An appreciation of the age-related physiologic variations in the shape of the foot
An understanding of the natural history of each variation and deformity
An appreciation of the effect of a chosen intervention on growth and development of the foot as well as the effect of growth and development on a chosen intervention
A thorough and working knowledge of the most unique “joint” in the human body, the subtalar joint complex, which is a combination of the talocalcaneal (subtalar) joint, plus the talonavicular and calcaneocuboid joints (transtarsal or Chopart joints)
The ability to obtain, and the commitment to evaluate, only weight-bearing or simulated weight-bearing radiographs
A dedication to preserving joint motion by utilizing softtissue releases and osteotomies instead of arthrodeses
A complete understanding of the phrase: “The foot is not a joint” (1)
from the normal shapes of the child to those of the adult through normal growth and development. Externally applied forces cannot modify these physiologic shape variations of the long bones. And the long-term health consequences of persistent physiologic variations of the long bones have yet to be proven.
TABLE 29-1 Causes of Cavus Foot Deformity | ||||||||||||||||||||||||||||||||
---|---|---|---|---|---|---|---|---|---|---|---|---|---|---|---|---|---|---|---|---|---|---|---|---|---|---|---|---|---|---|---|---|
|
plantar soft tissues become contracted. The incompletely ossified bones change shape due to excessive compression on their plantar aspects (Hueter-Volkmann law). The normal tripod structure of the foot becomes unbalanced. Bearing weight on the plantar-flexed first metatarsal causes the forefoot to supinate in relation to the tibia, thereby allowing the fifth metatarsal head to touch the ground. Because the forefoot is rigidly pronated in relation to the hindfoot, the subtalar joint is thereby driven into inversion, or varus (48) (Fig. 29-3). This flexible hindfoot varus deformity eventually becomes rigid as the plantar-medial soft tissues of the subtalar joint contract. The cavovarus foot, therefore, has two major rotational deformities in opposite directions from each other: pronation of the forefoot and supination (varus and inversion are other descriptive terms) of the hindfoot. It appears as if the foot is wrung out (1). Determination of the flexibility or rigidity of each deformity is important when planning an operation. Flexible deformities are treated with tendon transfers, and inflexible deformities are treated with soft-tissue releases, osteotomies, and, occasionally, arthrodeses. Coleman and Chestnut (50) devised the block test to help evaluate the flexibility of the hindfoot (Fig. 29-4). The patient stands with a block of wood under the lateral border of the foot to recreate the tripod while allowing the first metatarsal to plantar-flex. A flexible varus deformity of the hindfoot will correct to valgus alignment. One that is already contracted and rigid will not. In the first situation, surgery for deformity correction is confined to the forefoot. In the latter case, forefoot and hindfoot procedures are needed.
![]() FIGURE 29-3. The tripod effect. The hindfoot must assume a varus position when weight bearing if the first metatarsal is fixed in plantar flexion (9). Initial contact of plantar-flexed first metatarsal (4). Fifth metatarsal makes contact through supination of the forefoot (arrow), which also drives the hindfoot into varus. (From Paulos L, Coleman SS, Samuelson KM. Pes cavovarus. Review of a surgical approach using selective soft-tissue procedures. J Bone Joint Surg Am 1980;62: 942-953, with permission.) |
with DNA blood tests for CMT, electromyogram with nerve conduction studies, and muscle biopsy may be indicated.
of the foot. The forefoot becomes rigidly pronated in relation to the hindfoot. The tripod effect (48) accounts for the varus position that the hindfoot must assume during weight bearing due to the fixed pronation of the forefoot. Also contributing to the varus deformity of the hindfoot is the muscle imbalance between the tibialis posterior, an invertor of the subtalar joint, that remains strong and the peroneus brevis, an evertor of the subtalar joint, that becomes weak (47). The subtalar joint eventually becomes rigidly deformed in varus because of contracture of the plantar-medial soft tissues, including those of the subtalar joint complex. Although the triceps surae does not become contracted in CMT, it does in some of the other diseases that cause cavus.
![]() FIGURE 29-11 Superficial plantar-medial release. A: The abductor hallucis muscle has 3 origins on the medial surface of the calcaneus (labeled 1, 2, and 3 from plantar to dorsal). The posterior tibial neurovascular bundle (with white vessel loop around it) divides into medial and lateral plantar neurovascular bundles immediately before passing into the muscle. The laciniate ligament (flexor retinaculum) has been incised vertically in line with the NV bundles to expose the bundles. The plantar fascia is seen as a white band of dense collagen plantar-lateral to the abductor hallucis. It is sharply separated from the thick layer of plantar fat. B: After release of the lowest/largest origin of the abductor hallucis muscle (1) and the plantar fascia and short toe flexors, the lateral plantar neurovascular bundle can be seen traversing the foot in a distal-lateral direction. Release of those soft tissues using the tunnel of the NV bundle for guidance obviates injury to those important structures. C: The thin septum (and 2nd origin) of the abductor hallucis that separated the medial and lateral plantar NV bundles is divided under direct vision. D: The most dorsal origin (3) of the abductor hallucis, which is dorsal to the medial plantar NV bundle, is released. This completes a superficial plantar-medial release for a cavovarus foot deformity with flexible hindfoot varus. (From the private collection of Vincent S. Mosca, MD.) |
means congenital absence of all or part of a central digit, is an acceptable synonym.
However, in many cases of cleft foot, there is a marked increase in the width of the forefoot (Fig. 29-24), making it difficult or impossible to find shoes that fit comfortably. Painful callosities develop over the medial and lateral metatarsal heads.
![]() FIGURE 29-27. Preoperative (A) and postoperative (B) radiographs of symptomatic cleft foot treated with osteotomies. (From the private collection of Vincent S. Mosca, MD.) |
logistic model was used by Rebbeck et al. (99) to conclude that the probability of having clubfoot was explained by the mendelian segregation of a single gene with two alleles plus the effects of some other factors that are yet to be elucidated.
TABLE 29-2 Syndromes with Which Clubfoot Is Commonly Associated | ||||||||||
---|---|---|---|---|---|---|---|---|---|---|
|
![]() FIGURE 29-29. Clubfoot (left) with single heel crease and healthy foot (right) with multiple heel creases. (From the private collection of Vincent S. Mosca, MD.) |
The severity and rigidity of the deformities
Depth of the skin creases (Fig. 29-29)
Tightness and contractility of the muscles
is currently limited. Arthrography, computerized tomography (138), and magnetic resonance imaging (127, 139, 140) may have a role in research or in the evaluation of postsurgical deformities, but do not have a role in the routine assessment of the idiopathic clubfoot.
hip joint. Its bony elements are the posterior articular surface of the navicular and the anterior and posterior articular facets of the calcaneus. Epeldegui found that the soft tissues of the AP were likewise markedly different in shape and orientation in the clubfoot from the normal foot. The shape of the medial cuneiform has not been studied in the newborn, but it is trapezoid shaped in the older child with residual forefoot adductus deformity. The subtalar joint complex is severely inverted, a combination of internal rotation and plantar flexion. The axis of rotation is in the interosseus talocalcaneal ligament. The AP is inverted around the plantar-medially-deviated head and neck of the talus, thereby aligning the navicular at or near the medial malleolus. The calcaneus is rotated downward and inward resulting in parallel alignment with the talus in the frontal and sagittal planes. The posterior part of the calcaneus is tethered to the fibula by the calcaneofibular ligament. There is a varus deformity of the distal end of the calcaneus with medial deviation of a congruous calcaneocuboid joint in many clubfeet (78, 139, 140, 153, 155, 156, 157, 158 and 159, 161). There may be medial subluxation of the cuboid on the distal calcaneus in some feet (152, 162). The plantar fascia, short plantar muscles, and spring ligament are contracted. The Achilles, tibialis posterior, flexor hallucis longus, and flexor digitorum communis tendons are contracted. The posterior capsules of the ankle and talocalcaneal joints are contracted.
![]() FIGURE 29-33. Navicular (1). The axis of the anterior facet of the calcaneus (2) is tilted medially in relation to the axis of the middle facet (3) in the clubfoot (image on left) compared with the normal foot (image on right). (From Epeldegui T, Delgado E. Acetabulum pedis. Part I: talocalcaneonavicular joint socket in normal foot. J Pediatr Orthop B 1995;4:9, with permission.) |
produce a significant component of the clubfoot pathology. Sano et al. (166) confirmed these findings, showing that cells of the medial ligamentous structures contained vimentin uniformly and myofibroblasts in some cases. More recently, Khan et al. (167) were unable to show myofibroblast-like cells in clubfeet, and van der Sluijs and Pruys (168) demonstrated normal collagen cross-linking in clubfeet.
![]() FIGURE 29-34. Untreated clubfoot in an adult migrant farm worker. (From the private collection of Vincent S. Mosca, MD.) |
radiographic result. This study, along with subsequent studies from Iowa and elsewhere, has established the Ponseti method as the gold standard for conservative management of clubfoot not only in the United States but around the world.
![]() FIGURE 29-39. With each cast, the thigh-foot angle is progressively increased toward its final position of 70 degrees of external rotation. (From the private collection of Vincent S. Mosca, MD). |
![]() FIGURE 29-41. The percutaneous Achilles tenotomy is performed in the outpatient clinic under local anesthetic if dorsiflexion is limited (A). An assistant holds the leg at the knee and at the forefoot. The heel and lower portion of leg are given a generous Betadine prep. A small amount of local anesthetic is injected with a 25- to 27-gauge needle medial to the tendo Achilles. Large volumes of the anesthetic should be avoided because this can obscure palpation of the tendon. Alternatively, a Xylocaine cream can be applied to the posterior ankle region before the tenotomy to avoid obscuring the tendon with the injected anesthetic. The liquid local anesthetic can be injected immediately following the tenotomy in this scenario. At a position approximately 1 cm above the insertion of the Achilles tendon on the calcaneus, a small cataract knife or narrow Beaver blade is inserted from the medial side of the heel perpendicular to the medial border of the foot, with the blade parallel to the Achilles tendon and directed at the tendon. Upon encountering the tendon, the blade is slowly moved anteriorly until it slips past the anterior border of the tendon. This technique will help ensure that the blade does not pass near the posterior tibial neurovascular bundle. The knife blade is then turned to a 90-degree angle posteriorly and the tendon sectioned. The knife blade should not go through the skin posteriorly. The release that is obtained after complete tenotomy is easily felt, and 10 to 20 degrees of additional dorsiflexion are easily obtained (B). The skin is then cleaned with alcohol to remove the Betadine. A small square of Adaptic or Xeroform gauze can be placed over the wound and covered by sterile gauze. With the final cast applied, the foot should be in 20 degrees of dorsiflexion and 70 degrees of abduction (see Fig. 29-38 and Fig. 29-39). This final cast is worn for 3 weeks. |
extensive soft-tissue releases. Many were found to have poor foot function long-term. Most feet underwent one or more repeat surgical soft-tissue releases, and these feet were characterized by pain, stiffness, and arthritis. These young adults experienced a significantly impaired quality of life.
order, addressing only those deformities that have not responded to the nonoperative treatment that has been employed.
![]() FIGURE 29-44. Surgical Correction of Clubfoot. The incisions used for clubfoot surgery vary widely and are more numerous than can be described here. All have been used successfully, but what is done beneath the incision is far more important to the result than the incision itself. Turco (175) described a straight incision that ran from the base of the first metatarsal, under the medial malleolus, until it reached the Achilles tendon (A). He pointed out that a proximal extension of the incision along the Achilles tendon was contraindicated and that no undermining of the wound should be done. Ignoring these two admonitions has led to many wound problems. Crawford et al. (219) described an incision popularized by Giannestras in Cincinnati (B). This transverse incision begins on the medial side of the foot, over the naviculocuneiform joint. From there, the incision passes posteriorly to cross just beneath the tip of the medial malleolus. It continues across the back of the ankle at least 1cm proximal to the posterior heel crease and continues laterally to pass under the lateral malleolus, ending at the sinus tarsi. Although some surgeons have abandoned this incision because of wound complications, many more report using it routinely without problems. It is my incision of choice. Some surgeons prefer to use two incisions: one posterior and one medial, with a third incision laterally over the calcaneocuboid joint, if this is necessary. Carroll (152) has described a medial incision with three limbs (C). The center of the calcaneus, the front of the medial malleolus, and the base of the first metatarsal form a triangle. The center part of this incision is parallel to the base of the triangle, whereas the proximal part angles toward the center of the heel and the distal part crosses over the dorsum of the foot. The posterior incision (not shown) runs from a point in the midline about 4 cm above the tibiotalar joint obliquely to a point midway between the Achilles tendon and the lateral malleolus. |
![]() FIGURE 29-50. Although many illustrations of clubfoot surgery show the ligaments of the posterior capsule as distinct structures, the surgeon rarely sees them this way because they are merely condensations of the continuous posterior capsule. Occasionally, the posterior talofibular ligament and the calcaneofibular ligament stand out, the latter appearing like a tendon. The geographic cuts in the posterior capsule of the tibiotalar and subtalar joints divide the ligaments as shown: the posterior tibiotalar ligament (A), the posterior talofibular ligament (B), the tibiofibular ligament (C), the calcaneofibular ligament (D), and the deltoid ligament (E). The deltoid ligament consists of several parts. One part of the deltoid ligament, referred to as the deep deltoid ligament (anterior tibiotalar part of the deltoid ligament), is attached to the talus and, in the opinion of many surgeons, should not be divided in order to avoid the complication of lateral subluxation of the talus. Division of this part of the deltoid ligament is avoided by limiting the capsulotomy of the tibiotalar joint up to the posterior aspect of the medial malleolus. If it is desired to divide this portion of the deltoid ligament as a part of the operation, as is done in the procedure described by Goldner (191), it should be repaired. |
![]() FIGURE 29-51. A: The plantar-medial release is performed through the antero-medial extension of a Cincinnati incision. A vessel loop surrounds the posterior tibial neurovascular bundle (blue arrow) posterior to the medial malleolus. The proximal edge (black arrow) of the laciniate ligament (a.k.a. flexor retinaculum) is exposed. B: The laciniate ligament is released with scissors. C: The lowest (1) of the 3 origins of the abductor hallucis muscle is released from the calcaneus superficial to the lateral plantar neurovascular bundle. D: The plantar fascia and short toe flexors are next released superficial (plantar) to the lateral plantar neurovascular bundle. Release of those soft tissues using the tunnel of the NV bundle for guidance obviates injury to those important structures. E: The thin septum (2) of the abductor hallucis that separates the medial and lateral plantar NV bundles is divided under direct vision. F: The most dorsal origin (3) of the abductor hallucis, which is dorsal to the medial plantar NV bundle, is released. This completes the superficial plantar-medial release. |
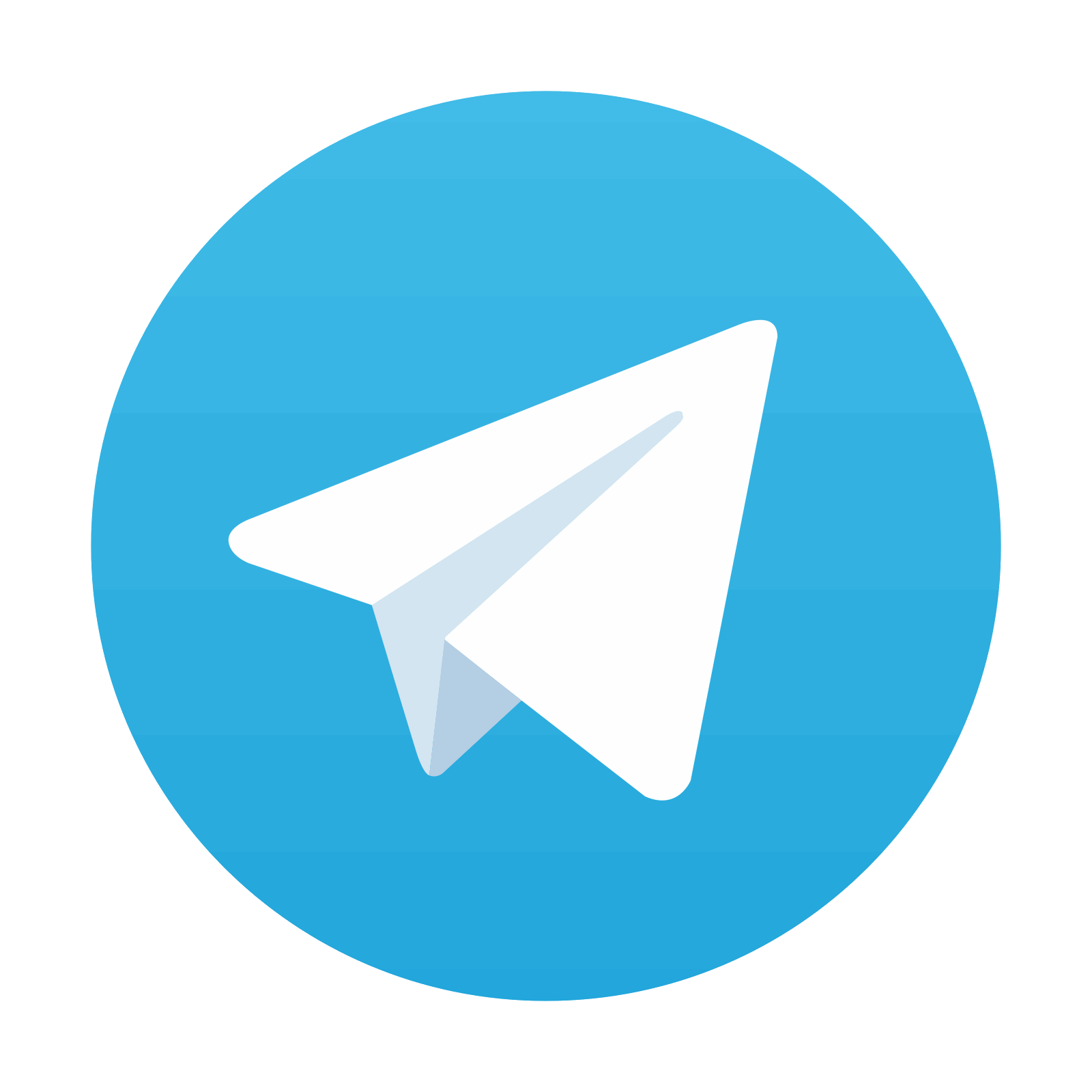
Stay updated, free articles. Join our Telegram channel

Full access? Get Clinical Tree
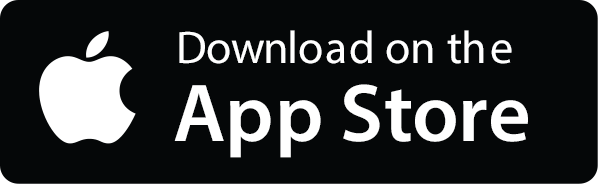
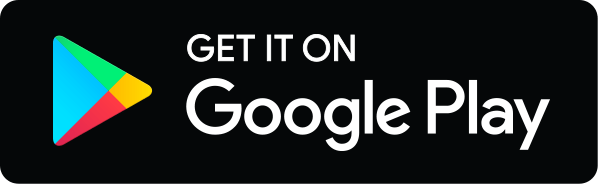
