The knee joint complex is extremely elaborate and includes three articulating surfaces, which form two distinct joints contained within a single-joint capsule: the patellofemoral and tibiofemoral joints. Anatomically and biomechanically the tibiofemoral joint and the patellofemoral joint (PFJ) can be considered as separate entities, in much the same way as the craniovertebral joints are when compared with the rest of the cervical spine. In 15–20% of the population, an accessory sesamoid bone occurring in the gastrocnemius, the fabella, is present as part of the knee joint complex.1,2 The fabella, when present, articulates with the lateral femoral condyle and is hence an articular sesamoid. The knee is one of the most commonly injured joints in the body. The types of knee injuries seen clinically can be generalized into the following categories: It is important that the clinician be familiar with the diagnostic and therapeutic procedures appropriate for all of the categories of injury. It is also important that the clinician have a good understanding of differential diagnosis as pain in the thigh, knee, and calf can result from a broad spectrum of conditions. The tibiofemoral joint consists of the distal end of the femur and the proximal end of the tibia (Fig. 20-1). The tibiofemoral joint has great demands placed on it in terms of both stability and mobility. The femur is the largest bone in the body and represents approximately 25% of a person’s height.3 Its distal aspect (Fig. 20-1) is composed of two femoral condyles that are separated by an intercondylar notch or fossa. The intercondylar notch serves to accept the anterior cruciate ligament (ACL) and the posterior cruciate ligament (PCL). FIGURE 20-1 Anterior and posterior views of the bones of the tibiofemoral joint. (Reproduced, with permission, from Chapter 36. Thigh. In: Morton DA, Foreman K, Albertine KH. eds. The Big Picture: Gross Anatomy. New York, NY: McGraw-Hill; 2011.)
CHAPTER 20
The Knee
OVERVIEW
Unspecified sprains or strains, and other minor injuries, including overuse injuries
Contusions
Meniscal or ligamentous injuries
ANATOMY
TIBIOFEMORAL JOINT
The femoral condyles (Fig. 20-1) project posteriorly from the femoral shaft. The smaller lateral femoral condyle is ball-shaped and faces outward, whereas the elliptical-shaped medial femoral condyle faces inward. The lateral condyle serves as the origin of the popliteus, whereas the lateral epicondyle serves as the origin of the lateral head of the gastrocnemius and the lateral collateral ligament (LCL). The medial epicondyle (Fig. 20-1) serves as the insertion site for the adductor magnus, the medial head of the gastrocnemius, and the medial collateral ligament (MCL).
The anteroposterior length of the adult medial femoral condyle is on average 1.7 cm greater than that of its lateral counterpart, resulting in an increased length of the articular surface on the medial femoral condyle as compared with that of the lateral femoral condyle.5 Thus, the articulating surfaces are asymmetric, yet work in unison.2 The distal and the posterior portion of the femoral condyles articulate with the tibia.
The proximal tibia (Fig. 20-2) is composed of two plateaus separated by the intercondylar eminence, including the medial and lateral tibial spines.6 The tibial plateaus are concave in a mediolateral direction. In the anteroposterior direction, the medial tibial plateau is also concave, whereas the lateral is convex, producing more asymmetry and an increase in lateral mobility. The medial plateau has a surface area that is approximately 50% greater than that of the lateral plateau, and its articular surface is three times thicker.7 The concavity of the tibial plateaus is accentuated by the presence of the menisci (see later).
FIGURE 20-2 Articulating surfaces of the tibia. (Reproduced, with permission, from Chapter 36. Thigh. In: Morton DA, Foreman K, Albertine KH. eds. The Big Picture: Gross Anatomy. New York, NY: McGraw-Hill; 2011.)
PATELLOFEMORAL JOINT
The PFJ is a complex articulation, dependent on both dynamic and static restraints for its function and stability.
The patella (Fig. 20-1), a ubiquitous sesamoid bone found in birds and mammals, plays an important role in the biomechanics of the knee. Its articular anatomy is uncomplicated: it is a very hard, triangular-shaped bone, situated in the intercondylar notch and embedded in the tendon of the quadriceps femoris muscle above and the patellar tendon below. The groove has a complex architecture with increasing height of the groove’s lateral facet at the proximal aspect, providing a deeper “patella track” in knee extension.8 The thickness of the patella varies considerably, attaining a maximum height of 2–2.5 cm (0.77–1 in) at its central portion.9 The posterior surface of the patella can include up to seven facets. A smaller facet, known as the odd facet, exists medially and is delineated by a second vertical ridge. These concave medial and lateral facets are separated by a vertical ridge and are covered with aneural hyaline cartilage, the thickest cartilage in the body (up to 7-mm thick).9 The articular cartilage of the patella is unique because it lacks conformity with the underlying bone.10 The hyaline cartilage, here as elsewhere, functions to minimize the friction that occurs at the functional contact areas of the joint surfaces.
Radiographic and cadaver studies have classified the patella into three Wiberg classifications (type A–C) based on the size and shape of these lateral and medial facets.
The patellar surface of the femur is divided into medial and lateral facets that closely correspond to those on the posterior surface of the patella.11 The PFJ functions to12
provide the articulation with low friction;
protect the distal aspect of the femur from trauma and the quadriceps from attritional wear;
improve the cosmetic appearance of the knee;
improve the moment arm (distance from the center of gravity and the center of rotation) of the quadriceps. This is achieved by elevating the quadriceps femoris muscle from the center of knee rotation. This increases the efficiency of the quadriceps muscle and provides it with leverage for extending the leg. The patellar contribution to the knee extensor moment arm increases with increasing amounts of knee extension;
decrease the amount of anteroposterior tibiofemoral shear stress placed on the joint.
JOINT CAPSULE AND SYNOVIUM
The capsule of the knee joint complex is composed of a thin, strong fibrous membrane and is the largest synovial capsule in the body. The capsule ascends anteriorly approximately 2 finger breadths above the patella to form the suprapatellar pouch. Posteriorly, it ascends to the origins of the gastrocnemius. Inferiorly, the capsule attaches to the edges of the articulating surfaces of the tibial plateaus, with the exception of the intercondylar eminence, and a small portion of the anterior intercondylar region.13 A synovial membrane lines the inner portion of the knee joint capsule. By lining the joint capsule, the synovial membrane excludes the cruciate ligaments from the interior portion of the knee joint, making them extrasynovial yet intraarticular.
The articularis genu, located superior to the patella, is thought to function to retract the knee capsule during knee extension. Thus, it serves a similar function as the anconeus in the elbow.
PROXIMAL TIBIOFIBULAR JOINT
The proximal, or superior, tibiofibular joint (Fig. 20-1) is an almost plane joint with a slight convexity on the oval tibial facet and a slight concavity of the fibular head. The joint is located below the tibial plateau on the lateral condyle of the tibia. The tibial articulating facet faces laterally, posteriorly, and inferiorly. Although the joint is often described as a simple, synovial, modified ovoid, it functions as a modified sellar when combined with the distal, or inferior, tibiofibular joint (see Chapter 21).
The joint capsule of the proximal tibiofibular joint complex is thicker anteriorly than posteriorly and, in 10% of the population, the synovium is continuous with that of the knee joint.14 The joint receives support from anterior and posterior ligaments, and an interosseous membrane. The interosseous membrane attaches between the medial border of the fibula and the lateral border of the tibia, providing attachment to the deep anterior and posterior muscles of the leg. The majority of its fibers pass obliquely in an inferior and lateral direction.
The proximal tibiofibular joint has more motion than its distal partner. The motion occurring at the proximal joint, consists of two glides, one in a superoinferior direction, and the other in an anteroposterior direction.7 These motions are possible because of the orientation of the joint line, which also facilitates an osteokinematic spin of the fibula. The motion at this joint can be decreased by articular fibrosis or by soft-tissue restraints; the biceps femoris can pull or hold it posteriorly, whereas the tibialis anterior can pull or hold it anteriorly.
Although the capsular pattern of restriction for this joint is unclear, it is probably indicated by pain during an isometric contraction of the biceps femoris with the knee at 90 degrees of flexion. The close-packed position for this joint, equally debatable, is probably weight-bearing (WB) ankle dorsiflexion.
Both the tibia and fibula are vulnerable to fracture at the lower third of their shaft. Anterior joint subluxations occur at this joint as a result of medial knee joint strain or an inversion sprain of the ankle. Posterior joint subluxation can occur as a result of a lateral knee joint strain, but is often missed because of the more serious ligament damage to the knee.
The nerve supply to this joint is provided by the common fibular (peroneal) and recurrent articular nerves. The joint receives its blood supply from a perforating branch of the fibular (peroneal) artery, and the anterior tibial artery.
LIGAMENTS
The static stability of the knee joint complex depends on four major knee ligaments, which provide a primary restraint to abnormal knee motion:
Anterior cruciate (Fig. 20-1). Provides the primary restraint to anterior translation and medial rotation of the tibia on the femur and is a secondary restraint to valgus and varus rotation of the tibia.
Posterior cruciate (Fig. 20-1). Provides the primary restraint to posterior translation and medial rotation of the tibia on the femur and is a secondary restraint to valgus and varus rotation of the tibia.
Medial (tibial) collateral (Fig. 20-1). Provides the primary restraint to valgus and lateral rotation of the tibia, and is a secondary restraint to the anterior and posterior translation of the tibia on the femur.
Lateral (fibular) collateral (Fig. 20-1). Provides the primary restraint to varus and lateral rotation of the tibia, and is a secondary restraint to the anterior and posterior translation of the tibia on the femur.
Cruciate Ligaments
The two central intraarticular cruciate ligaments derive their name from the Latin word crucere (cross) because they cross each other (Fig. 20-1). Both the ACL and PCL lie in the center of the joint, and each is named according to their attachment sites on the tibia.14 The cruciate ligaments, which differ from those of other joints in that they restrict normal, rather than abnormal, motion, are the main stabilizing ligaments of the knee and restrain against anterior (ACL) and posterior (PCL) translations of the tibia on the femur. They also restrain against excessive internal and external rotation and varus movement of the tibia.
The blood supply to the cruciate ligaments is largely provided from the middle and inferior geniculate branches of the popliteal artery. The cruciate ligaments are innervated by the posterior articular nerve, a branch of the posterior tibial nerve.18 The function of this nerve supply is questionable although it may serve as proprioceptive in nature.19 In addition, the cruciate ligaments contain mechanoreceptors, suggesting that disruption of the ligament structure can produce partial deafferentation of the joint.20 Evidence of a proprioceptive function of the ACL comes from extensive histologic observations demonstrating that the ACL appears to contain proprioceptive nerve endings.21,22 Indeed, Krauspe et al.,18 in single-fiber studies, identified a total of 26 mechanoreceptors of the cruciate ligament among 13 animals.
Although these cruciate ligaments function together, they are described separately.
Anterior Cruciate Ligament
The ACL is a unique structure and one of the most important ligaments to knee stability, serving as a primary restraint to anterior translation of the tibia relative to the femur, and a secondary restraint to both internal and external rotation in the non–weight-bearing (NWB) knee.23–26 An injury to this ligament has terminated many a promising sports career.27,28
The ACL (Fig. 20-1) is composed of a vast array of individual fascicles. These, in turn, are composed of numerous interlacing networks of collagen fibrils. The fascicles originate on the inner aspect of the lateral femoral condyle in the intercondylar notch (resident’s ridge), which serves as the anterior limit of the ACL in the anatomical position, and travel obliquely and distally through the knee joint. They enter the anterior intercondylar surface of the tibial plateau, where they partially blend with the lateral meniscus. As the fascicles of the ACL course through the knee joint and attach to their insertion sites, they fan out and give a slight spiral appearance to the ligament, a phenomenon that is more pronounced during knee flexion.29,30
The synovial tissue that enfolds the ACL consists of an intimal layer, facing the joint cavity, and a subsynovial layer. The subsynovial layer is in direct contact with the ACL and contains neurovascular structures. The ACL is considered intraarticular yet extrasynovial because of the posterior invagination of the synovial membrane.
Although the posterior articular nerve is the major nerve for the ACL, afferent fibers have also been demonstrated in the medial and lateral articular nerves.31
Like all ligaments, the ACL behaves as a viscoelastic structure, allowing it to dissipate energy and to adjust its length and internal load distribution as a function of load history.32,33 This means that the normal ACL is capable of microscopic adjustments to internal stresses over time, thus influencing the laxity, stresses, and kinematics of the joint in subtle but potentially important ways.34 One anatomic factor that contributes to selective fiber recruitment during tensile loading is the specific location of the insertions of the ACL on the femur and the tibia. These differing insertion sites allow different fibers of the ACL to be recruited with every subtle three-dimensional (3D) change in the position of the joint.29,34–36
The ACL consists of two functional bundles—the anteromedial (AM) and posterolateral (PL) bundles—named for their position on the tibia. Butler et al.37 have shown that whatever the angle of knee flexion, the ACL absorbs nearly 90% of the force causing anterior translation.26,38,39 The femoral footprints of the AM and PL bundles are vertically aligned when the knee is in full extension, and the femoral origin of the AM bundle is located superior to the PL insertion.40 In this configuration, the two bundles are parallel to each other, whereas with the knee in 90 degrees of flexion, the two bundles cross each other, and the femoral insertions are nearly horizontally aligned.40,41 The tensile strength of the ACL is equal to that of the knee collaterals, but is half that of the PCL.42 Since its fibers are unyielding, forcing the ACL more than 5% beyond its resting length may result in rupture.26 Several factors can influence the amount of tension on the ACL41,43:
When the knee is in full extension, the AM and PL bundles are under tension.
When the knee is at 60 to 90 degrees of flexion, the PL bundle is lax and allows rotation of the tibia on the femur.
The PL bundle limits anterior translation of the tibia at low angles of knee flexion (0–30 degrees).
The AM bundle primarily resists anterior translation of the tibia and undergoes less change in length than the PL bundle throughout the range of knee motion.
The PL bundle is maximally lengthened when the knee is in full extension, and the AM bundle is under maximum tension when the knee is flexed between 45 degrees and 60 degrees.
Compressive loading of the tibiofemoral joint, such as that occurs during WB, has been shown to reduce anteroposterior laxity and stiffen the joint when compared with the NWB.44 These changes appear to reflect the increased strain borne by the ACL during the transition from NWB to WB.45 Thus, the popular belief in the beneficial effects of early WB and closed-kinetic chain exercises (CKCEs) following anterior cruciate reconstruction may be open to question (Table 20-1).45
TABLE 20-1 | Rank Comparison of Peak ACL Strain Values During Commonly Prescribed Rehabilitation Activities |
Rehabilitation Activity | Peak Strain (Mean ± ISD) | No. of Subjects |
Isometric quads contraction at 15 degrees (30 Newton meters [Nm] of extension torque) | 4.4 (0.6)% | 8 |
Squatting with sport cord | 4.0 (1.7)% | 8 |
Active flexion–extension of the knee with 45-N weight boot | 3.8 (0.5)% | 9 |
Lachman test (150 N of anterior shear load: 30 degrees of flexion) | 3.7 (0.8)% | 10 |
Squatting | 3.6 (1.3)% | 8 |
Active flexion–extension (no weight boot) of the knee | 2.8 (0.8)% | 18 |
Simultaneous quadriceps and hamstring contraction at 15 degrees | 2.8 (0.9)% | 8 |
Isometric quadriceps contraction at 30 degrees (30 Nm of extension torque) | 2.7 (0.5)% | 18 |
Stair climbing | 2.7 (2.9)% | 5 |
Anterior drawer (150 N of anterior shear load: 90 degrees of flexion) | 1.8 (0.9)% | 10 |
Stationary bicycling | 1.7 (1.9)% | 8 |
Isometric hamstring contraction at 15 degrees (10 Nm of flexion torque) | 0.6 (0.9)% | 8 |
Simultaneous quadriceps and hamstring contraction at 30 degrees | 0.4 (0.5)% | 8 |
Passive flexion–extension of the knee | 0.1 (0.9)% | 10 |
Isometric quads contraction at 60 degrees (30 Nm of extension torque) | 0.0% | 8 |
Isometric quadriceps contraction at 90 degrees (30 Nm of extension torque) | 0.0% | 18 |
Simultaneous quadriceps and hamstring contraction at 60 degrees | 0.0% | 8 |
Simultaneous quadriceps and hamstring contraction at 90 degrees | 0.0% | 8 |
Isometric hamstring contraction at 30 degrees, 60 degrees, and 90 degrees to −10 Nm of flexion torque | 0.0% | 8 |
ACL, anterior cruciate ligament; ISD, implied standard deviation. Data from Beynnon BD, Fleming BC. Anterior cruciate ligament strain in-vivo: A review of previous work. J Biomech. 1998;31:519–525. |
Stair-climbing exercises using exercise equipment such as the Stairmaster 4000PT (Randall Sports Medicine, Kirkland, WA) have been shown to produce moderate strain on the ACL compared with other rehabilitation activities.46
The circumstances that cause the highest loads and strains on the ACL during daily function are34 quadriceps-powered extensions of the knee, moving it from approximately 40 degrees of flexion to full extension; hyperextension of the knee; excessive internal tibial rotation; or excessive varus or valgus stress on the tibia if a collateral ligament is torn.
Both estimates47,48 and measurements23,24 have shown that the maximum measured strain differential in the ACL is approximately 5% during any rehabilitation exercise.34,49 This strain represents only approximately one-quarter of the failure strain of the normal ACL, suggesting that these exercises load the normal ACL to only a small fraction of its failure capacity.32,34,36
Posterior Cruciate Ligament
The PCL attaches posteriorly to the insertion of the posterior horns of the lateral and medial menisci on the posterior part of the posterior intercondylar fossa of the tibia.50 From here, the PCL extends obliquely medially, anteriorly, and superiorly to attach to the lateral surface of the medial femoral condyle (Fig. 20-1). Overall, the ligament is wider at its femoral origin and narrowest near the tibial insertion.51 The PCL is covered by synovial lining and is therefore considered to be extrasynovial yet intraarticular.
Information regarding the biomechanical function of the PCL is scant compared with that of the ACL. It is known that the PCL is 50% thicker and has twice the tensile strength of the ACL.52 The PCL is more vertical in extension and horizontal in flexion.53 Like the ACL, the PCL consists of two bundles: anterior lateral and posterior medial. Overall, the ligament is most taut with further flexion of the knee.54 Specifically, the anterior lateral bundle is taut in flexion, while the posterior medial bundle is taut in extension. According to Butler et al.,37 the PCL provides 90–95% of the total restraint to posterior translation of the tibia on the femur, with the remainder being provided by the collateral ligaments, posterior portion of the medial and lateral capsules, and the popliteus tendon. The contribution percentage resisting posterior translation decreases as the knee extends. The PCL is significantly loaded if a posteriorly directed force is applied to the tibia when the knee is flexed to 90 degrees or greater while in neutral rotation.55 If the same force is applied to the tibia when the knee is in terminal extension, the load on the PCL is not increased.55 This is contrary to the popular belief that hyperextension of the knee is the mechanism of injury of the PCL. The PCL also restrains internal rotation of the tibia on the femur and helps prevent posteromedial instability at the knee.56
Other functions of the PCL include acting as a secondary restraint to external rotation of the tibia on the femur at 90 degrees of flexion,57 assisting with a rolling/gliding mechanism of the tibiofemoral joint, and resisting varus/valgus forces at the knee after the collateral ligaments have failed.
A significant force is needed to tear the PCL. Thus, tears of the PCL are usually the result of severe contact injuries that often occur in traumatic situations, such as a dashboard injury during a motor vehicle accident.
In concert with the PCL are the anterior and posterior meniscofemoral ligaments, which comprise approximately 22–50% of the cross-sectional area of the PCL.51,58 Although these ligaments arise from the common PCL origin they are distinct structures having different insertion sites.59 Both are named with respect to the orientation to the PCL (see “Lateral Meniscus”). Since the insertion site of the meniscofemoral ligaments is different from that of the PCL, if a midsubstance tear of the PCL occurs, it is possible that one or both of the meniscofemoral ligaments may remain intact.60
Medial Collateral Ligament
Both the MCL and the LCL are considered to be extra-articular ligaments.
The MCL, or tibial collateral ligament (Fig. 20-1), develops as a thickening of the medial joint capsule.61 It can be subdivided into a superficial band and a deep band.
The superficial band is a thick, flat band, and has a fan-like attachment proximally on the medial femoral condyle, just distal to the adductor tubercle, from which it extends to the medial surface of the tibia approximately 6 cm below the joint line, covering the medial inferior genicular artery and nerve.62 The superficial band blends with the posteromedial corner of the capsule and, when combined, is referred to as the posterior oblique ligament.63 The superficial band is separated from the deep layer of the ligament by a bursa. Since the superficial band is farther from the center of the knee, it is the first ligament injured when a valgus stress is applied.64
The deep band (medial capsular ligament) is a continuation of the capsule. It blends with the medial meniscus and consists of an upper meniscofemoral portion and a lower meniscotibial portion.
The anterior fibers of the MCL are taut in flexion and can be palpated easily in this position. The posterior fibers, which are taut in extension, blend intimately with the capsule and with the medial border of the medial meniscus, making them difficult to palpate.
Information regarding the biomechanical function of the collateral ligaments is quite scarce compared with that of the ACL. It would appear that the MCL is the primary stabilizer of the medial side of the knee against valgus forces, and external rotation of the tibia, especially when the knee is flexed.65 Grood et al.66 determined that the MCL was the primary restraint, providing 57% and 78% of the total restraining moment against valgus force at 5 and 25 degrees of flexion.67
Lateral Collateral Ligament
The LCL, or fibular collateral ligament (Fig. 20-1), arises from the lateral femoral condyle and runs distally and posteriorly to insert into the head of the fibula. The LCL forms part of the so-called arcuate–ligamentous complex. This complex also comprises the biceps femoris tendon, iliotibial tract, and the popliteus.
The cord-like LCL develops independently and remains completely free from the joint capsule and the lateral meniscus. It is separated from these structures by the popliteus tendon and straddled by the split tendon of the biceps femoris. The LCL can be divided into three parts:
- Anterior. This part consists of the joint capsule.
- Middle. This part is considered to be part of the iliotibial band (ITB) and covers the capsular ligament.
- Posterior. This Y-shaped portion of the ligament is part of the arcuate–ligamentous complex, which supports the posterior capsule.69,70
The main function of the LCL is to resist varus forces. It offers the majority of the varus restraint at 25 degrees of knee flexion,71,72 and in full extension. Abnormal varus laxity arising subsequent to injuries of the LCL and other PL structures has been shown to increase stress on cruciate ligament grafts and has been implicated as one of the causes of crucial ligament reconstruction failure.68
OTHER RESTRAINTS
Some structures in the knee clearly augment the functions of the ACL and the PCL. These structures are known as secondary restraints.34 The secondary restraints include the structures in the PL and posteromedial corners of the knee, which serve to control anterior tibial translation relative to the femur.34,55,73,74
Dynamic stability synergistic to the PCL is provided by unopposed contraction of the quadriceps complex, which increases anterior tibial translation. Conversely, an isolated contraction of the hamstrings results in a posterior translation of the tibia, which is synergistic to the ACL. Cocontraction of the hamstrings and the quadriceps has been theorized to minimize tibial translation in either direction.34 The popliteus muscle–tendon complex (PMTC) contributes to both static and dynamic PL knee joint stabilization.75 During concentric activation, the popliteus internally rotates the tibia on the femur. During eccentric activation, it serves as a secondary restraint to tibial external rotation on the femur (see “Popliteus”).76
The knee joint is also strengthened externally by the patellar ligament, oblique popliteal ligaments, and the fabella.
- The patellar ligament, or patellar tendon, lies in the thickened portion of the quadriceps femoris tendon between the top of the patella and the tibia (Fig. 20-1). The patellar ligament strengthens the anterior portion of the knee joint and prevents the lower leg from being flexed excessively.
- The oblique popliteal ligament, located on the posterior surface of the knee joint, is a dense thickening in the posterior capsule made up of a continuation of the popliteal tendon and part of the insertion of the semimembranosus.66 It arises posterior to the medial condyle of the tibia and extends superomedially to attach to the posterior fibrous capsule. The oblique popliteal ligament provides reinforcement to the lateral capsule, limits AM rotation of the tibia, and prevents hyperextension of the knee.
- The fabella is located in the PL corner of the knee and may be osseous or cartilaginous in makeup. When the fabella is present, there is a fabellofibular ligament, which courses superiorly and obliquely from the lateral head of the gastrocnemius to the fibular styloid.70 The fabellofibular ligament helps prevent excessive internal rotation of the tibia and adds further ligamentous support on the lateral and PL aspects of the knee.77 Seebacher et al. found through dissection that the arcuate ligament was quite large in the absence of a fabella.1
MENISCI
The word meniscus comes from the Greek word mēniskos, meaning “crescent,”, diminutive of mēnē, meaning “moon.”78 The lateral and medial menisci (Fig. 20-2), attached on top of the tibial plateaus, lie between the articular cartilage of the femur and the tibia. The knee meniscus was once described as “a functionless embryonic remnant.”79 However, both menisci are now recognized as being vital for the normal function and long-term health of the knee joint. The characteristic crescent shape of the medial and lateral menisci is achieved between the 8th and 10th week of gestation.80 The unique and complex structure of the menisci makes treatment and repair challenging and, if left untreated, may lead to degenerative joint changes.80a The blood supply of the meniscus, which is key to successful meniscal recovery or repair, comes from the perimeniscal capsular arteries, which are branches of the lateral, medial, and middle genicular arteries, which in turn are branches of the popliteal artery.80 Although the developing menisci are highly vascular throughout, by adulthood this vascularity has significantly reduced such that only the outer 10–25% of the lateral meniscus (with the exception of the PL corner of the lateral meniscus adjacent to the popliteus tendon) and the outer 10–30% of the medial menisci are vascularized (referred to as the red zone), which allows these areas to have the potential for healing.81 The remaining inner portions of the menisci (65–75%) are considered avascular and have less potential to heal. These inner portions receive nourishment from the synovial fluid by diffusion or mechanical pumping (i.e., joint motion).78 However, despite the lack of vascularity to the inner portions, tears involving the avascular zone may heal. This healing capacity may be improved with the addition of a fibrin clot or with such techniques as trephination (making a burr hole).82,83
Medial Meniscus
The crescent-shaped, or U-shaped, medial meniscus (Fig. 20-2), with the wider separation of its anterior and posterior horns, is larger and thicker than its lateral counterpart and sits in the concave medial tibial plateau.84 The medial meniscus is significantly wider posteriorly than anteriorly. It is attached to the anterior and posterior tibial plateau by coronary ligaments. These ligaments connect the outer meniscal borders with the tibial edge and restrict movement of the meniscus. The medial meniscus also has an attachment to the deeper portion of the MCL and the knee joint capsule. The horns of the lateral meniscus are closer together than those of the medial, which makes the former almost circular and the latter nearly semilunar (Fig. 20-2). The anterior horn is attached to the tibial plateau near the intercondylar fossa anterior to the ACL.80 There is significant variability in the attachment location of the anterior horn of the medial meniscus.78 The posterior horn of the medial meniscus, which is attached to the posterior intercondylar fossa of the tibia between the lateral meniscus and the PCL, receives a piece of the semimembranosus tendon.78
The transverse genicular ligament serves as a link between the lateral and medial menisci.
Lateral Meniscus
The lateral meniscus, which forms a C-shaped incomplete circle,84 sits atop the convex lateral tibial plateau (Fig. 20-2). It occupies a larger portion (approximately 80%) of the articular surface than the medial meniscus (approximately 60%) and is more mobile than its medial counterpart.78 Both horns of the lateral meniscus are attached to the tibia.78
The periphery of the lateral meniscus attaches to the tibia, the capsule, and the coronary ligament, but not to the LCL. The posterior horn of the lateral meniscus attaches to the inner aspect of the medial femoral condyle via the anterior and posterior meniscofemoral ligaments of Humphrey and Wrisberg, respectively78:
- The ligament of Humphrey, also known as the anterior meniscofemoral ligament, runs anteriorly to the PCL and inserts on the posterior aspect of the lateral meniscus.59
- The ligament of Wrisberg, also known as the posterior meniscofemoral ligament, runs posteriorly to the PCL to insert either into the superior/lateral aspect of the tibia, the posterior aspect of the lateral meniscus, or the posterior capsule.85
The meniscofemoral ligaments become taut with internal rotation of the tibia and help with stabilization of the meniscus.62
The lateral meniscus has an interesting relationship with the popliteus tendon, which supports it during knee extension and separates it from the joint (see later discussion).75
Menisci Function
The menisci assist in a number of functions, including load transmission, shock absorption, joint lubrication and nutrition, secondary mechanical stability (particularly the posterior horn of the medial meniscus that blocks anterior translation of the tibia on the femur),84 and the guiding of movements.86
Load Transmission
The meniscus is viscoelastic, with greater stiffness at higher deformation rates. Many studies have confirmed the role of the menisci in load transmission by showing decreased contact area and increased peak articular stresses following partial or total meniscectomy.86–89 WB produces axial forces across the knee, which compress the menisci, resulting in “hoop” (circumferential) stresses. By converting joint loading forces to radial-directed hoop stresses on their circumferential collagen fibers, and taking advantage of their viscoelastic nature, the menisci transmit 50–60% of the joint load when the knee is in extension, and 85–90% when the knee is in flexion.90–92 The lateral meniscus carries 70% of the compressive load in the lateral compartment, compared with just 40% of the medial meniscus in its respective compartment.84
Shock Absorption
Because of their viscoelastic nature, the menisci are able to assist in shock absorption. During the normal gait pattern, the articular surface of the knee bears up to six times the body weight, with over 70% of that load borne by the medial tibial plateau.84 The medial tibial plateau bears most of this load during stance when the knee is extended, with the lateral tibial plateau bearing more of the much smaller loads imposed during the swing phase.93 This is compensated for by the fact that the medial tibial plateau has a surface area roughly 50% larger than the lateral plateau, and articular cartilage that is approximately three times thicker than the lateral articular cartilage.94
Joint Lubrication
The menisci assist in joint lubrication by helping to compress synovial fluid into the articular cartilage, which reduces frictional forces during WB. A meniscectomy increases the coefficient of friction within the knee, thereby increasing the stresses on the articular surfaces.97
Joint Stability
The menisci deepen the articulating surfaces of tibial plateaus. This increases the stability of the knee, especially during axial rotation and valgus–varus stresses.97,98
If the ACL is intact, the menisci do not significantly contribute to anteroposterior stability.99 However, in an ACL-deficient knee, the posterior horn of the medial meniscus functions as a secondary restraint to an anteroposterior translation by wedging between the femur and the tibia.100,101 In contrast, the increased mobility of the lateral meniscus prevents it from contributing to anteroposterior stability.102 This difference helps to explain the higher incidence of medial meniscus tears seen in ACL-deficient knees.102
Proprioception
Mechanoreceptors have been identified in the anterior and posterior horns of the menisci.103 The presence of these mechanoreceptors would seem to suggest that the menisci are capable of detecting proprioceptive information in the knee joint, thus playing an important afferent role in the sensory feedback mechanism of the knee.78
Guiding Movement
During flexion and extension of the knee, the menisci move posteriorly and anteriorly, respectively. The lateral meniscus has greater mobility because it does not attach to the LCL, and, as mentioned previously, its capsular attachment is interrupted by the passage of the popliteus tendon.75,104 The posterior medial corner of the medial meniscus has the least amount of motion because it is constrained by its attachment to the tibial plateau by the meniscotibial portion of the posterior oblique ligament which has been reported to be more prone to injury.78 During knee motion, the menisci move on the tibial plateau with the femoral condyles to maintain joint congruence.104 The femur, accompanied by the menisci, rolls anteriorly on the tibia during extension, and posteriorly during flexion (Fig. 20-3A). Thompson et al.105 demonstrated that the mean anteroposterior excursion of the menisci during a 120-degree arc of motion was 5.1 mm at the medial meniscus, and 11.2 mm at the lateral. The inner sides of the menisci, which are attached by their horns to the tibial plateau, move with the tibia. As the body of each meniscus is fixed around the femoral condyle, they move with the femur. Therefore, during movements between tibia and femur, distortion of the menisci is inevitable.
FIGURE 20-3 Tibiofemoral motions.
During flexion of the knee, the menisci move posteriorly. The medial meniscus is moved about 5 mm by the pull of the semimembranosus tendon, and the lateral meniscus is pulled about 11 mm by the popliteus, resulting in an external rotation of the tibia.
During extension, the menisci move anteriorly.
During external rotation of the tibia, the menisci will follow the displacement of the femoral condyles, which means that the medial meniscus is pushed posteriorly, and the lateral meniscus is pulled anteriorly. During internal rotation, the opposite occurs. These rotations are conjunct, integral with flexion and extension, but can also be adjunct and independent, best demonstrated with the knee semiflexed. Conjunct external rotation of the tibia on the femur during the last stages of knee extension is part of a locking mechanism called the “screw home” mechanism (Fig. 20-3B), described later.
The medial coronary ligament is stretched during external rotation of the tibia, whereas the lateral coronary ligament is stretched during internal rotation of the tibia.
BURSAE
There are a number of bursae situated in the soft tissues around the knee joint. The bursae serve to reduce friction and to cushion the movement of one body part over another.
Superficial and Deep Infrapatellar Bursae
The superficial infrapatellar bursa is located between the patellar tendon and the skin, whereas the deep infrapatellar bursa is located between the patellar tendon and the tibia.
Prepatellar Bursa
The prepatellar bursa is located between the skin and the anterior aspect of the patella.
Tibiofemoral Bursa
The tibiofemoral bursae consist of a bursa between the head of the gastrocnemius muscle and the joint capsule on both sides, a bursa between the LCL and both the biceps femoris and popliteus, and a bursa between the MCL and the femoral condyle. There are also a number of bursae between the various tendons of the pes anserinus and between the MCL and the superficial pes anserinus.
The bursae around the knee can have contact with each other and with the knee joint capsule.
Fat Pads
There are three fat pads located at the anterior knee: the quadriceps fat pad, the prefemoral fat pad, and the infrapatellar (Hoffa’s) fat pad. The fat pads of the knee house neurovascular projections. The functions of the fat pads include the following:
Synovial fluid secretion
Joint stability
Neurovascular supply
Occupiers of dead space
PLICA
Synovial plicae of the knee were first described at the beginning of the last century.106,107 Postmortem studies have shown plica to be present in 20–50% of knees,108–110 with the highest prevalence in individuals of Japanese descent.107,111–114
Synovial plica represents a remnant of the three separate cavities in the synovial mesenchyme of the developing knee. These cavities are supposed to coalesce into one cavity at the 12-week stage of fetal growth.115,116 The size and extent of this remnant depend on the degree of reabsorption.117
The three joints involved in the developing knee from which the remnants evolve are113
- the joint between the fibular and the femur;
- the joint between the tibia and the femur;
- the joint between the patella and the femur.
The most common plica in the knee is called the anterior or inferior plica, or mucous ligament.115,118 This plica is represented by a tape-like fold running from the fat pad to the intercondylar notch of the femur and overlying the ACL. The plicae to the medial and lateral sides of the patella, which run in a horizontal plane from the fat pad to the side of the patellar retinaculum, are referred to as the superomedial or superolateral plicae or the suprapatellar membrane, or the medial or lateral synovial shelf.115
It has been suggested that symptomatic synovial plicae are one of the causes of anterior knee pain in children and adolescents.119–121
RETINACULA
The wing-like retinacula of the knee are formed from structures in the first and second layers of the knee joint. The retinacula can be subdivided into the medial and the lateral retinacula for clinical examination and intervention purposes.122,123 The retinacula serve to connect the patella to a number of structures, including the femur, menisci, and tibia, both medially and laterally.124
The lateral retinaculum is the stronger and thicker of the two. It consists of two distinct layers of fibrous connective tissue: the superficial and deep retinacula. These structures are oriented longitudinally with the knee extended.123
The superficial retinaculum consists of fibers from the vastus lateralis (VL) and the ITB.125
The deep retinaculum consists of the lateral patellofemoral ligament, the deep fibers of the ITB, and the lateral patellotibial ligament.125 These structures connect the patella to the ITB and help prevent medial patellar excursion.126
Although partially located deep to the ITB, the lateral retinaculum is blended with the biceps femoris to form a so-called conjoint tendon.1,123 This relationship may explain why adaptively shortened hamstrings can lead to patellofemoral symptoms.124 It is also well established that adaptive shortening of the lateral retinaculum is a common finding in patellofemoral dysfunction.127–131
Given the fact that the medial retinaculum is thinner than the lateral retinaculum, it is not thought to be as significant to patella position and tracking as its lateral counterpart.
MUSCLES
The major muscles that act on the knee joint complex are the quadriceps, the hamstrings (semimembranosus, semitendinosus, and biceps femoris), the gastrocnemius, the popliteus, and the hip adductors (Table 20-2).
TABLE 20-2 | Muscles of the Knee: Actions, Nerve Supply, and Nerve Root Derivation |
Action | Primary Muscles | Peripheral Nerve Supply | Nerve Root Derivation |
Flexion of knee | Biceps femoris Semimembranosus Semitendinosus Gracilis Sartorius Popliteus Gastrocnemius Tensor fascia latae | Sciatic Sciatic Sciatic Obturator Femoral Tibial Tibial Superior gluteal | L5, S1–2 L5, S2–2 L5, S1–2 L2–3 L2–3 L4–5, S1 S1–2 L4–5 |
Extension of knee | Rectus femoris Vastus medialis Vastus intermedius Vastus lateralis Tensor fascia latae | Femoral Femoral Femoral Femoral Superior gluteal | L2–4 L2–4 L2–4 L2–4 L4–5 |
Internal rotation of flexed leg (non–weight bearing) | Popliteus Semimembranosus Semitendinosus Sartorius Gracilis | Tibial Sciatic Sciatic Femoral Obturator | L4–5 L5, S1–2 L5, S1–2 L2–3 L2–3 |
External rotation of flexed leg (non–weight bearing) | Biceps femoris | Sciatic | L5, S1–2 |
Data from Magee DJ. Orthopaedic Physical Assessment. 2nd ed. Philadelphia, PA: WB Saunders; 1992. |
Quadriceps
The four muscles that make up the quadriceps are the rectus femoris, the vastus intermedius, the VL, and the vastus medialis (Fig. 19-7). The quadriceps tendon represents the convergence of all four muscle–tendon units, and it inserts into the anterior aspect of the superior pole of the patella. The quadriceps muscle group is innervated by the femoral nerve. The quadriceps muscles can act to extend the knee when the foot is off the ground, although more commonly, they work as decelerators, preventing the knee from buckling when the foot strikes the ground.132,133
Rectus Femoris
The rectus femoris (see Fig. 19-7), which originates at the anterior inferior iliac spine (AIIS) is the only quadriceps muscle that crosses the hip joint. The other quadriceps muscles originate on the femoral shaft. This gives the hip joint substantial significance with respect to the knee extensor mechanism in the examination and intervention.133 The line of pull of the rectus femoris, with respect to the patella, is at an angle of about 5 degrees with the femoral shaft.133
Vastus Intermedius
The vastus intermedius (see Fig. 19-7) has its origin in the proximal part of the femur, and its line of action is directly in line with the femur.
Vastus Lateralis
The VL (Fig. 19-7) is composed of two functional parts: the VL and the vastus lateralis oblique (VLO).132 The VL has a line of pull of about 12–15 degrees to the long axis of the femur in the frontal plane, whereas the VLO has a pull of 38–48 degrees.133
Vastus Medialis
The vastus medialis (Fig. 19-7) is composed of two parts that are anatomically distinct132: the vastus medialis obliquus (VMO) and the vastus medialis proper, or longus (VML).134 The VML appears to have little biomechanical significance unlike its counterpart, the VMO.
Vastus Medialis Obliquus. The VMO arises from the adductor magnus tendon.135 The insertion site of the normal VMO is the medial border of the patella, approximately one-third to one-half of the way down from the proximal pole. If the VMO remains proximal to the proximal pole of the patella and does not reach the patella, there is an increased potential for malalignment.10
The vector of the VMO is medially directed, and it forms an angle of 50–55 degrees with the mechanical axis of the leg.132,135–137 The VMO is least active in the fully extended position138–140 and plays little role in extending the knee, acting instead to realign the patella medially during the extension maneuver. It is active in this function throughout the whole range of extension.
According to Fox,141 the vastus medialis is the weakest of the quadriceps group and appears to be the first muscle of the quadriceps group to atrophy and the last to rehabilitate.142 The normal VMO/VL ratio of electromyographic (EMG) activity in standing knee extension from 30 to 0 degrees is 1:1,143 but in patients who have patellofemoral pain, the activity in the VMO decreases significantly; instead of being tonically active, it becomes phasic in action.144 The presence of swelling also inhibits the VMO, and it requires almost half of the volume of effusion to inhibit the VMO as it does to inhibit the rectus femoris and VL muscles.145
The VMO is frequently innervated independently from the rest of the quadriceps by a separate branch of the femoral nerve.132
Vastus Medialis Longus. The VML originates from the medial aspect of the upper femur and inserts anteriorly into the quadriceps tendon, giving it a line of action of approximately 15–17 degrees off the long axis of the femur in the frontal plane.133
Since the quadriceps group is aligned anatomically with the shaft of the femur and not with the mechanical axis of the lower extremity, any quadriceps muscle contraction (regardless of knee-flexion angle) results in compressive forces acting on the PFJ.146
Hamstrings
As a group, the hamstrings primarily function to extend the hip and to flex the knee. The hamstrings are innervated by branches of the sciatic nerve.
Semimembranosus Muscle
The semimembranosus muscle (Fig. 19-8) arises from the lateral facet of the ischial tuberosity and the ischial ramus. This muscle inserts into the posteromedial aspect of the medial tibial condyle and has a key expansion that reinforces the posteromedial corner of the knee capsule. The semimembranosus pulls the meniscus posteriorly, and internally rotates the tibia on the femur, during knee flexion, although its primary function is to extend the hip and flex the knee.
Semitendinosus Muscle
The semitendinosus muscle (see Fig. 19-8) arises from the upper portion of the ischial tuberosity via a shared tendon with the long head of the biceps femoris. From there, it travels distally, becoming cord-like about two-thirds of the way down the posteromedial thigh. Passing over the MCL, it inserts into the medial surface of the tibia and deep fascia of the lower leg, distal to the gracilis attachment, and posterior to the sartorius attachment. These three structures are collectively called the pes anserinus (“goose foot”) at this point. Like the semimembranosus, the semitendinosus functions to extend the hip, flex the knee, and internally rotate the tibia.
Biceps Femoris
The biceps femoris (see Fig. 19-8) muscle is a two-headed muscle. The longer of the two heads arises from the inferomedial facet of the ischial tuberosity, whereas the shorter head originates from the lateral lip of the linea aspera of the femur. The muscle inserts on the lateral tibial condyle and the fibular head. The biceps femoris functions to extend the hip, flex the knee, and externally rotate the tibia. The superficial layer of the common tendon has been identified as the main force creating external tibial rotation and controlling the internal rotation of the femur.147 The pull of the biceps on the tibia retracts the joint capsule and pulls the iliotibial tract posteriorly, keeping it tight throughout flexion.
Gastrocnemius
The gastrocnemius originates from above the knee by two heads, each head connected to a femoral condyle and to the joint capsule (Fig. 20-4). Approximately halfway down the leg, the gastrocnemius muscles merge to form an aponeurosis. As the aponeurosis gradually contracts, it accepts the tendon of the soleus, a flat, broad muscle deep to the gastrocnemius. The aponeurosis and the soleus tendon end in a flat tendon called the Achilles tendon, which attaches to the posterior aspect of the calcaneus. The two heads of the gastrocnemius and the soleus are collectively known as the triceps surae (see Chapter 21).
FIGURE 20-4 Gastrocnemius muscle.
Although the primary function of the gastrocnemius–soleus complex is to plantar flex the ankle and to supinate the subtalar joint, the gastrocnemius also functions to flex or extend the knee, depending on whether the lower extremity is WB or not. It has been proposed that weakness of the gastrocnemius may cause knee hyperextension.148
In addition, it has been theorized that the gastrocnemius acts as an antagonist to the ACL, exerting an anteriorly directed pull on the tibia throughout the range of knee flexion–extension motion, particularly when the knee is near full extension.149,150
Popliteus
The popliteus originates from the lateral femoral condyle near the LCL. The muscle has several attachments, including the lateral aspect of the lateral femoral condyle, the posteriormedial aspect of the head of the fibula and the posterior horn of the lateral meniscus.151 The larger base of this triangular muscle inserts obliquely into the posterosuperior part of the tibia above the soleal line. The muscle has several important functions, including the reinforcement of the posterior third of the lateral capsular ligament69 and the unlocking of the knee during flexion from terminal knee extension. It performs this latter task by internally rotating the tibia on the femur (a good example of an arcuvial muscle), preventing impingement of the posterior horn of the lateral meniscus by drawing it posteriorly, and, with the PCL, preventing a posterior glide of the tibia.69,152–154 Since knee joint injury frequently involves some component of transverse-plane rotation and the popliteus muscle has been described as an important, primary, dynamic, transverse-plane, rotatory knee joint stabilizer, an understanding of its function in relation to other PL knee joint structures is important.155 Attached to the popliteus tendon is the popliteofibular ligament, which forms a strong attachment to the popliteal tendon and the fibula. This ligament adds to PL stability.156–159 A medial portion of the popliteus penetrates the joint, becoming intracapsular with the lateral meniscus. This part of the popliteus tendon is pain sensitive, and an injury here can often mimic a meniscal injury on the lateral aspect of the joint line.75 Differentiation between these two lesions can be elucidated with the reproduction of pain with resisted flexion in an extended and externally rotated position of the tibia if the popliteus is involved.
Hip Adductors
The hip adductors, which play an indirect role in the medial stability of the knee (Table 20-3), are described in Chapter 19. The exception to this is the two-joint gracilis muscle, the third member of the pes anserinus group, which in addition to adducting and flexing the hip, assists in flexion of the knee and internal rotation of the lower leg.
TABLE 20-3 | Hip Adductors Involved in Knee Stability |
Muscle | Proximal Attachment | Distal Attachment | Innervation |
Adductor longus | Pubic crest and symphysis | By an aponeurosis to middle third of linea aspera of femur | Obturator nerve, L3 |
Adductor magnus | Inferior ramus of pubis, ramus of ischium, and inferolateral aspect of ischial tuberosity | By an aponeurosis to linea aspera and adductor tubercle of femur | Obturator nerve and tibial portion of sciatic nerve, L2–4 |
Gracilis | Thin aponeurosis from medial margins of lower half of body of pubis, whole of inferior ramus, and joining part of ramus of ischium | Upper part of medial surface of tibia, below tibial condyle and just proximal to tendon of semitendinosus | Obturator nerve, L2 |
Tensor Fascia Latae
The tensor fascia latae (TFL) arises from the outer lip of the iliac crest and the lateral surface of the anterior superior iliac spine (ASIS) (Fig. 19-5). Over the flattened lateral surface of the thigh, the fascia latae thickens to form a strong band, the iliotibial tract. When the hip is flexed, the TFL is anterior to the greater trochanter and helps maintain the hip in flexion. As the hip extends, the TFL moves posteriorly over the greater trochanter to assist with hip extension. The TFL is also a weak extensor of the knee, but only when the knee is already extended. The muscle is innervated by the superior gluteal nerve, L4–L5.
Iliotibial Band (Tract)
The ITB or tract begins as a wide covering of the superior and lateral aspects of the pelvis and thigh in continuity with the fascia latae (Fig. 20-4 and Fig. 19-5). It inserts distal and lateral to the patella at the tubercle of Gerdy on the lateral condyle of the tibia. Anteriorly, it attaches to the lateral border of the patellar. Posteriorly, it is attached to the tendon of the biceps femoris. Laterally, it blends with an aponeurotic expansion from the VL.160 (see Chapter 19).
Like the patella tendon, the ITB can be viewed as a ligament or a tendon. Its location adjacent to the center of rotation of the knee allows it to function as an anterolateral stabilizer of the knee in the frontal plane161 and to both flex and extend the knee.133,162 During stationary standing, the primary function of the ITB is to maintain knee and hip extension, providing the thigh muscles an opportunity to rest. While walking or running, the ITB helps maintain flexion of the hip and is a major support of the knee in squatting from full extension until 30 degrees of flexion. In knee flexion greater than 30 degrees, the iliotibial tract becomes a weak knee flexor, as well as an external rotator of the tibia.
MAJOR NERVES AND BLOOD VESSELS
The posterior structure of the knee joint is a complex of nerves and blood vessels.
The knee joint is innervated by the posterior articular branch of the posterior tibial nerve, which is formed from all five anterior divisions (L4, L5, and S1–S3) of the sacral plexus (see below), and the terminal branches of the obturator and femoral nerves.78 The course and distribution of the femoral nerve are described in Chapter 3. The lateral portion of the capsule is innervated by the recurrent peroneal branch of the common fibular (peroneal) nerve, which is formed by the upper four posterior divisions (L4, L5, and S1, S2) of the sacral plexus (see below). The saphenous nerve is the largest cutaneous branch of the femoral nerve (L2–L4). It leaves the subsartorial canal approximately 8–10 cm above the medial condyle of the knee and gives off branches to the medial aspect of the knee. Entrapment of the saphenous nerve during its course here can occur because of direct trauma, genu valgus, or knee instability, resulting in saphenous neuritis. The sciatic nerve (see Chapter 3) provides motor branches to the hamstrings and all muscles below the knee.163 It also provides the sensory innervation to the posterior thigh and entire leg and foot below the knee (except the medial aspect, which is innervated by the saphenous nerve).163
The major blood supply to this area comes from the femoral (see Chapter 19), popliteal, and genicular arteries.
Popliteal. Before bifurcating into the anterior and posterior tibial arteries, the popliteal artery normally courses beneath and between the medial and lateral heads of the gastrocnemius, adjacent to the plantaris and popliteus muscles, and through the tendinous arch of the soleus.164,165 Alteration of these normal structural relationships can cause compression of the popliteal artery—popliteal artery entrapment syndrome (see Chapter 5).
Genicular. The descending genicular artery arises from the femoral artery, just before it passes through the adductor hiatus and immediately divides into the saphenous branch of descending genicular artery and the articular branches of descending genicular artery. The superior medial genicular artery and the superior lateral genicular artery both arise from the popliteal artery. The middle genicular artery is a small branch of the popliteal artery that originates inferior or distal to both of the superior genicular arteries. Arising from the middle genicular artery are the inferior medial and inferior lateral genicular arteries.
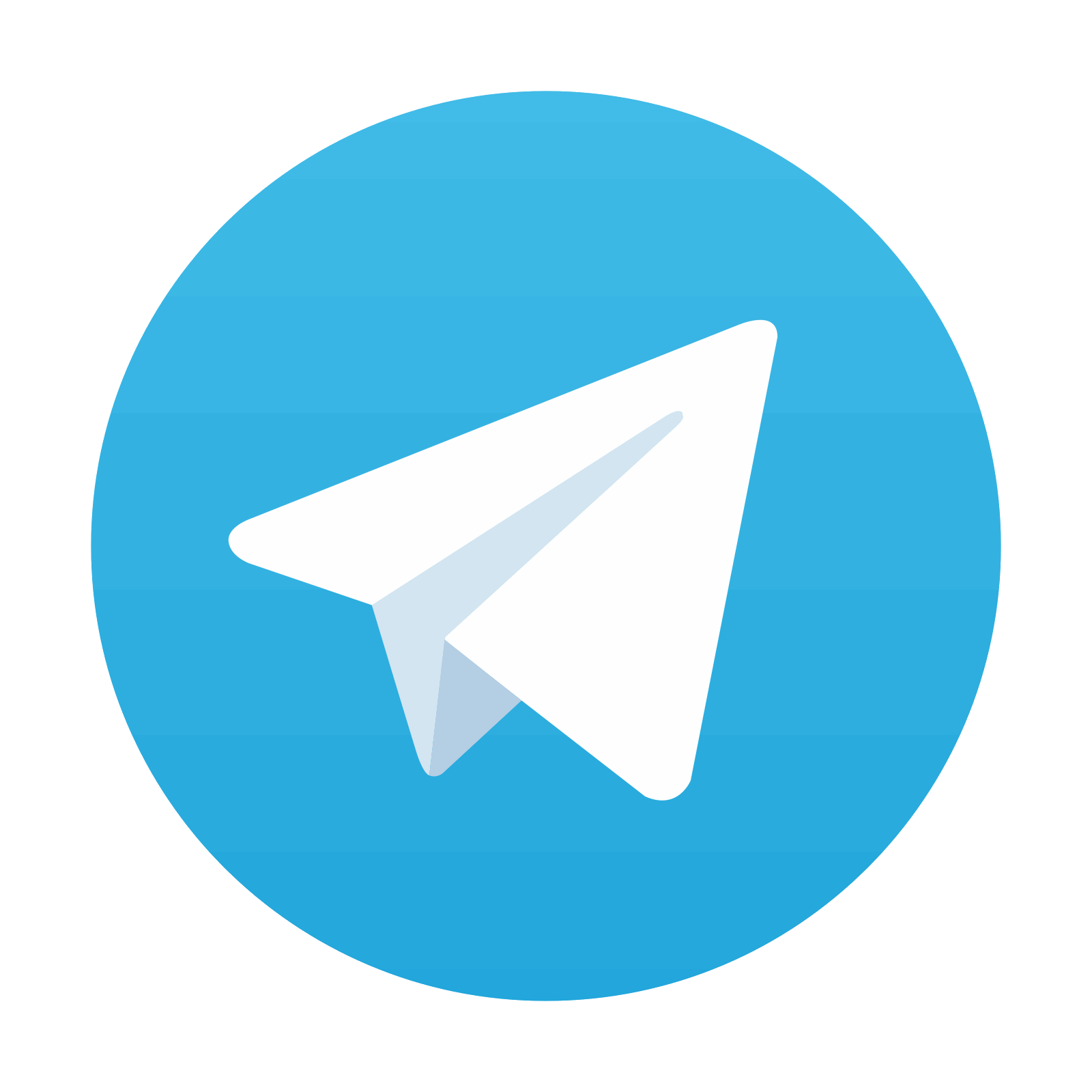
Stay updated, free articles. Join our Telegram channel

Full access? Get Clinical Tree
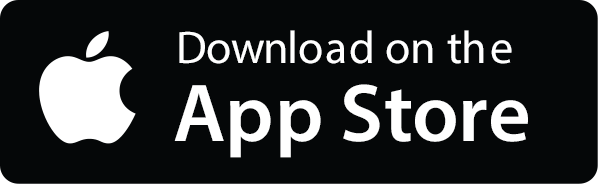
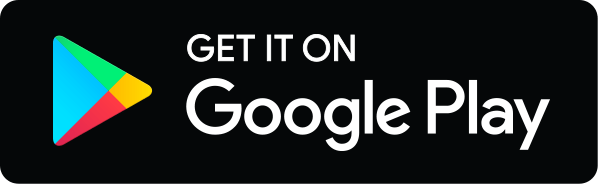
