Chapter 19 The Cerebral Dysfunction Syndrome
After reading this chapter you should be able to answer the following questions:
Question 1 | Is decreased circulation to the brain leading to loss of cell function reversible? |
Question 2 | Is decreased cerebral blood flow a rational explanation for previously observed effects of spinal manipulation? |
After spinal manipulation, patients often comment on the relief of some other health complaint that is not apparently related to the problem for which treatment was given. This chapter gives only an overview of the concepts mentioned. The development of reversible penumbra and irreversible infarction zones appear to depend on time as well as intensity of ischemia. Some regions of the brain are more vulnerable to ischemia than others: white matter is more resistant than gray matter, and there are differences in effects of degrees of ischemia between humans, nonhuman primates (even between monkeys and baboons), and other animals (for example, cats and rats).
• Nerve compression/irritation
• Spinal cord compression/irritation
• Somatovisceral (autonomic) reflexes
• Sympathetic nerve irritation
• Joint fixation (intrinsic and extrinsic)
• Intraarticular meniscoid locking
The cerebral dysfunction theory1,2 was developed to explain some of the reported and observed effects of manipulation. It is proposed that manipulation can result in increased cerebral blood flow (CBF), which restores normal cerebral functioning. Therefore manipulation can have hibernocerebrosomatic, hibernocerebrocognitive, and hibernocerebropsychologic effects.
Brain Hibernation
Ischemic penumbra was a term coined in 19813 to describe a condition in which CBF was between normal blood flow (allowing normal functioning) and blood flow low enough to result in irreversible tissue damage (causing cell death), much like a solar eclipse in which the penumbra is an area between normal light and darkness. In this penumbra state, neurons may be paralyzed (electrocerebral silence) yet viable (ionic pump function is maintained).
CBF normally approximates 0.5 ml/g/min in human beings with cerebral oxygen consumption of about 0.035 ml/g/min. A decrease in CBF is compensated for by an increase in oxygen extraction from the bloodstream (that is, the brain has a perfusion reserve). Such compensation (to allow normal neuronal functioning) can be made down to a level of approximately 0.23 ml/g/min.4,5 Neurophysiologic research4–18 demonstrates that if circulation to the brain is further decreased, cells may remain alive but their function ceases. If adequate circulation is restored, neuronal function is reactivated. Therefore areas of the brain can functionally hibernate (Figure 19-1).
The relationship between cerebral electrical activity and blood flow has been amply proven in experimental studies. Differences in actual figures are related to the experimental animals being studied. Neurologic deficits begin to appear in awake monkeys when regional CBF decreases below 0.23 ml/g/min6 and a gradual silencing of neurons sets in. Some cells continue to fire at levels at which others have become silent,5 with some cells still active at 0.06 ml/g/min.7 Local CBF of approximately 0.15 ml/g/min abolishes somatosensory potential recordings in the baboon cortex.8 Evoked potentials may be altered yet recover when flows of 0.16 ml/g/min are maintained for 14 to 60 minutes.8 Cerebral conduction time (a function of evoked response) is affected when CBF decreases below 0.15 ml/g/min.9 CBF below 0.18 ml/g/min in cats causes cessation of spontaneous neuronal spike activity in the cerebral cortex.10 In humans, flattening of the electroencephalogram (EEG) occurs immediately if hemispheric flow decreases below 0.16 to 0.17 ml/g/min, as evidenced by measurements of CBF and EEG during clamping of one carotid artery in endarterectomy.11,12 This flow level (approximately 0.23 ml/g/min) can be regarded as “critical” because electrical function in the cortex is disturbed below but is normal above this “critical” level (see Figure 19-1).
Cerebral blood flow of less than 0.10 ml/g/min results in deterioration of the energy state and ion pump failure, because in the absence of oxygen to produce ATP, anaerobic glycolysis is used. Glucose can be converted without oxygen to lactate and ATP, but the amount of energy yielded is small. This eventually leads to the accumulation of lactic acid with resultant intracellular and extracellular acidosis. When energy requirements are inadequate to maintain the normal distribution of K+(intracellular) and Na+(extracellular), membrane depolarization and ion pump failure occur with a massive efflux of K+ from the cells. This occurs at approximately 0.08 to 0.1 ml/g/min in lightly anesthetized baboons and is most likely applicable to the human brain. (Note: The threshold of membrane and metabolic failure is higher in smaller animals.)4,5 If such chaos persists for 5 to 10 minutes, irreversible cell damage and death occur (Figure 19-2).
Recovery of neurons suffering from a decreased blood supply is related to the duration of ischemia. Evoked potential in the baboon cortex could not recover after flow levels of 0.11 ml/g/min for 15 to 65 minutes13; most neurons recover from long ischemia (2 to 3 hours) only if flow levels remain above approximately 0.12 ml/g/min6(Figure 19-3).
Hibernation occurs between these two flow thresholds (0.12 to 0.23 ml/g/min, a difference of only 0.11 ml/g/min) and is characterized by electrical silence with normal or only slightly elevated extracellular potassium concentration. The residual perfusion (in the hibernation range) supplies sufficient oxygen to maintain a close to normal tissue concentration of ATP. Although changes in the concentrations of phosphocreatine (reduced), lactate (increased), ADP (increased), and adenosine monophosphate (AMP; increased) occur, resulting in some degree of energy failure, research suggests that this does not lead to neuronal damage.3,4,14–16 Although electrical activity may have been lost, recovery without irreversible damage (histologic signs of structural infarction) may occur (even if the increase in blood flow is very small) only as long as the residual blood flow has been on the safe side of the threshold for energy failure and ion pump failure. There is some evidence that clinical and electrical function may turn on and off in the ischemic area of the brain.17,18
Diaschisis
It is proposed that diaschisis possibly occurs after hypofunctioning of one part of the brain. Diaschisis was a term proposed by Von Monakow in 191419 to describe depression of brain function at structurally intact sites remote from the brain lesion. Human and animal studies have confirmed that localized damage to one area of the brain does cause reduction of excitatory impulses to another part of the brain, resulting in depression of the distant region20(Figure 19-4). Neurophysiologic research of diaschisis has been done in animals and stroke victims.21–32 These conditions represent the most severe and dramatic manifestations of diaschisis. Research has demonstrated that lesions in one hemisphere can affect functioning in the following areas:
• Contralateral cerebral hemisphere33–36
• Ipsilateral thalamus34,37–39
• Ipsilateral basal ganglia34,37
• Ipsilateral visual cortex; both primary and associated visual cortex34,37,40–43
Research has also indicated that reduction of regional CBF or metabolism in the ipsilateral frontoparietal cortex can occur in patients with lesions of the thalamus42 and with small infarctions of the internal capsule.34,42 Crossed cerebellar diaschisis has been reported48,49 after hypertensive hemorrhages. Although diaschisis is proposed as part of the cerebral dysfunction theory, further research is needed to determine whether decreased brain functioning in the hibernation range has this effect and to determine the necessary severity, size, and location of the hibernating region needed to produce alterations in functioning of distant regions of the brain.
Examples of Brain Hibernation
Clinical recovery of patients with embolic transient ischemic attacks is evidence of a state of ischemic hibernation, with complete reversibility. Similarly good recovery in minor stroke patients occurring over days or weeks suggests a cortical hibernation state of longer duration.5 Hibernation of cortical functions can occur for months or even years; when blood flow is increased, lost function returns. Cases reported in the literature tend to be the uncommon and the most severe and dramatic manifestations of this syndrome, but they are presented here to demonstrate that even in severe cases, return of adequate CBF can restore cerebral function to normal. This theory proposes that more subtle disabilities commonly occur in the brain but that they have not been considered and investigated.
1. Roski et al.50 reported on a 50-year-old patient with a documented 7-year loss of vision (right superior homonymous hemianopia and part of the right inferior quadrants). He had an expressive dysphasia (his speech was slow and deliberate); difficulty with reading, writing, and spelling; and weakness of his right hand and a right arm drift. A computed tomography (CT) scan demonstrated a lesion in the left occipital lobe. A dynamic flow study demonstrated decreased flow in the distribution of the left middle cerebral artery. Selective arteriography demonstrated occlusion of the left internal carotid and right vertebral arteries. After an anastomosis from the left superficial temporal artery to the angular branch of the left middle cerebral artery, the visual defect immediately completely resolved. In addition, his speech was noticeably improved and his right hand grasp was normal with no arm drift.
2. Brain dysfunction associated with decreased regional CBF has been reported in cases of depression,51–53 anxiety,51,54 personality disorders,55,56 and attention, concentration, and memory deficits.51 Examinations of CBF in depressed patients (against control groups matched for age, sex, and handedness) showed highly reduced values for gray matter blood flow in both hemispheres when compared with controls.51,53 The degree of depression was significantly inversely correlated with regional CBF (most manifest in the frontal regions). The authors believed that because depression is not associated with cerebral atrophy, functional hypoactivity of the neurons seems the most probable explanation for the cognitive dysfunctions (attention, concentration, and memory deficits) seen in depression. Another point relating depression to reduced CBF is that electroconvulsive therapy (ECT), which has antidepressant effects, causes cerebral blood vessel vasodilation.
3. Aphasias/dysphasias (associated with lesions of the posterior para-Sylvian association cortex) have been reversed after arterial anastomosis.50,57,58 Jacques and Garner57 described two patients with aphasia (one was global, the other expressive) and motor weakness (hemiparesis) that markedly regressed after superficial temporal to middle cerebral artery anastomosis (ST-MCAA).
4. Hand weakness has been reported to decrease after arterial anastomosis (increase in CBF).50,57,58 Lee et al.59 mention several improvements in neurologic status after an increase in CBF after ST-MCAA in patients at least three months after ischemic infarcts, so that all neurologic improvement would have occurred. They found that after ST-MCAA:
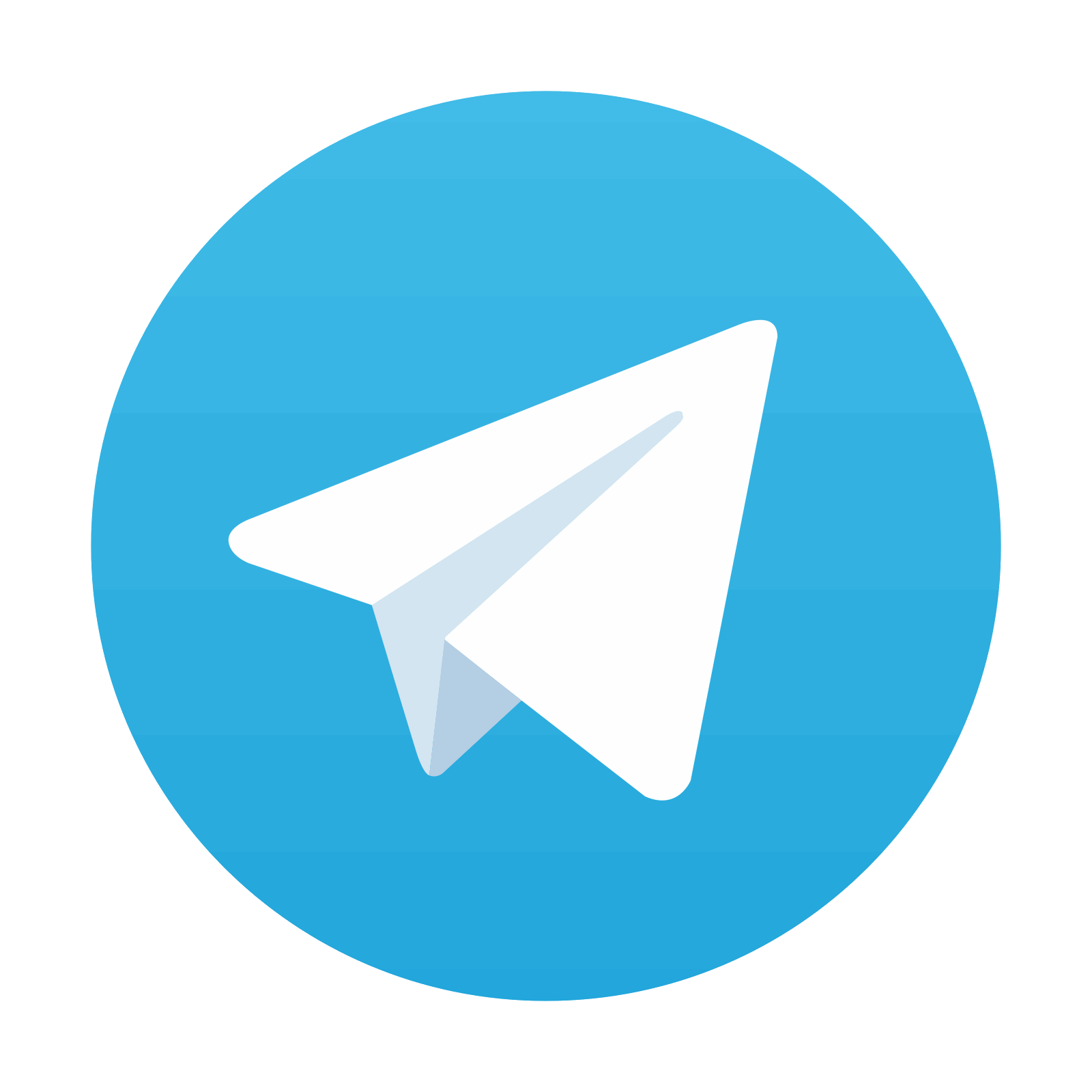
Stay updated, free articles. Join our Telegram channel

Full access? Get Clinical Tree
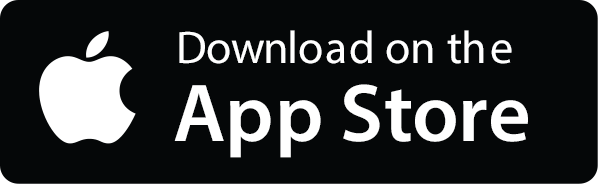
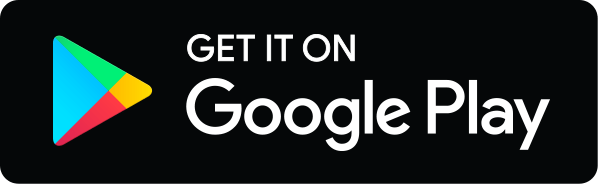