Consequently, the clinician should take great care when choosing to implement standing single-leg CKC rehabilitation strength training exercises (e.g. Figure 21.4) without a long-leg hinged knee brace. Such single-leg brace-free CKC strength training exercises are not implemented after LCL-R until at least nine weeks post-surgery (Table 21.3).
Does a surgical reconstruction prevent knee OA?
Surgical reconstruction of a torn ACL has frequently been recommended with the intention of delaying or preventing the onset of tibiofemoral OA. To date, it is clear that an ACL-R does not protect the knee from developing OA (Myklebust and Bahr 2005; Roos 2005; Keays et al. 2007; Lohmander et al. 2007). As such, following a technically proficient ACL-R, appropriate and sufficient exercise rehabilitation is critical since muscle functions to protect joint surfaces from excessive impact forces (Buckwalter, 2003). It is almost inevitable that any physically active young adult who sustains a significant tibiofemoral joint injury of any kind will develop premature knee OA during their lifespan (Gelber et al. 2000; Roos 2005; Lohmander et al. 2007). As is the case following ACL-R, PCL-R alone does not protect the tibiofemoral joint or PFJ from the development of OA (Sekiya et al. 2005; Jackson et al. 2008). Again, as for ACL-R, appropriate and sufficient exercise rehabilitation is critical following PCL-R to induce optimal neuromuscular function in order to protect joint surfaces from excessive impact forces (Buckwalter 2003; Fontbote et al. 2005).
Generic knee rehabilitation concepts
Basic concepts
Knee and lower limb biomechanics have been extensively studied with regard to running, jumping, hopping and leaping in sports (Hewett et al. 1996; Decker et al. 2002; Lewek et al. 2002; McLean et al. 2004; Fontbote et al. 2005; Noyes et al. 2005; Paterno et al. 2007; Ortiz et al. 2008). In other words, for all injured knees to perform safely and effectively in running, jumping, hopping and leaping activities without re-injury, all injured knees should eventually be able to perform the same necessary ROM and tolerate the same inherent joint compression and shear forces typical to such high-velocity high-impact movement patterns. As such, although different types of knee injury can have very different weight-bearing, ROM and rehabilitation strength training restrictions in the early stages of exercise rehabilitation (e.g. ≤ 12 weeks post-injury/ post-surgery) (Table 21.3), all types of knee injury should eventually be progressed to tolerate, for example, the same approximate joint compression and shear forces natural to running, jumping, hopping and leaping in sports in the late stages of rehabilitation (e.g. ≥ 12 weeks to 12 months post-injury/surgery). So, there are ‘generic’ knee rehabilitation concepts from early to late stage exercise rehabilitation.
Effects of proximal muscles on knee function and injury
Basic concepts
It is well established that lumbo-pelvic-hip complex frontal plane alignment can have a powerful effect on whole lower limb alignment and, in particular, knee alignment in the frontal and transverse planes (Powers 2003). It has been identified how trunk lateral flexor and gluteal muscle weakness is related to excessive knee valgus and poor frontal plane alignment during single-leg CKC tasks (Willson et al. 2006; Jacobs et al. 2007), whilst others have statistically proven how impaired trunk and gluteal muscle function is consistently linked to non-contact knee injury (Nadler et al. 2000; Zazulak et al. 2007). Moreover, Bobbert and Van Zandwijk (1999) have demonstrated how increased gluteal muscle function directly enhances both quadriceps and hamstring muscle function during dynamic CKC tasks. Therefore, generic exercises for all tibiofemoral joint injuries should include strength training for the trunk lateral flexors, gluteus maximus, and gluteus medius.
Trunk lateral flexors
The trunk lateral flexors have a profound effect on maintaining pelvic alignment in the frontal plane (Neumann 2002), and weakness and dysfunction in these muscle groups predicts non-contact knee injury (Zazulak et al. 2007). An excellent exercise to specifically target these muscle groups is the isometric side-bridge in standing (Figure 21.8) which can later be progressed to side-laying (Figure 21.9). As an exercise this can be performed using 10 repetitions per set for up to eight seconds each to avoid the effects of cumulative ischemia over multiple repetitions (McGill 2007). In testing, normal mean total holding times are ≥ 80 seconds for each side (McGill et al. 1999).
Figure 21.8 Standing isometric side-bridge. This exercise can be standardised between patients by placing the lateral border of the foot nearest the wall approximately one shoe-length from the wall. Photograph © Copyright Nicholas Clark. Reproduced with permission.
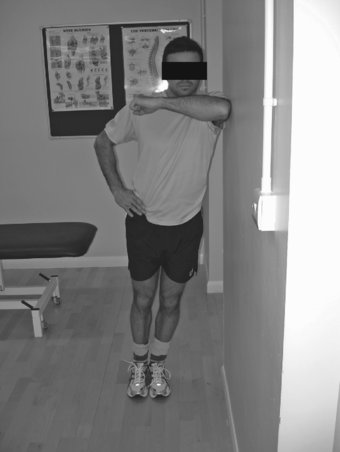
Figure 21.9 Side-laying isometric side-bridge. This exercise can be made more difficult by straightening the legs. Photograph © Copyright Nicholas Clark. Reproduced with permission.
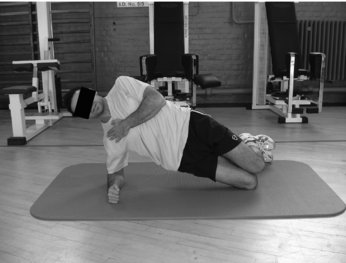
Gluteus maximus
The gluteus maximus has the ability to limit excessive hip adduction and internal rotation of the femur (Neumann 2002), also playing a crucial role in preventing lower limb flexion collapse in single-leg stance (Liu et al. 2006). Unilateral weakness of the gluteus maximus is linked to eventual onset of lower limb injury and low back pain (LBP) (Nadler et al. 2000), and so exercises for this muscle group should also be included in all knee rehabilitation programmes. Effective exercises for the gluteus maximus include prone isometric setting (Figure 21.10) eventually progressing to straight-leg hip extension in four-point kneeling (Ekstrom et al. 2007). This exercise can be performed using 10 repetitions per set for up to eight seconds each to avoid the effects of cumulative ischemia over multiple repetitions (McGill 2007).
Figure 21.10 Prone isometric gluteus maximus setting. Pillows under the torso make the position more comfortable for the patient. Pillows under the ankles place the knee in flexion to relax the hamstrings. As the patient’s ability to demonstrate good gluteus maximus isometric holding times improves, the ankle pillows can be removed and the patient can lift the leg and thigh ≤ 5cm from the floor, after which the patient can then be progressed to four-point kneeling (see text). Photograph © Copyright Nicholas Clark. Reproduced with permission.
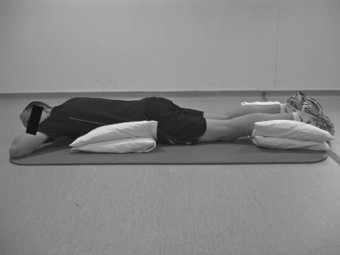
Gluteus medius
The gluteus medius is critical for both controlling frontal plane alignment of the pelvis on the femur (Neumann 2002), and for controlling knee valgus via its ability to limit excessive femoral adduction and internal rotation (Neumann 2002; Powers 2003). Since valgus collapse of the knee is linked with traumatic non-contact injury to the ACL, MCL, and lateral meniscus (Boden et al. 2000; Hewett et al. 2005) as well as non-traumatic gradual onset knee joint pain (Powers 2003; Leetun et al. 2004), exercises for this muscle are extremely important in knee injury prevention and rehabilitation programmes. Effective exercises for this muscle group are side-lying hip abduction + external rotation in ≤ 45° hip flexion with the feet supported (Figure 21.11) and side-lying straight-leg hip abduction (Ekstrom et al. 2007; Distefano et al. 2009). Exercise training and testing for this muscle can be the same as described for the gluteus maximus.
Figure 21.11 Side-laying hip abduction and external rotation at 45° hip flexion. Note how the patient is against a wall to stabilize the pelvis. This exercise can be made more difficult by extending the hips to just 30° flexion. Photograph © Copyright Nicholas Clark. Reproduced with permission.
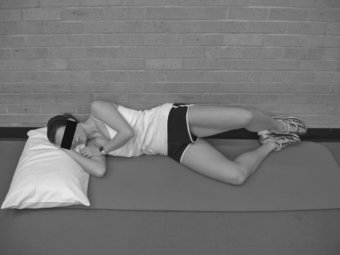
Models and levels of sensorimotor control
Motor control is defined simply as “the control of both movement and posture” (Shumway-Cook and Woollacott 1995). There are at least nine models of motor control, most of which function on a ‘sensory-motor’ basis. This means that before effective motor output is executed, accurate sensory input must be received, and there is a critical need for normal proprioceptive feedback to the CNS since mechanoreceptor feedback modifies motor output at all three levels of the CNS, these being the spinal cord, brainstem and cerebral cortex (Ghez 1991a, 1991b).
Proprioception
Proprioception has been poorly defined and confused with other sensoriomotor functions such as balance (Riemann and Lephart 2002a, 2002b). Proprioception is correctly defined as the sense of position and movement of the joints and limbs, which correspond to static joint position sense (JPS) and kinaesthesia, respectively (Martin and Jessell 1991). Several authors have identified impaired JPS and kinaesthesia in ACL-injured (Borsa et al. 1997, 1998; Roberts et al. 1999), PCL-injured (Safran et al. 1999), and meniscal-injured (Jerosch et al. 1996) patients.
In view of these findings, it is clinically useful to identify exercise training methods that are capable of enhancing peripheral joint proprioception. If we consider that the muscle spindle is the most potent proprioceptor which is always stimulated with active movements as a consequence of alpha-gamma coactivation (Gordon and Ghez 1991), any active movement can then be considered ‘proprioceptive training’ since it generates a barrage of proprioceptive impulses from the most potent (muscle) proprioceptors and joint mechanoreceptors. Consequently, OKC training (Docherty et al. 1998; Friemert et al. 2006), CKC (axial loading) training (Rogol et al. 1998), balance training on a wobble board (Waddington et al. 1999, 2000) and plyometric training (Waddington et al. 2000) have all been shown to enhance peripheral joint proprioception defined by reduced errors in active static JPS repositioning tasks.
Neuromuscular control
Definition and classification
Neuromuscular control refers to any aspect of CNS control of muscle to function as a dynamic restraint and stress shield of inert tissues and with the specific aim of maintaining functional joint stability (FJS) (Riemann and Lephart 2002a, 200b). In other words, neuromuscular control is the active restraint of excessive joint motion and the coordinated dampening of joint loads in response to specific sensory feedback (Riemann and Lephart 2002a, 2002b), and can be classified into acute and learned neuromuscular control (Clark 2008).
Strength training alone does not consistently modify potentially dangerous whole lower limb and local knee joint kinematics or kinetics (Herman et al. 2008). As such, neuromuscular control is an important concept since different exercise training methods induce the different physiological and biomechanical adaptations that comprise its separate components. This is another major premise of this chapter – different training methods induce different adaptations, and so a comprehensive injury prevention or injury rehabilitation programme must be composed of several different types of exercise in order to thoroughly encompass all the different components of neuromuscular control. Neuromuscular control can be considered as the motor component of sensorimotor control.
Acute neuromuscular control
Acute neuromuscular control is an almost instantaneous motor response to ‘at-that-moment-in-time’ sensory information about sudden joint displacements, and is composed of the electromechanical delay (EMD), and the rate-of-force development (RFD). The EMD is the timeframe between the onset of reflex muscle electrical activity and the onset of measurable force development (Winter and Brooks 1991). The RFD is the timeframe between the onset of measurable force development and the achievement of a specific quantity of force (e.g. 25% 1RM) (Kaneko et al. 2002). Relatively shorter EMD and RFD timeframes equate to faster reactive muscle activity which is clearly desirable when resisting sudden potentially dangerous knee joint displacements. To date, there is no convincing evidence that the EMD can be shortened with training, whereas the knee muscle RFD can be significantly shortened with OKC and CKC strength training (Hakkinen and Komi 1983, 1986; Hakkinen et al. 1998; Aagaard et al. 2002; Bruhn et al. 2004), as well as balance training (Bruhn et al. 2004; Gruber and Gollhofer 2004; Ihara and Nakayama 1986), and agility training (Wojtys and Huston 1996). Therefore, a selection of different training methods can enhance knee acute neuromuscular control in the form of faster reactive muscle activity.
Learned neuromuscular control
Where acute neuromuscular control refers to reactive muscle activity at a single point-in-time, learned neuromuscular control refers to how the CNS uses repeated sensory feedback generated within multiple training sessions to induce long-term training adaptations that includes joint-protective motor programmes and more favourable knee and lower limb kinematics and kinetics. Learned neuromuscular control includes decreased vertical ground reaction force (VGRF), decreased knee joint reaction force (JRF), decreased knee joint shear forces, decreased knee abduction (valgus) forces, improved frontal plane hip-knee-ankle alignment, increased hip-knee-ankle flexion, improved hamstring:quadriceps (H:Q) ratios, improved feed-forward pre-impact muscle activation, and improved postural stability. All of these components contribute to improved whole lower limb alignment and decreased knee joint forces during highly dynamic tasks, and so are highly desirable for knee injury prevention and rehabilitation programmes.
Increased VGRF during the loading response phase of landing tasks results in increased tibiofemoral anterior shear forces (McNair and Marshall 1994; Yu et al. 2006; Sell et al. 2007) and, since the VGRF is a major component of the compression JRF (Enoka 2002) increased knee impact forces. This offers the potential for both ACL and tibiofemoral articular surface injury. Balance, deceleration (landing) and plyometric training have all been shown to reduce the VGRF during athletic tasks (Hewett et al. 1996; Cowling et al. 2003; Myer et al. 2006a), and so are essential training methods for reducing knee joint shear and compression forces.
Increased knee abduction forces during landing tasks co-exist with increased valgus hip-knee-ankle frontal plane alignment and reduced hip-knee-ankle saggital plane flexion (Hewett et al. 1996, 2005; Noyes et al. 2005), placing the ACL, MCL and lateral meniscus at high risk of injury during the rapid loading response phase of deceleration manoeuvres. Open kinetic chain and CKC strength training, balance training, deceleration training and plyometric training all show the ability to reduce knee abduction forces, improve whole lower limb frontal plane alignment, and increase whole lower limb saggital plane flexion excursion (Hewett et al. 1996; Myer et al. 2005, 2006b, 2007; Noyes et al. 2005), thereby reducing potentially dangerous forces imposed on the ACL, MCL, lateral meniscus, and tibiofemoral joint articular surfaces.
Poor postural stability (i.e. balance) has been implicated in non-contact knee injury because of its detrimental effects on whole lower limb alignment and local knee valgus (Hewett et al. 2005; Myer et al. 2006a). Closed kinetic chain strength training, balance board training, deceleration training, and plyometric training have been proven to enhance postural stability during balance tasks (Myer et al. 2006a), and so are also considered critical to a knee injury prevention and rehabilitation programme.
Summary
Based on the studies just cited, and as for acute neuromuscular control, many different training methods result in different training adaptations associated with favourable learned neuromuscular control of the knee. Therefore, comprehensive knee injury prevention and rehabilitation programmes should ideally include all of the training methods just discussed either in a single training session or certainly within a weekly periodised training plan (Myer et al. 2005, 2006a, 2006b, 2007). An example of an ‘end-stage’ single-session knee rehabilitation programme sequence of exercises is presented in Figure 21.12, with exercise order moving from most complex to least complex in order to minimise the effects of fatigue (Fleck and Kraemer 1997).
Between-sex differences
There is no doubt that female athletes experience a greater relative number of knee injuries than males (Agel et al. 2005). This is also the case for low back pain (LBP) and foot, ankle and lower leg injuries (Strowbridge 2002, 2005). Compared to males, females demonstrate less knee muscle strength and less proprioceptive acuity (Rozzi et al. 1999; Lephart et al. 2002), lower reflex gluteal activation levels after foot-strike in landing tasks (Hart et al. 2004, 2007; Zazulak et al. 2005), and higher VGRF, higher knee anterior shear forces, higher knee abduction forces, and increased knee valgus alignment during deceleration manoeuvres (Hewett et al. 1996; McLean et al. 2005; Noyes et al. 2005), all of which are thought to increase females’ predisposition to increased incidence of knee injury.
In an injury prevention context, most of the neuromuscular control variables just described can be significantly improved with different exercise methods that include strength training, balance training, deceleration training and plyometric training (Lephart et al. 2005; Myer et al. 2005, 2007; Noyes et al. 2005), and such training methods have been shown to significantly reduce the incidence of non-contact male and female knee injury (Hewett et al., 1999; Mandelbaum et al. 2005; Olsen et al. 2005). In an injury rehabilitation context, even though females consistently demonstrate greater impairment in proprioception and neuromuscular control variables than males, the principles underlying female exercise rehabilitation progression can essentially be the same as for males. However, comparatively speaking, since females may well be starting their knee exercise rehabilitation at a relatively ‘lower’ proprioception and neuromuscular control ‘baseline’ than males, female knee rehabilitation and return to full function may then take longer than the time required for males.
Proposed knee exercise rehabilitation pathway
The existence of a knee exercise rehabilitation pathway (Figure 21.13) where rehabilitation is progressed in such a way that the complexity of exercise is progressed once a sufficient strength training base has been established would appear possible especially since many different training methods induce different favourable proprioception and neuromuscular control training adaptations (Clark, 2008). The rationale for focussing on isolated (OKC) and functional (CKC) muscle strength prior to the implementation of more dynamic exercise rehabilitation methods relates to ensuring injured tissues are able to first tolerate the higher tensile and compressive loads associated with more dynamic and impact exercises (Clark 2001, 2004) as well as correcting between-limb and within-limb compensations and facilitating the most desirable joint kinematics associated with enhanced force absorption during the loading response phase of walking, running, hopping and jumping gait (i.e. increased saggital plane knee flexion).
Figure 21.13 Knee exercise rehabilitation pathway. © Copyright Nicholas Clark (2008). Reproduced with permission.
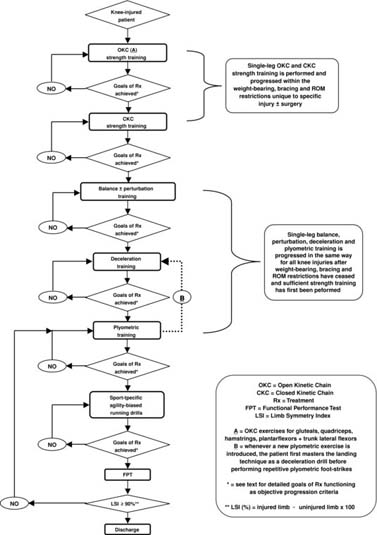
The authors present a ‘multi-modal’ knee exercise rehabilitation pathway (Clark, 2008) that has been employed with consistent success for more than seven years with recreational and professional athletes as well as frontline military operatives. The driving principle of this pathway is that patients do not progress from one exercise type to another until specific clinical criteria are achieved as goals of treatment.
These criteria will be outlined in more detail later. Such conditions ensure the patient is progressed through rehabilitation as safely and effectively as possible according to their own unique individual ability, and so goals of treatment also function as progression-criteria (Clark 2004, 2008). When implementing the pathway in Figure 21.13, it is important to remember that as the patient progresses and a new exercise type is added to a single training session, each new exercise type ‘builds’ on the type that preceded it, so that the patient is always performing all the previous exercise types for the remaining rehabilitation process.
Balance and perturbation training
Basic concepts
For the purposes of this chapter, balance training refers to single-leg CKC training performed on exercise devices that function as an unstable base-of-support (BOS). Such devices include, for example, rocker-boards (Figure 21.14), wobble-boards, foam rollers, inflatable equipment (Figure 21.15) and mini trampolines, although the clinician can obviously use any other method deemed useful for to creating an unstable BOS. The patient should be encouraged to maintain optimal pelvis-hip-knee-ankle alignment during balance training (Figure 21.15). The authors cue patients to this effect by instructing in keeping the anterior superior iliac spines (ASIS) as level as possible, and to keep the tibial tuberosity inbetween the first and third toes, at no time should the tibial tubercle deviate inside the first toe indicating valgus collapse of the knee and whole lower limb (Figure 21.15).
Figure 21.14 Frontal plane-biased rocker-board balance training. Photograph © Copyright Nicholas Clark. Reproduced with permission.
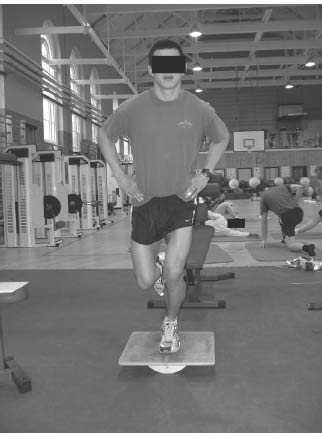
Figure 21.15 Single-leg squat on inflatable balance training device. Note the anterior superior iliac spines are level (horizontal line) and the tibial tubercle does not deviate inside the first toe (downwards arrow). Photograph © Copyright Nicholas Clark. Reproduced with permission.
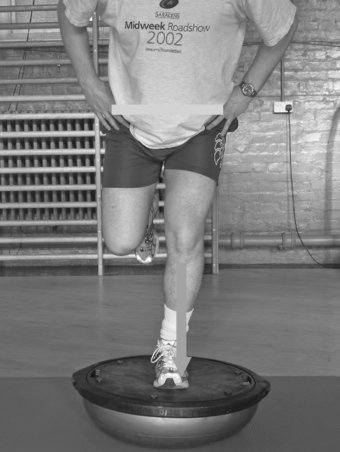
Perturbation training refers to orchestrated training situations where the clinician attempts to deliberately unbalance the patient by rhythmically tapping and knocking the balance device itself, pushing and pulling the patient at the shoulder or pelvic girdles (i.e. rhythmic stabilisations), or by distracting the patient with ball tosses (Figure 21.16). Since this type of training does not include impact forces, it seems sensible to become proficient in this method of training before introducing high-impact exercise drills (e.g. plyometrics).
Figure 21.16 Perturbation and distraction training using a ball toss. © Copyright Nicholas Clark. Reproduced with permission.
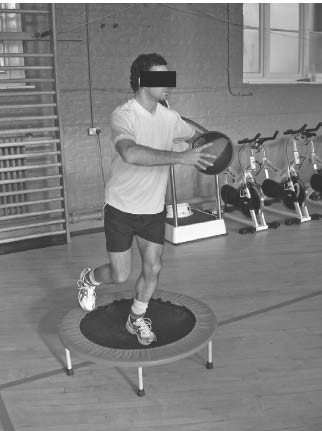
Entry Criteria
Since the intent of balance training is to perform single-leg CKC exercise on an unstable BOS, it seems appropriate to ensure patients can maintain optimal knee and whole lower limb alignment on a stable BOS. As such, patients should ideally demonstrate the ability to perform three sets of 15 repetitions of a single-leg squat to ≥ 45° knee flexion on the ground on both legs in order to demonstrate reasonable strength-endurance before undertaking balance training.
Methods
Patients can begin balance training using a rocker-board or foam roller to deliberately cause frontal plane varus-valgus oscillation of the knee (Figure 21.14), progressing to a round-base balance device for multi-planar training (Figure 21.15), and then to perturbation and distraction training (Figure 21.16). Patients should be able to perform three sets of 15 repetitions of a single-leg squat to ≥ 45° knee flexion on both legs before progressing from one balance training device to another.
Goals of treatment and objective progression criteria
The minimal goal of treatment for this type of training should be to perform three sets of 15 repetitions of a single-leg squat to ≥ 45° knee flexion on both legs on a round-base device (i.e. multi-planar balance training) before progressing to impact training (e.g. deceleration/plyometric drills).
Deceleration training
Basic concepts
Deceleration training refers to exercise drills intended to teach correct landing technique for running, jumping, hopping and leaping activities in sports and high-level occupational tasks. Such training uses exercises designed to decelerate the momentum of the lower limb and trunk following foot-strike and the onset of impact forces emphasising eccentric-biased muscle control of saggital plane knee and lower limb joint flexion, followed by isometric-biased muscle activity to stabilise the knee, lower limb and trunk with optimal frontal and transverse plane pelvis-hip-knee-ankle alignment. As such, the sequence of muscle activity is eccentric to isometric – there is no consecutive concentric propulsion phase. Clinically, such eccentric-biased drills are applied to dampen and shock-absorb high tensile and impact (compressive) forces away from joints by reducing the VGRF. In simplest terms, deceleration training is preparation for plyometric training, and always precedes the introduction of any new plyometric drill so that patients can first master correct landing technique.
Entry criteria
Since deceleration training involves high shear forces as well as high VGRF, ideal entry criteria are modified from Clark (2001, 2004, 2006), and include the normal muscle strength values outlined in Table 21.4 and Table 21.5 and Figure 21.17 and Figure 21.18, all of the trunk and gluteal muscle function recommendations described earlier in this chapter, as well as three sets of 15 repetitions of a single-leg squat to ≥ 45° knee flexion on a round-base balance device. At first glance this may seem like a high number of criteria, however many of these should already have been achieved much earlier in the rehabilitation process, and such criteria are necessary to ensure as safe and effective introduction as possible of the high joint shear and compression forces inherent in high-impact training.
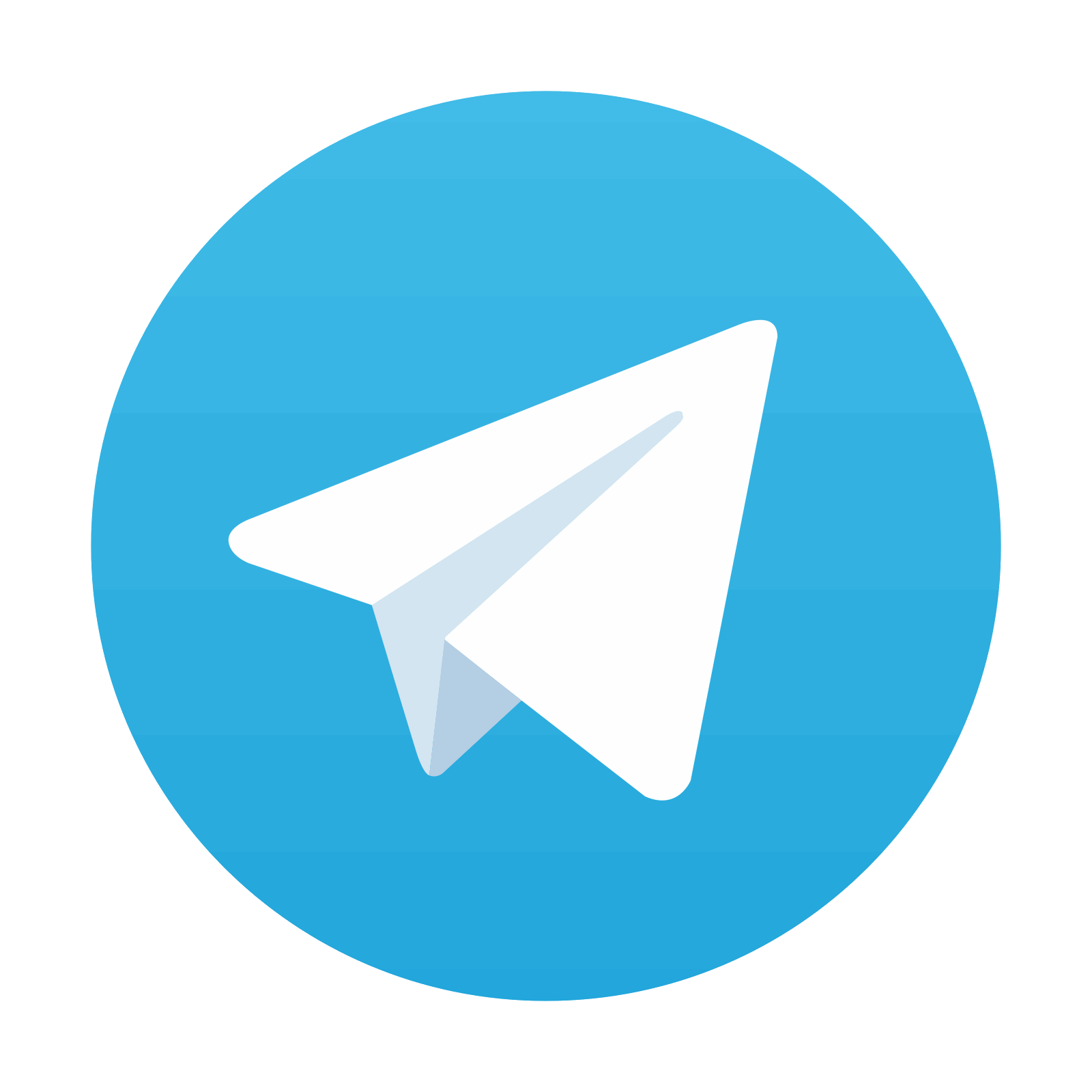
Stay updated, free articles. Join our Telegram channel

Full access? Get Clinical Tree
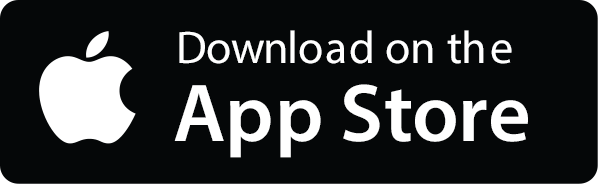
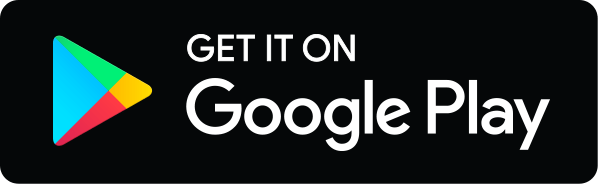