The craniovertebral region is a collective term that refers to the occiput, atlas, axis, and supporting ligaments, which accounts for approximately 25% of the vertical height of the entire cervical spine. Injuries to this region have the potential to involve the brain, brain stem, and spinal cord, resulting in a myriad of symptoms ranging from headache and vertigo to cognitive and sympathetic system dysfunction.1 Craniovertebral injuries that involve cognitive and sympathetic system dysfunction demonstrate a poorer prognosis and a lengthy recovery. The craniovertebral region is considered as a separate entity from the rest of the cervical spine because of its distinct embryology and anatomical structure. Kapandji2 notes that the occiput, atlas, and axis actually form a primary kyphotic curve and that this curve serves as a delineation between the craniovertebral region and the cervical spine proper.
CHAPTER 23
The Craniovertebral Region
OVERVIEW
ANATOMY
Foramen Magnum
The general shape of the foramen magnum is oval, with the longer axis oriented in the sagittal plane.3 The margin of the foramen is relatively smooth and serves as the most superior attachment for a variety of the ligaments of the vertebral column. The smaller anterior region of the foramen magnum is characterized by a pair of tubercles to which the alar ligaments attach. The posterior portion of the foramen magnum houses the brain stem–spinal cord junction.
On either side of the anterolateral aspect of the foramen magnum are two ovoid projections, called occipital condyles. The long axis of these paired occipital condyles is situated in a posterolateral to anteromedial orientation. The occipital condyles articulate with the first cervical vertebra.
Atlas
The atlas is a ring-like structure that is formed by two lateral masses, which are interconnected by anterior and posterior arches (Fig. 23-1A). The demarcation of the two regions is marked by a pair of tubercles to which the transverse ligament of the atlas attaches. Although the atlas has a smaller vertical dimension than any other cervical vertebrae, it is considerably wider. Because this vertebra does not have a spinous process, there is no bone posteriorly between the occipital bone and the spinous process of C2. This results in an increase in the potential for craniovertebral extension.
FIGURE 23-1 Bony structures of the craniovertebral joints. A. The atlas (C1). B. The axis.
The superolateral aspect of each of the posterior arches has a transverse foramen to accommodate the vertebral artery (see Chapter 24). The articular surface of the inferior facet is circular and relatively flat and slopes inferiorly from medial to lateral. The upper articular facets of C1 are elongated from anterior to posterior, with the anterior ends closer together and more upwardly curved than their posterior counterparts.3 This arrangement results in much more extension than flexion being available at the articulation between the occipital condyles and the atlas—the occipitoatlantal (O-A) joint.4
O-A Joint
The O-A joint represents the most superior zygapophyseal joint of the vertebral column and the only vertebral level that has an articulation characterized by a convex surface (occipital condyle) moving on a concave joint partner (articulating facet of the atlas).5
Axis
The axis serves as a transitional vertebra (Fig. 23-1B) because it is the link between the cervical spine proper and the craniovertebral region.
The dimensions of the axis are significantly different from those of the atlas. The atlas is considerably wider than the axis, but because of the long spinous process, the axis extends much farther posteriorly and is the first palpable midline structure below the occiput.6 Like the atlas, the transverse process of the axis has a transverse foramen to allow passage of the vertebral artery (not shown).
A unique feature of the axis is the odontoid process, or dens (Fig. 23-2B). The dens, which develops with age, extends superiorly from the body to just above the C1 vertebra, before tapering to a blunt point (Fig. 23-2). Very dense, thick trabecular bone is present in the center of the tip of the dens, and the cortical bone at the anterior base of the body of C2 (where the anterior longitudinal ligament attaches) is uniformly thick.7 Hypodense bone, however, is present consistently beneath the odontoid process at the upper portion of the body of C2.8 This area of hypodense bone is susceptible to fracture. The anterior aspect of the dens has a hyaline cartilage-covered mid-line facet for articulation with the anterior tubercle of the atlas (the median atlantoaxial (A-A) joint). The posterior aspect of the dens usually is marked with a groove where the transverse ligament passes. The dens functions as a pivot for the upper cervical joints and as the center of rotation of the A-A joint. The most variable dimension of the axis is the dens angle in the sagittal plane, which can range from –2 degrees (leaning slightly anterior) to 42 degrees (leaning posterior).9 This extremely variable angle can make assessment of fracture reduction challenging.7
FIGURE 23-2 The dens and its relationship with the ligamentous structures of the craniovertebral region.
A-A Joint
This is a relatively complex articulation, which consists of
two lateral zygapophyseal joints between the articular surfaces of the inferior articular processes of the atlas and the superior processes of the axis;
two medial joints: one between the anterior surface of the dens of the axis and the anterior surface of the atlas, and the other between the posterior surface of the dens and the anterior hyalinated surface of the transverse ligament6 (Fig. 23-2).
The relatively large superior articular facets of the axis (Fig. 23-1B) lie lateral and anterior to the dens. These facets slope considerably downward from medial to lateral in line with the zygapophyseal facets of the mid-low cervical spine.10 As the lateral A-A joints function to convey the entire weight of the atlas and the head to lower structures, the lamina and pedicles of the axis are quite robust. The stout, moderately long spinous process serves as the uppermost attachment for muscles that are essentially lower cervical in nature and for muscles that act specifically on the craniovertebral joints.
One of the functions of the intervertebral disk (IVD) in the spine is to facilitate motion and provide stability. Thus, in the absence of an IVD in this region, the supporting soft tissues of the joints of the upper cervical spine must be lax to permit motion, while simultaneously being able to withstand great mechanical stresses.
Craniovertebral Ligaments
The craniovertebral region is noted for a variety of ligaments, some of which have been the focus of a number of clinical tests to determine their efficiency in preventing unwanted and potentially dangerous movements. The controlling structures for these segments, which must be considered together, are as follows:
Capsule and accessory capsular ligaments. The lateral capsular ligaments (anterolateral O-A ligament) of the O-A joints are typical of synovial joint capsules. These ligaments run obliquely from the basiocciput to the transverse process of the atlas. By necessity, they are quite lax, to permit maximal motion, so they provide only moderate support to the joints during contralateral head rotation.
Apical ligament. The apical ligament of the dens extends from the apex of the dens to the anterior rim of the foramen magnum. The ligament is short and thick. It runs from the top of the dens to the basiocciput and is thought to be a remnant of the notochord. The apical ligament appears to be only a moderate stabilizer against posterior translation of the dens relative to both the atlas and the occipital bone.
Vertical and transverse bands of the cruciform ligament. See Fig. 23-2 and later discussion.
Alar and accessory alar ligaments. See Fig. 23-2 and later discussion.
Anterior O-A membrane. The anterior O-A membrane is thought to be the superior continuation of the anterior longitudinal ligament. It connects the anterior arch of vertebra C1 to the anterior aspect of the foramen magnum.
Posterior O-A membrane. The posterior O-A membrane is a continuation of the ligamentum flavum. This ligament interconnects the posterior arch of the atlas and the posterior aspect of the foramen magnum and forms part of the posterior boundary of the vertebral canal.
Tectorial membrane. The tectorial membrane is the most superficial of the three membranes and interconnects the occipital bone and the axis. This ligament is the superior continuation of the posterior longitudinal ligament and connects the body of vertebra C2 to the anterior rim of the foramen magnum. This bridging ligament is an important limiter of upper cervical flexion and holds the occiput off the atlas.11
A-A Ligaments
The anterior A-A ligament is continuous with the anterior O-A membrane above.12 The posterior A-A ligament interconnects the posterior arch of the atlas and the laminae of the axis.
Occipitoaxial Ligaments
The O-A ligaments are very important to the stability of the upper cervical spine.
Alar Ligament
The alar ligaments (see Fig. 23-2) connect the superior part of the dens to fossae on the medial aspect of the occipital condyles, although they can also attach to the lateral masses of the atlas.13,14 A study of 44 cadavers15 found the orientation of the ligament to be superior, posterior, and lateral. In another study,13 19 upper cervical spine specimens were dissected to examine the macroscopic and functional anatomy of alar ligaments. The study found that the most common orientation (10/19) was cauda-cranial, followed by transverse (5/19). In two of the specimens, a previously undescribed ligamentous connection was found between the dens and the anterior arch of the atlas, the anterior atlantodental ligament. In 12 specimens, the ligament also attached via caudal fibers to the lateral mass of the atlas. The posteroanterior orientation of the ligaments in 17 of the 19 subjects was directly lateral from the dens to the occipital attachment or slightly posterior.
The function of the ligament is to resist flexion, contralateral side bending, and contralateral O-A rotation.16 In addition, the alar ligaments restrict anteroposterior translation of the occiput on C1 to some degree.17 Because of the connections of the ligament, side bending of the head produces a contralateral or ipsilateral rotation of C2, depending on the source.18
The Cruciform Ligament
The cruciform (cross-shaped) ligament has superior, inferior, and transverse portions (Fig. 23-2). The superior and inferior portions of this ligament attach to the posterior aspect of the body of the dens and the anterior rim of the foramen magnum. The transverse portion, which stretches between tubercles on the medial aspects of the lateral masses of the atlas, connects the atlas with the dens of the axis. The transverse portion of the ligament is so distinct and important that it is often considered a separate ligament (Fig. 23-2). The major responsibility of the transverse ligament is to counteract anterior translation of the atlas relative to the axis, thereby maintaining the position of the dens relative to the anterior arch of the atlas.11
The transverse ligament also limits the amount of flexion between the atlas and axis.19 These limiting functions are of extreme importance because the excessive movement of either type could result in the dens compressing the spinal cord, epipharynx, vertebral artery, or superior cervical ganglion. The integrity of the transverse ligament is also essential to the stability of atlas fractures; degenerative, inflammatory, and congenital disorders; and other abnormalities that affect the craniovertebral region.
The importance of the ligament is reflected in its physical properties. Spontaneous or isolated traumatic tears of this ligament are extremely rare events. The ligament is comprised almost entirely of collagen, with a parallel orientation close to the atlas and the dens, but with an approximately 30-degree obliquity at other points in the ligament. Dvorak et al.20 found the transverse ligament to be almost twice as strong as the alar ligaments and to have a tensile strength of 330 Newtons (N) (33 kg/73 lb).
Craniovertebral Muscles
Anterior Suboccipital Muscles
Rectus Capitis Anterior. The rectus capitis anterior (RCA) runs vertically. It travels deep to the longus capitis from the anterior aspect of the lateral mass of the atlas to the inferior surface of the base of the occiput, anterior to the occipital condyle. The RCA flexes and minimally rotates the head. Thus, an adaptively shortened RCA on the right could feasibly produce a decreased left translation in extension during mobility testing of the O-A joint. The muscle is supplied by the anterior (ventral) rami of C1 and C2.
Rectus Capitis Lateralis. This muscle arises from the superior surface of the C1 transverse process and inserts into the inferior surface of the jugular process of the occiput. It is homologous to the posterior intertransverse muscle of the spine. The rectus capitis lateralis side bends the head ipsilaterally. It is supplied by the anterior (ventral) rami of C1 and C2.
Posterior Suboccipital Muscles. The posterior suboccipitals lie beneath the splenius capitis and trapezius muscles. These muscles function in the control of segmental sliding between C1 and C221 and may have an important role in proprioception, having more muscle spindles than any other muscle for their size.21 All of the posterior suboccipital muscles are innervated by the posterior ramus of C1 and are also strongly linked with the trigeminal nerve.22,23 The suboccipitals receive their blood supply from the vertebral artery.
Rectus Capitis Posterior Major. The rectus capitis posterior major is the largest of the posterior suboccipitals. It runs from the C2 spinous process, widening as it runs cranially to attach to the lateral part of the inferior nuchal line (Fig. 23-3). Located inferior and lateral to the occipital protuberances, the rectus capitis posterior major muscles, when working together, extend the head. When working individually, the muscles produce ipsilateral side bending and rotation of the head.
FIGURE 23-3 Muscles of the craniovertebral joints.
Rectus Capitis Posterior Minor. The rectus capitis posterior minor is a small unisegmental muscle that runs from the posterior arch tubercle of the atlas to the medial part of the inferior nuchal line (see Fig. 23-3). Because of the shortness of the atlantean tubercle, the muscle is very horizontal, running almost parallel with the occiput. The muscle functions to extend the head and provides minimal support during ipsilateral side bending of the head.
Obliquus Capitis Inferior. This is the larger of the two oblique muscles and runs from the spinous process and lamina of the axis superolaterally to the transverse process of the atlas (see Fig. 23-3).
The inferior oblique muscle works to produce ipsilateral rotation of the atlas and skull and to control anterior translation and rotation of C1 (atlas). An adaptively shortened right inferior oblique may exert an inferior and posterior pull on the right transverse process of the atlas, producing a right rotated A-A joint.25,26 This results in a gross limitation of left rotation of the head while in cervical flexion, but a minimal limitation of left rotation of the head in extension.
Obliquus Capitis Superior. The superior oblique arises from the transverse process of the atlas and runs superoposterior and medially to the bone between the superior and inferior nuchal lines, lateral to the attachment of rectus capitis posterior major (see Fig. 23-3). Because of its posteromedial orientation, the superior oblique functions to provide contralateral rotation and ipsilateral side bending of the O-A joint, when acting unilaterally. When working together, the two superior obliques can produce an extension of the head. Dysfunction of this muscle has been implicated as a common cause of chronic headaches.27–31
The posterior suboccipital muscles can work concentrically with the larger extensors and rotators of the cervical spine, or eccentrically, controlling the action of the flexors. Because two of these muscles parallel the occiput, their controlling influence may be more linear than angular, producing or guiding the arthrokinematic, rather than the osteokinematic, motion.21
Nerve Supply
The posterior (dorsal) ramus of spinal nerve C1, when present (see Chapter 3), is larger than the anterior (ventral) ramus. It exits from the spinal canal by passing posteriorly between the posterior arch of the atlas and the rim of the foramen magnum, along with the vertebral artery. It then enters the suboccipital triangle and supplies most of the muscles that form that triangle. It usually has no cutaneous distribution.
The posterior (dorsal) ramus of spinal nerve C2 (see Fig. 23-3), also known as the greater occipital nerve, is larger than the anterior (ventral) ramus of C2. It exits from the vertebral canal by passing through a slit between the posterior arch of the atlas and the lamina of the axis. This nerve is the largest of the cervical posterior (dorsal) rami and is primarily a cutaneous nerve. It supplies most of the posterior aspect of the scalp, extending anteriorly to a line across the scalp that runs from one external auditory meatus to the other. Because this nerve has an extensive cutaneous distribution, it has a very large posterior (dorsal) root ganglion. This ganglion is commonly located in a vulnerable location, almost directly between the posterior arch of C1 and the lamina of C2 (see Fig. 23-3). The interval between these two bony structures is small, and it is reduced with the extension of the upper cervical spine. Given the sensitivity of the posterior (dorsal) root ganglion to compression, the possible relationship between the forward head position and occipital headaches is apparent.27–31 The O-A joints,32 A-A joints,33 and the C2 spinal nerve34 have all been implicated as primary nociceptors in cervicogenic headaches (CHs). Among individuals with chronic neck pain, 58–88% describe associated headaches.35,36 The prevalence of C2–3 zygapophyseal joint pain has been estimated at 50–53% of those patients with a chief complaint of headaches after whiplash injury.35,36
Blood Supply
The intradural vertebral artery supplies the most superior segments of the cervical spinal cord (see Chapter 24). The cervical cord is supplied by two arterial systems, central and peripheral, which overlap but are discrete. The first is dependent entirely on the single anterior spinal artery (ASA). The second, without clear-cut boundaries, receives supplies from the ASA and both posterior spinal arteries.37 Because the ASA is medial and dominant, unilateral spinal cord infarctions are very rare. However, they may occur in the perfusion territory supplied by the ASA.38,39 This is a result of obstruction of either a duplicated ASA39 or one of the sulcal arteries, which arise from the ASA and turn alternatively left or right to supply one side of the central cord.40 Peripheral hemicord infarction may result from ischemia, in the territory of the ASA38 or posterior spinal artery.41
BIOMECHANICS
The upper cervical spine is responsible for approximately 50% of the motion that occurs throughout the entire cervical spine. Motions at the O-A and A-A joints occur relatively independently, while, below C2, the normal motion is a combination of motion at other levels.
Articular facet asymmetry of the human upper cervical spine has been recognized for more than 30 years.42,43 The implications of this anatomic observation in the human spine has been considered in relation to joint disease, specifically facet tropism (the phenomenon observed in living tissue, of moving toward or away from a stimulus), which is associated with subsequent degenerative joint disease.44,45
O-A Joint
The deep atlantal sockets of the atlas facilitate flexion–extension but impede other movements. In living individuals, the average mean motion of flexion and extension at this joint is a combined range of 15–20 degrees.46 However, the variability in range of motion in the normal subject is large. Lind et al.47 reported a mean of 14 degrees with a standard deviation of 15 degrees in normal subjects. Side-bending motion occurs at an average of a little over 9 degrees to both sides,48–50 with an axial rotation range of 0 degrees (8 degrees when the movement was forced).49,50
Although it is generally agreed that rotation and side bending at this joint occur to opposite sides when they are combined, particular rules for patterns of defined coupled motion are not supported by the current literature.51
A-A Joint
The design of the ligamentous and bony structures at the A-A joint allow for a large arc of rotation.
The major motion that occurs at all three of the A-A articulations is an axial rotation. Werne48 reported 47 degrees to each side in cadavers. A more recent study using CT scanning observed 32 degrees (SD = 10) of axial rotation to either side.50 This amount of rotation has the potential to cause compression of the vertebral artery (see Chapter 24).52,53 To help prevent this compression, as the atlas rotates, the ipsilateral facet moves posteriorly while the contralateral facet moves anteriorly so that each facet of the atlas slides inferiorly along the convex surface of the axial facet, telescoping the head downward.
The first 25 degrees of head rotation (approximately 60%) occur primarily at the A-A articulations.54 However, the axial rotation of the atlas is not a pure motion, because it is coupled with a significant degree of extension (14 degrees) and, in some cases, flexion.55
In living individuals, the reported range of flexion–extension motion at this joint is highly variable, averaging 10 degrees.56 Flexion at the A-A joint is limited by the tectorial membrane. The extension is limited by the anterior arch of C1, as it makes contact with the odontoid process. The flexion and extension motions are associated with small anteroposterior translational movements, which can create a small space between the back of the anterior arch of the atlas and the odontoid. This space is called the atlanto-odontoid interval (ADI). Because of the arthrokinematics of the joint, flexion increases the ADI, whereas extension decreases it. Excessive gapping of the ADI can have serious consequences as it can lead to a compression of the spinal cord by the atlas.57 An ADI of more than 3 mm in adults, and 4.5 mm in children younger than 12 years of age, detected on radiographs is indicative of gross instability and is strongly suggestive of a compromise of the transverse ligament.57 An increased ADI can be associated with a history of trauma but may also be associated with severe ligamentous laxity in patients with rheumatoid arthritis (RA), neoplastic disease, Down’s syndrome, and aplasia or dysplasia of the dens.18
Side bending at this joint is approximately 5 degrees. Although coupling at this joint is commonly cited as contralateral side bending during rotation, it is highly variable because of the passive nature of the kinematics of the atlas.58 Whether the atlas flexes or extends during axial rotation depends on the geometry of the A-A joint and the precise direction of any forces acting through the atlas from the head.58 The direction of the conjunct rotation appears to be dependent on the initiating movement.59,60 If the initiating movement is side bending (latexion), the conjunct rotation of the joint is to the opposite side. If the initiating movement is a rotation (rotexion), the conjunct motion (side bending) is to the same side.59,60 If correct, this principle can be exploited in the assessment of the craniovertebral joints:
- Rotexion. During rotation of the head to the right (rotexion):
- Left side bending and right rotation occur at the O-A joint, accompanied by a translation to the right.
- Right side bending and right rotation occur at the A-A joint and at C2–3.
In other words, if the head motion is initiated with rotation, ipsilateral side bending of the A-A joint and C2–3 occurs, whereas at the O-A joint, contralateral side bending occurs.
- Left side bending and right rotation occur at the O-A joint, accompanied by a translation to the right.
- Latexion. Side bending of the head to the right produces
- left rotation of the O-A joint, accompanied by a translation of the occiput to the left;
- left rotation of the A-A joint;
- right rotation of C2–3.
- left rotation of the O-A joint, accompanied by a translation of the occiput to the left;
In other words, if head motion is initiated with side bending, contralateral rotation of both the O-A and A-A joints occurs, but ipsilateral rotation occurs at C2–3.
EXAMINATION
The primary objective of the examination of this region is to rule out any serious injury, especially if the patient reports any recent trauma to the head or neck. The pain distribution from cervical structures is outlined in Table 23-1, and the differential diagnoses are described in Chapter 5. The initial examination should focus on the general appearance of the patient (including skin lesions such as rashes), vital signs (pulse, blood pressure, and temperature), and the presence of any upper motor neuron signs or symptoms.62 Once serious injury has been ruled out, a biomechanical assessment of the craniovertebral joints can be performed. In addition, because of their close relationship with the craniovertebral joints, the cervical spine (see Chapter 25) and temporomandibular joint (see Chapter 26) should be assessed as part of a comprehensive examination of this region.
TABLE 23-1 | Pain Distribution from Cervical Structures |
Structure | Pain Area |
Occipital condyles | Frontal |
Occipitocervical tissues | Frontal |
C1 posterior (dorsal) ramus | Orbit, frontal, and vertex |
C1–2 | Temporal and suboccipital |
C3 posterior (dorsal) ramus | Occiput, mastoid, and frontal |
Data from Meadows J. A Rationale and Complete Approach to the Sub-Acute Post-MVA Cervical Patient. Calgary, AB: Swodeam Consulting; 1995. |
The biomechanical examination of the craniovertebral joints is best performed in a sequential manner (Fig. 23-4). In general, the O-A joint is examined and treated before the A-A joint to avoid confusion between findings from the combined testing of both joints. The examination and any intervention are terminated if any serious signs and/or symptoms are produced. In these instances, an appropriate referral is made.
FIGURE 23-4 Craniovertebral examination algorithm.
History
The craniovertebral region, as with the rest of the neck, is a common area for myofascial pain syndromes. These syndromes are frequently associated with complaints of local muscle soreness, and muscle spasm.61 Pain may also be referred to this region from trigger points in the upper trapezius muscle, splenius capitis, sternocleidomastoid (SCM) muscle, digastric muscle, cervicis muscles, posterior cervical muscles (semispinalis capitis, semispinalis cervicis, multifidus, and rotators), and suboccipital muscles (rectus capitis posterior major and minor and inferior and superior obliques) (refer to “Intervention” section).61
Complaints of headaches are also common in patients with craniovertebral joint dysfunction.32–34 Some of the more common types of headaches are described in Chapter 5. Cervicogenic headaches are described later in the chapter. In all patients presenting with an unexplained or an unusual headache, a full neurologic and general physical examination is required.62 This examination should focus on the patient’s mental status and speech, gait, balance and coordination, a cranial nerve and long tract examination (see Chapter 3).62
Patients who complain of a headache should be asked to describe the headache. The clinician must always be alert to the potential coincidental occurrence of secondary headache syndromes. These include a headache associated with trauma, vascular disease, nonvascular intracranial disorders, substance abuse or withdrawal, noncephalic infections, metabolic disorders, disorders of facial or cranial structures, and cranial neuralgia.63 Several conditions may mimic episodic or chronic tension-type headache (see Chapter 5), including muscle tension. Chronic tension-type headaches are often erroneously attributed to chronic sinusitis, but clinical and radiologic evidence must be obtained before making this diagnosis. A causal relationship between a tension-type headache and oromandibular dysfunction is controversial, but the two conditions often coexist.64 The most important structures that register pain within the skull are the blood vessels, particularly the proximal part of the cerebral arteries, as well as the large veins and venous sinuses.65 The chronic headache of intracranial hypotension is most often distinguished by an increase of pain on standing. Descriptions such as throbbing and pounding are suggestive of a vascular origin but can also inculpate a migraine, fever, neuralgia, or hypertension. Chronic, recurrent headaches can be associated with eyestrain, excessive eating or drinking, or smoking.
Intrinsic to the understanding of the relationship of a headache to the craniovertebral region are the intracranial pain pathways and their interconnections, especially the trigeminocervical pathway (see Chapter 3).
The location of the headache can provide the clinician with useful information as to the source.
Forehead pain may be caused by sinusitis or a muscle spasm of the occipital or suboccipital region.
Occipital pain can be caused by eyestrain, herniated cervical disk, hypertension, neuralgia, or an ear or eye disorder.
Parietal pain may be indicative of meningitis or a tumor.
Facial pain can be caused by sinusitis, trigeminal neuralgia, dental problems, or a tumor.
Dizziness (vertigo) and nystagmus are nonspecific neurologic signs that require a careful diagnostic workup (see Chapter 3). A report of vertigo, although potentially problematic, is not a contraindication to the continuation of the examination.
Systems Review
The craniovertebral region houses many vital structures. These include the spinal cord, the vertebral artery, and the brain stem. It is extremely important for the clinician to approach this area with caution and to rule out the presence of serious pathology. Craniovertebral and cranial dysfunction can be responsible for a number of signs and symptoms that may be benign or may indicate the presence of serious pathology (Table 23-2).
TABLE 23-2 | Examination Findings and their Possible Conditions |
Findings | Possible Condition |
Dizziness | Upper cervical impairment, vertebrobasilar ischemia, and craniovertebral ligament tear; also may be relatively benign |
Quadrilateral paresthesia | Cord compression and vertebrobasilar ischemia |
Bilateral upper limb paresthesia | Cord compression and vertebrobasilar ischemia |
Hyperreflexia | Cord compression and vertebrobasilar ischemia |
Babinski or clonus sign | Cord compression and vertebrobasilar ischemia |
Consistent swallow on transverse ligament stress tests | Instability, retropharyngeal hematoma, and rheumatoid arthritis |
Nontraumatic capsular pattern | Rheumatoid arthritis, ankylosing spondylitis, and neoplasm |
Arm pain lasting >6–9 mo | Neoplasm |
Persistent root pain <30 y | Neoplasm |
Radicular pain with coughing | Neoplasm |
Pain worsening after 1 mo | Neoplasm |
>1 level involved | Neoplasm |
Paralysis | Neoplasm or neurologic disease |
Trunk and limb paresthesia | Neoplasm |
Bilateral root signs and symptoms | Neoplasm |
Nontraumatic strong spasm | Neoplasm |
Nontraumatic strong pain in elderly patient | Neoplasm |
Signs worse than symptoms | Neoplasm |
Radial deviator weakness | Neoplasm |
Thumb flexor weakness | Neoplasm |
Hand intrinsic weakness or atrophy | Neoplasm, thoracic outlet syndrome, and carpal tunnel syndrome |
Horner’s syndrome | Superior sulcus tumor, breast cancer, cervical ganglion damage, and brain stem damage |
Empty end-feel | Neoplasm |
Severe post-traumatic capsular pattern | Fracture |
Severe post-traumatic spasm | Fracture |
Loss of ROM posttrauma | Fracture |
Posttraumatic painful weakness | Fracture |
ROM, range of motion. Data from Meadows J. Orthopaedic Differential Diagnosis in Physical Therapy. New York, NY: McGraw-Hill; 1999. |
Due to the proximity of the cranial structures, the clinician should develop the habit of quickly screening patients with neck and head pain for their ability to orient to time, place, and name; concentrate; reason and process information; make judgments; communicate effectively; and recall information. Obtaining this information needs to be done in a sensitive manner. Asking questions as to whether the patients know their own name and what day it is may be considered inappropriate in some patients. Most of the concerns about the patient’s mental status can be addressed through general conversation, or as part of the history. Perhaps surprisingly, the incidence of neurologic involvement in upper cervical spine injury is relatively low (18–26%).66 This may be because significant cord damage in the upper cervical spine is usually fatal secondary to respiratory arrest.7
When compression of the spinal cord is suspected, a thorough neurologic examination should be performed. If confirmed, the appropriate medical services should be contacted. The cranial nerves should be assessed, particularly if there are complaints about vision, or the patient appears to have problems with speech or swallowing. Patients with referred pain in the region of the trigeminal nerve commonly have an underlying disorder of the upper cervical spine, such as A-A instability caused by RA.61,67 As described in Chapter 3, the various cranial nerve tests can be performed in approximately 5 minutes with practice.
Tests and Measures
The examination is terminated if any serious signs and symptoms are produced.
Observation
The patient is observed in the sagittal, coronal, and transverse planes.
Sagittal Plane. Observing the status of the cervical curve and the relative position of the patient’s chin to the chest helps the clinician to assess postural alignment in the sagittal plane. A popular view among clinicians is that habitual adoption of extreme cervical posture may account for symptoms of pain and dysfunction arising from the head and neck area.68 The argument is that alterations in spinal alignment may lead to changes in muscle activity and loading on the surrounding soft-tissue and articular structures, which then predisposes patients to such complaints.69,70 This argument has been supported by several studies, which have found a statistically significant correlation between the tendency to hold the head forward, relative to the true vertical in the so-called forward head posture, and symptoms of pain.70,71 However, in other investigations, no such correlation has been confirmed.72,73
Tucking or elevation of the chin in the presence of a normal cervical curve may indicate craniovertebral dysfunction.74
The ears are observed for the presence of asymmetry in size, shape, or color. The top of the ear is normally in line with the eyebrow.
Coronal Plane. Alignment in the coronal plane is assessed by observing the orientation of the head relative to the trunk and shoulders, the leveling of the mastoid processes, and the symmetry of the cervical soft tissues. The face is observed for any asymmetry or indications of bruising, puffiness, prominence, swelling, perspiration, or abnormal skin color. Any asymmetry in the relative size of the pupils of the eyes and the distance between the upper and lower lids is noted. Pupillary size differences can occur in normal individuals but initially should arouse concern, because an abnormal unilateral change in size may be caused by an autonomic dysfunction or a central nervous system lesion.75 The superior lid should cover a portion of the iris but not the pupil itself unless ptosis or drooping of the eyelid is present75 (see Chapter 3).
Missing teeth should be accounted for as a loss of teeth may be a result of trauma, avulsion, or loosening.
Transverse Plane. Alignment in the transverse plane is assessed by observing the patient from behind and noting the orientation of the head. The orientation of the head is best observed by noting any asymmetry in the position of the mastoids, which can indicate whether the head is more rotated or tilted to one side, both of which may indicate a positional fault of the craniovertebral joints. Bruising around the mastoids or around the crown of the head (Battle’s sign), with a history of trauma, may indicate the presence of a cranial vault injury, such as a basilar fracture. A low hairline may indicate a condition such as Klippel–Feil syndrome,76 defined as a short neck with decreased cervical movement and low posterior hairline. Radiologically, patients with Klippel–Feil syndrome show a failure of cervical segmentation. The etiology is unclear, but the syndrome is believed to be caused by faulty segmentation of the mesodermal somites.77 The syndrome appears to be heterogeneous, with environmental factors possibly contributing.78 Autosomal dominant and recessive patterns of inheritance also have been reported.79
Active Range of Motion, Passive Overpressure, and Resistance
Short Neck Flexion. The clinician instructs the patient to place his or her chin on the surface of the throat. This motion simulates flexion at the craniovertebral joints. If this maneuver produces tingling in the feet or electric shock sensations down the neck (Lhermitte’s sign), it is highly indicative of serious pathology. Although Lhermitte’s sign is not a specific symptom, it is commonly encountered in patients with meningitis (see Chapter 5) and cervical spinal cord demyelination caused by multiple sclerosis.80 The sign also has been found in many other conditions that cause a traumatic or compressive cervical myelopathies, such as cervical spondylosis, cervical instability, and epidural or subdural tumors.81,82
If the patient reports a pulling sensation during short neck flexion, the cervicothoracic junction may be at fault. Active short neck flexion tests cranial nerve XI and the C1 and C2 myotomes, as well as the patient’s willingness to move. Placing the neck in short neck flexion places the short neck extensors (C1), which are innervated by the spinal accessory nerve, on a stretch. The clinician applies overpressure and tests the short neck extensors by asking the patient to resist (Fig. 23-5). Positive findings with this test are severe pain, nausea, muscle spasm, or cord signs, the latter of which may indicate a dens fracture or a tumor and cause the examination to be terminated.81 Thus, if a patient is able to perform short neck flexion, a cervical fracture or a transverse ligament compromise can be provisionally ruled out.
FIGURE 23-5 Short neck flexion with overpressure and resistance.
Short Neck Extension. The clinician instructs the patient to look upward by only lifting the chin. The patient extends the head on the neck, and the clinician attempts to lift the occiput in the direction of the ceiling (Fig. 23-6). An inability to perform, or pain during, this motion (in the presence of normal motion in the other planes) may indicate significant tearing of the anterior cervical structures. If this test produces tingling in the feet, it is highly suggestive of compression to the spinal cord. This compression may occur because of “buckling” or ossification of the ligamentum flavum, with resultant loss of its elasticity.83 A loss of balance or a drop attack with this maneuver would strongly suggest a compromise of the vertebrobasilar system. A drop attack is defined as a loss of balance without a loss of consciousness (see Chapter 24). The short neck flexors (C1), which are innervated by the spinal accessory nerve, can be tested in this position by applying overpressure as though lifting the patient’s chin toward the ceiling while the patient resists (see Fig. 23-6).
FIGURE 23-6 Short neck extension with overpressure and resistance.
Side Bending. The patient is asked to sidebend their head around the appropriate axis (through the patient’s nose). Side bending is included here for completeness. Much more a function of the lower cervical spine, side bending of the neck is nonetheless significantly decreased in cases of craniovertebral instability or articular fixation. It could be argued that, in the presence of serious ligamentous disruption due to a subluxation of the atlas under the occiput, this motion may provoke symptoms, but it is likely that such a significant incidence of instability would be detected earlier in the examination.84 The side-bending motions that do occur in the craniovertebral joints are essentially conjunct motions, so the results from this test are unlikely to provide much in the way of additional information.
Rotation. Neck and head rotation could be considered as the functional motion of the craniovertebral joints. Thus, if the patient’s symptoms and loss of motion are not reproduced with active rotation, it is doubtful that damage to tissues making up the craniovertebral joints is significant or even present. The patient is asked to perform active neck rotation. An inability to move any amount in either direction is potentially a very serious sign, as it could indicate the presence of a dens fracture or a C1–2 dislocation–fracture. Every measure must be taken to determine the cause of this inability to move. In cases of a suspected fracture or severe instability, the patient should be placed in a cervical collar and the physician immediately notified. In addition to the presence of a fracture, some of the other serious conditions that can be provoked by cervical rotation include vertebral artery compromise—cervical rotation is the most likely (single) motion to reproduce signs or symptoms of vertebral artery compromise (see Chapter 24).81,85–87
The findings from the cervical rotation tests may also afford the clinician some information with regard to a biomechanical lesion of the craniovertebral joints:
A loss of rotation associated with pain and a history of recent trauma. This could indicate the presence of an acute/subacute, posttraumatic arthritis of the craniovertebral joints. Since it may also indicate a soft-tissue injury, further testing would be required.
A loss of rotation associated with pain and a history of chronic trauma. This finding could indicate a chronic painless hypomobility with an adaptive but painful ipsilateral hypermobility involving the craniovertebral joints (e.g., pain with right rotation could occur if the right O-A joint cannot flex, and there is a hypermobility of the right A-A joint). It may also occur if the left O-A joint cannot extend. Again, further testing is needed to confirm this hypothesis.
A loss of rotation range of motion associated with no pain, but with a history of chronic trauma. This finding, which could indicate a chronic, posttraumatic arthritis, is likely to be an incidental finding since most patients seek help because of pain. However, depending on the extent of the loss of rotation, the patient may have become aware of a loss of function.
Full rotation range of motion associated with pain and a history of chronic trauma. This could indicate a chronic fibrotic (painless) hypomobility with an adaptive but painful contralateral hypermobility (e.g., pain with right rotation could occur if the left O-A joint cannot flex and the right O-A joint develops a compensatory hypermobility).
Palpation
Objective palpation of this area is guided by a sound anatomic knowledge. Palpation usually proceeds layer by layer. It should be noted that asymmetric joint geometry is common in this region.88 For spine palpation to be a valid indicator for manual techniques, the clinician applying it must first be able to differentiate between asymmetric motion caused by vertebral dysfunction and that caused by asymmetric joint anatomy.88 However, examination of the skin overlying the spine has been found to be very helpful, because certain skin changes in a particular location may point in the direction of a dysfunctional spinal area.89 The skin is assessed for its thickness, moisture, and ease of displacement in all directions. Abnormal autonomic skin reactions, such as erythematous changes, increased sweat production, and pain that can be induced with minimal palpatory pressure, may indicate a segmental dysfunction.90
Palpation may be started in the area indicated by the patient as painful. These painful sites must be correctly localized. The bony landmarks of this region that should be routinely palpated include the occiput, mastoid, atlas, and axis.
Occiput. The clinician locates the external occipital protuberance, which is the most prominent bony structure at the occiput in the midline. By following the external occipital protuberance laterally, the clinician can locate the superior nuchal line. The semispinalis capitis muscle is located about 1½ fingerbreadths below the superior nuchal line.90
Mastoid. The mastoid processes are located behind each ear. Once this structure is located, the clinician moves the fingers inferiorly toward the tip of the mastoid process. Starting from the medial tip of the mastoid process, the palpating finger is moved superiorly to the upper pole of the mastoid sulcus, an important area in the examination of the irritation zones of the occiput and C1.90
Atlas. By placing the palpating fingers between the mastoid process and the descending ramus of the mandible, the clinician can locate the transverse process of the atlas. The inferior oblique and the superior oblique both have attachments to this site.
Axis. The spinous process of C2 is the first prominent bony landmark that is accessible to palpation below the external occipital protuberance of the occiput. The spinous process of C2 serves as the origin of the inferior oblique muscle and the rectus capitis posterior major muscle.
Passive Physiologic Mobility Testing of the Occiput, Atlas, and Axis
O-A Joint. When mobility testing this joint, the first point to remember is that the joint is capable of both flexion and extension, and that side bending and rotation can also occur, albeit slight. The second point to keep in mind is that the arthrokinematics of this joint are the reverse of those occurring in the other zygapophyseal joints and that they occur in a different plane (horizontal). The joint mobility of the O-A joint can be assessed in sitting VIDEO or in supine.
With the patient supine, the head is extended around the axis for the O-A joint VIDEO. The head is then side bent left and right. As the side bending is performed, a gradual translational force is applied in the direction opposite to the side bending. The range of movement of the side bending is assessed from side to side as is the end-feel of the translation. This procedure is then repeated for flexion.
During extension of the O-A joint (Fig. 23-7), the occipital condyles glide anteriorly to the limit of their symmetric extension range. During left side bending and right translation in extension, the coupled right rotation is produced. This rotation causes the right occipital condyle to return toward a neutral position while the left condyle advances toward the extension barrier. If left side bending in extension is limited, then the limiting factor is on the left joint of the segment (ipsilateral to the side bending), which is preventing the advance of the condyle into its normal position. Thus, extension and right translation test the anterior glide of the left O-A joint, whereas extension and left translation stress the anterior glide of the right O-A joint (Table 23-3).
FIGURE 23-7 Passive mobility testing of O-A in extension.
TABLE 23-3 | Movement Restrictions of the Craniovertebral Joints and their Probable Causes |
Movement Restriction | Probable Causes |
Flexion and right side bending | Left flexion hypomobility Extensor muscle tightness Posterior capsular adhesions Left subluxation (into extension) |
Extension and right side bending | Right extension hypomobility Left flexor muscle tightness Anterior capsular adhesions Right subluxation (into flexion) |
Flexion and right side-bending motion greater than extension and left side bending | Left capsular pattern (arthritis and arthrosis) |
Flexion and right side bending equal to extension and left side bending | Left arthrofibrosis (very hard capsular end-feel) |
Right-side flexion in flexion and extension | Probably an anomaly |
During flexion of the O-A joint, the occipital condyles glide posteriorly (Fig. 23-8). The right rotation associated with left side bending causes the left condyle to move away from the flexion barrier toward the neutral position, while the right condyle is moved posteriorly further into the flexion barrier. Thus, flexion and translation to the right tests the posterior glide of the right O-A joint, whereas flexion and left rotation tests the posterior glide of the left O-A joint (see Table 23-3).
FIGURE 23-8 Passive mobility testing of O-A in flexion.
It is apparent that during these tests, the arthrokinematic and osteokinematic movements are tested simultaneously; thus, the end-feel must be used to determine the cause of the restriction. The following patterns of impairment are more or less commonly seen, and the causes of the impairments can be deduced. However, it must be remembered that deductions are only of value if the resultant intervention is successful.59
A patient who has a subluxation into flexion (loss of anterior glide) on the right O-A joint should demonstrate decreased extension, decreased right side bending and left rotation, and a jammed end-feel with translation to the left.
A patient with a periarticular restriction of the left O-A joint into flexion (loss of posterior glide) should demonstrate decreased flexion, decreased right side bending and left rotation, and a capsular end-feel with translation to the left.
A patient with a fibrous adhesion of the right O-A joint (loss of anterior and posterior guide) should demonstrate decreased extension and right side bending and decreased flexion and left side bending, with a hard capsular end-feel at both extremes.
With motion testing, a decreased flexion and right side bending with a pathomechanic end-feel indicates a left O-A joint being subluxed into extension.
With motion testing, a decreased extension, and right side-bending limitation indicates a capsular pattern of the right O-A joint. A decreased extension and left side-bending limitation, with a spasmodic end-feel (flexion with greater range), indicates traumatic arthritis of the left O-A joint.
A decreased right translation of the O-A in flexion may indicate a right posterior O-A joint dysfunction or an impaired or adaptively shortened right superior oblique muscle.
A-A Joint. There are a number of methods to assess the passive physiologic mobility of the A-A joint VIDEO. The most common method described in many texts involves the patient lying supine and the clinician passively applying full cervical flexion and then introducing cervical rotation. The problem with this technique is that it relies on the fact that the mid-to-lower cervical spine will be locked with the flexion. Because the neck is often prevented from further flexion when the chin meets the sternum, the clinician has no way of knowing whether full cervical flexion has occurred. Thus, some of the subsequent rotation may be attributed to a combination of cervical spine and A-A motion. This assumption may not be important with asymmetric lesions but can result in false-negative findings with symmetric lesions.
A better method of assessment involves the use of cervical side bending. With the patient positioned in supine, the clinician side bends the head and neck around the craniovertebral axis and then rotates the head in the direction opposite to the side bending (Fig. 23-9). The clinician assesses the amount of range available and then assesses the other side.
FIGURE 23-9 Passive mobility testing of A-A rotation in supine.
Combined Motion Testing
Flexion and extension at the O-A joints involve a posterior and anterior gliding of the occipital condyles, respectively. The same gliding (although reciprocal in opposing facets) is utilized in the rotation. At the A-A joint, flexion and extension primarily involve a “rolling” action of the condyles, with an insignificant amount of gliding. Therefore, craniovertebral flexion and extension will have a minimal effect on A-A rotation.93 Thus, if a symptom or range of motion is drastically altered by craniovertebral flexion or extension, an assumption could be made that the dysfunction is at the O-A joint.93 For example, if the right occipital condyle cannot glide posteriorly, the right joint will be unable to flex or to permit rotation to the right, as both these motions involve a posterior glide at the right O-A joint. In the combined motion tests, the right rotation restriction will be more evident when combined with craniovertebral flexion but will be less evident when combined with craniovertebral extension.
The findings from the combined motion tests can be used to determine which joint glide is to be assessed. For example, if it was determined in the combined motion testing that the right O-A joint is restricted or painful with flexion (implicating the posterior glide), the O-A joint is positioned and assessed in its extreme of flexion and right rotation (the two motions associated with a posterior glide of the right O-A joint).
Linear Segmental Stress Testing
The craniovertebral region demonstrates a high degree of mobility, but little stability, with the ligaments affording little protection during a high-velocity injury. Instability of this region can result from a number of causes:
Trauma (especially a hyperflexion injury to the neck).
RA, psoriatic arthritis, or ankylosing spondylitis. Nontraumatic hypermobility or frank instability of the O-A joint has been reported in association with RA.94
Corticosteroid use. Prolonged exposure to this class of drug can produce a softening of the dens and transverse ligament by deteriorating the Sharpey fibers, which attach the ligament to the bone. Steroid use also promotes osteoporosis, predisposing bones to fracture.
Recurrent upper respiratory tract infections or chronic sore throats in children. Grisel’s syndrome95 is a spontaneous A-A dislocation, affecting children between the ages of 6 and 12 years. The outstanding symptom is a spontaneously arising torticollis. The most likely etiology seems to be an inflammation of the retropharyngeal space caused by upper respiratory tract infections or by adenotonsillectomy and producing pharyngeal hyperemia and bone absorption.
Congenital malformation. Nontraumatic hypermobility or frank instability of the O-A joint has been reported in association with congenital bony malformations.96
Down’s syndrome. Nontraumatic hypermobility or frank instability of the O-A joint has been reported in children and adolescents with Down’s syndrome.97,98
Immature development. Patients younger than 12 years of age often have an immature or absent dens (see later).
Osteoporosis.
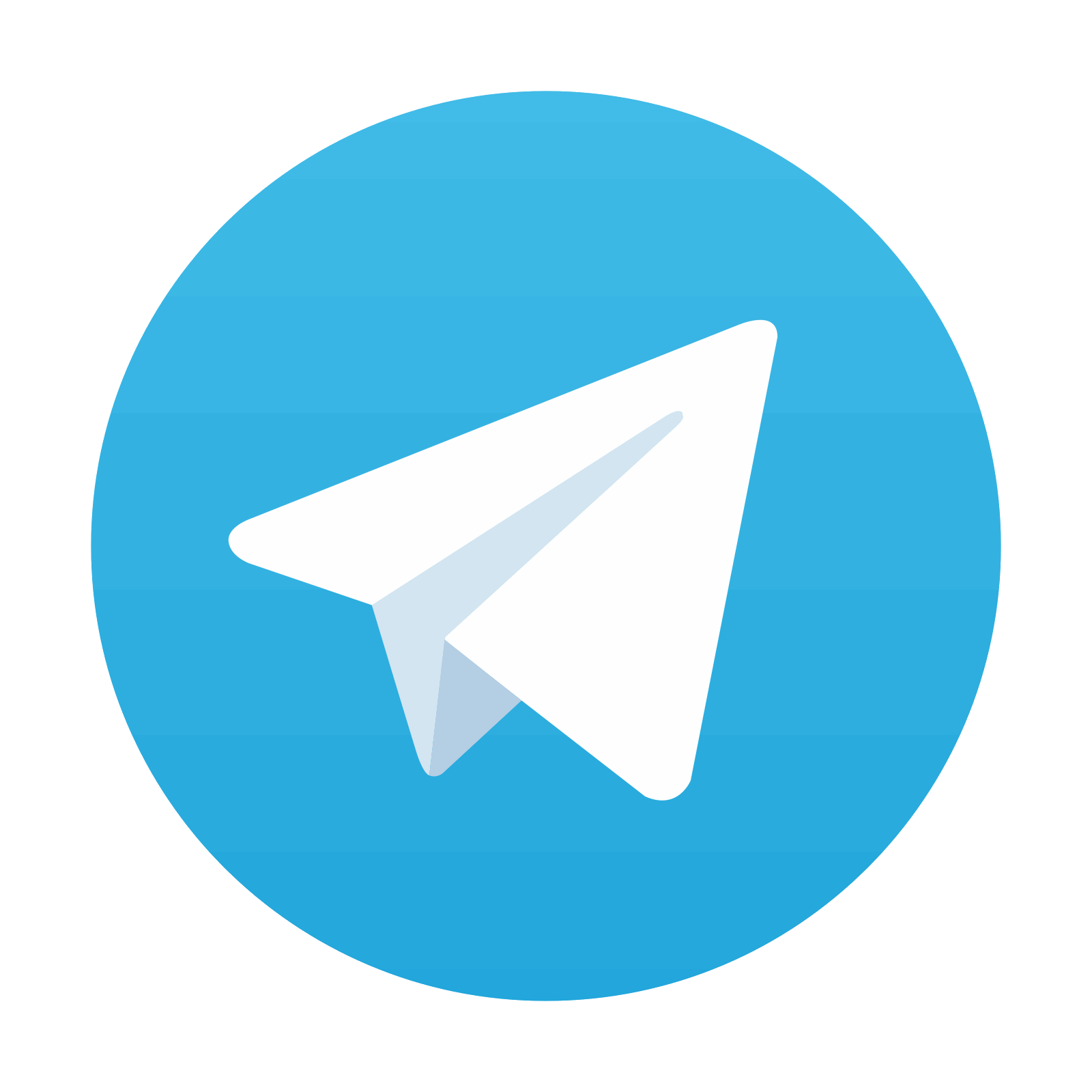
Stay updated, free articles. Join our Telegram channel

Full access? Get Clinical Tree
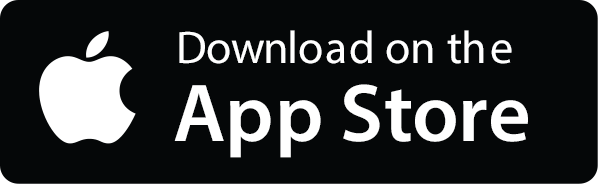
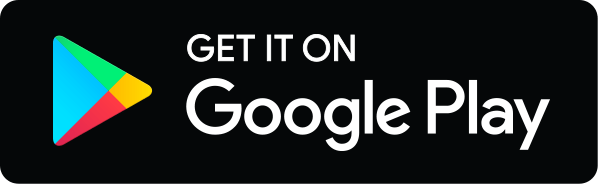
