Tendon consists of tendon cells (fibroblasts) that lie in longitudinal rows and are elongated cells extending within the tendon structure and communicate via gap junctions within the three-dimensional space of the tendon. Also there is the extracellular matrix (ECM) that forms the scaffold for the tendon and which consists of a range of collagens (I, II, III, V, VI, IX and XI), proteoglycans and water (see Table 5.1 for relative proportions of components).
Table 5.1 Components of tendon tissue and relative constituent proportion (taken from Ker 2002). Reprinted, with permission, from Burgess KE, Pearson SJ, Breen L, Onambélé GN. (2009). Tendon structural and mechanical properties do not differ between genders in a healthy community-dwelling elderly population. J Orthop Res. 2009 Jun;27(6):820–5. (p. 823) © Elsevier
Wet mass (%) | Dry mass (%) | |
Water | 65b, 53–70e, 75g, 60j | |
Collagen: total | 50a, 76b, c, 99.7d, 60–85e, 65–80f | |
Type I | 67g, 65h | |
Type III | 10.0b, 3.5c, 1.8g, 0h | |
Type VI | 2.0d | |
Elastic fibers | 2e, 1–2f | |
Hyaluronan | 0.01f, 0.09g, 0.10h | |
Other proteins: total | 4.6j | |
Sulfated proteoglycans | ||
Decorin | 1.0j | |
Biglycan | ‘Half the decorin amount’d | |
Aggrecan | ‘Little’i | |
Other glycoprotein | ||
COMP | 1.0i | |
Anorganic | <0.2f | |
This list is indented only to give an indication of the main extracellular components. Other proteins which might be relevant, but for which I have not found quantitative data include tenascin-C, the small proteoglycans biglycan, fibromodulin, and lumican and the glycoproteins undulin and PRELP (Proline arginine-rich end leucine-rich repeat protein). Abbreviation: COMP = cartilage oligomeric matrix protein. | ||
aBank et al. (1999): human supraspinatus and biceps brachii. | ||
bBirch et al. (1999): horse forelimb superficial digital flexor. | ||
cBirch et al. (1999): horse forelimb deep digital flexor. | ||
dDerwin et al. (2001): mouse tail. | ||
eElliott (1965): general review. | ||
fKannus (2000): general review, giving ranges. | ||
gRiley et al. (1994): human supraspinatus. | ||
hRiley et al. (1994): human biceps. | ||
iSmith et al. (1997): horse. | ||
jVogel and Meyers (1999): bovine forelimb deep digital flexor. |
The largest proportion of collagen contained in tendon is type I, this lends strength to the tendon structure, whereas, the proteoglycans gives the tendon its viscoelastic properties.
It is now well known that tendon is a metabolically active tissue and adapts readily to influences such as mechanical loading and unloading (Heinemeier et al. 2009). This can be understood as a dynamic mechanism responding to feedback signalling via mechanotransduction pathways in an attempt to optimise the tissue characteristics to its function. Previous work has shown that tendon adapts to loading by modifying its mechanical properties and dimensions. Similarly it has been observed that tendon mechanical properties such as compliance are increased with unloading due to regulation of structural proteins, i.e. collagens. In line with this is the observation that tendon elastic properties are associated with the attached muscle (Muraoka et al. 2005) in that a muscle that is able to generate large force will generally be attached to a tendon that has adapted to cope with these forces.
The mechanisms of how the tendon responds to mechanical signals at a cellular level are still not well understood but are thought to involve the transduction of mechanical stressors via the ECM. The ECM forms the substrate for the anchoring of cells and enables the tendon to maintain its structural integrity. It has been suggested that forces generated end to end along the tendon are transferred along it by the matrix in shear connecting along the length of the discontinuous collagen fibres (Ker 1999).
Components called integrins, which are described as heterodimeric transmembrane proteins, form cell surface receptors that connect the tendon ECM to the cytoskeleton. Thus these are part of the adhesion complex, forming adhesion foci complexes at the cell membrane and are thought to be involved in the complex signalling processes resulting in modifications of structural proteins. These molecules when activated for example via mechanical stress, can initiate chemical signalling cascade processes, such as tyrosine phosphorylation, nuclear factor transcription factor (NF-kB), mitogen activated protein kinase (MAPK) pathway activation, either directly or as part of the process involving other growth factors (see Figure 5.2). Another ECM molecule that is receiving some attention is tenascin C; this is also seen to be up regulated with mechanical stimulus and is a ligand for integrin. The actual role of tenascin in tendon tissue is as yet not clear, but may involve actions related to tissue remodelling and general maintenance of the intercellular structures enabling gliding of bordering collagen bundles (Riley et al. 1996).
Figure 5.2 Schematic diagram showing possible signalling pathways for the up regulation of collagen synthesis via mechanosensitive pathways (from Chiquet 1999). Reprinted, with permission, from Chiquet, M. (1999). Regulation of extracellular matrix gene expression by mechanical stress. Matrix Biology. 18:417–426. © Elsevier (p. 422)
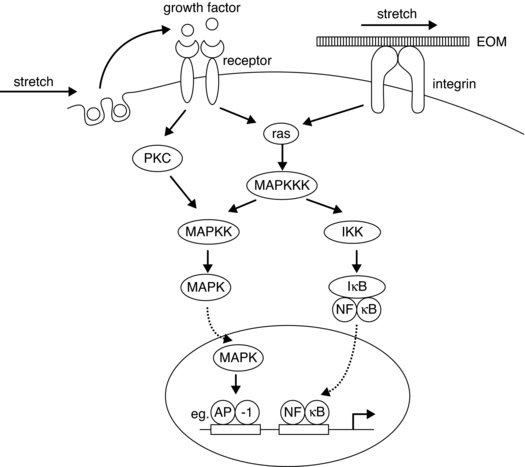
How integrins sense strain is not clear, in that although conformational changes are seen when forces are applied and ligands bind, other possible strain sensing mechanisms may involve a link between the calcium channel responses to stretch (reviewed in Chiquet 1999). Calcium channels are observed to open transiently for a relatively short period with stretch, this response is seen to be down regulated by interfering with the integrins (Chen and Grinnell 1995).
Functional aspects of tendon
Tendon is sited between the muscle and bone and acts to transmit forces generated by the muscle to the bone to enable movement. The forces experienced by tendons are usually tensile, although due to their arrangements with the bony anatomy, tendons may also experience compressive and shear forces. For example, tendon can glide over bony areas to allow moment arms to be utilised, potentially increasing torque transmitted via the tendon. The tensile properties of tendon allow for its capacity to resist rupture under normal loading conditions. Tendon is described as having viscoelastic qualities. These properties enable the tendon to act both as a damper to reduce potentially damaging high forces, but also as a storage medium for energy generated during movement.
It is usual to describe the tendon response to loading in terms of strain (percentage change of length from rest) and stress (force per unit area). A typical stress–strain curve for human tendon can be seen in Figure 5.3.
Figure 5.3 Stress–strain curve for elderly males and females (mean age 72 yrs) (Burgess et al. 2009b). Reproduced, with permission, from Burgess KE, Pearson SJ, Breen L, Onambélé GN. (2009). Tendon structural and mechanical properties do not differ between genders in a healthy community-dwelling elderly population. J Orthop Res. 2009 Jun;27(6):820–5. (p. 823) (C) 2009 John Wiley & Sons Ltd.
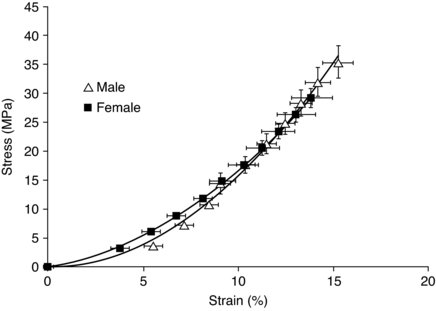
With respect to the strain, tendon has the characteristics of larger strain at low load, due in part to the crimp structure of the collagen fibrils, and a linear region where strain is proportional to stress, beyond this point the tendon starts to become plastic in that it takes on a new resting length when the load is removed. If stress is continued to be applied then ultimately tendon rupture will result (see Figure 5.4). Tendons with less crimp have been observed to fail before those with a more pronounced tendon crimp pattern (Wilmink et al. 1992). This may be reflective of the mechanical connections between the collagen fibrils and the ECM or some qualitative differences in the collagen structure.
Figure 5.4 Diagram showing elongation properties of tendon with progressive loading. Low load region (toe) shows characteristic greater strain than linear region, with subsequent plastic region leading to rupture.
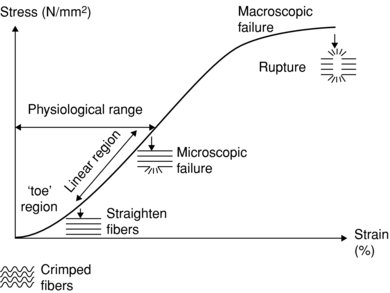
Regarding the ultimate tensile stress for tendon tissue, a conservative limit has been suggested of 100 MPa (Bennett et al. 1986). However Johnson et al. (1994) examined human patellar tendons and estimated that the ultimate tensile strength was 65 ± 15 MPa for young subjects.
Ker (2002) discusses ‘stress for life’, where a functional stress is determined from the ratios of the tendon to the muscle cross-sectional area (csa) and the product of the maximal isometric stress developed by the muscle (0.3 MPa, Wells 1965), with ranges from 8–100 MPa, but more normally approximating 13 MPa. It can be seen then that in certain circumstances a tendon may be subjected to stress which may be nearing its rupture limit, for example the patellar tendon has been reported to be subjected to approximately 12.2 kN during actions such as competitive weightlifting (Zernicke et al.1977), but under normal circumstances there is a relatively large margin of safety. This is perhaps best illustrated by considering the tendons involved in locomotion, such as the Achilles; it has been determined that this structure may experience forces in the order of 7 kN during running (Komi et al. 1992), this equates to approximately 8.5 times body mass for an individual of mass 80 kg. This may be even greater during actions such as long jump or triple jumping and has been reported to be in the order of 9 kN during jumping (Komi 1990).
However, with regard to tendinous damage, it is more likely generally that tendons suffer what is termed fatigue damage resulting from repeated loading cycles (see section on Chronic tendon injury).
Tendon has been shown to become damaged at strain values approximating 15% (Stäubli et al. 1999), although these values have been previously reported to be as high as 30% (Haut and Pawlison 1990). A functional design aspect of tendon to help limit damage is that of the ability of the tendon fascicles to slide with respect to each other whilst simultaneously transmitting forces along the tendon length. With respect to strain, it has previously been shown that there are qualitative differences between male ands female tendon, with females having greater compliance in comparison to males (Onambele et al. 2007). Similarly previous work has reported that in response to acute stretch females shown larger decreases in tendon stiffness in contrast to males, this may have implications where stretching is used as part of a warm up (Burgess et al. 2009a). This may reflect differences in the collagen content and/or composition between males and females. These gender differences do not seem to be retained in elderly tendon, however (Burgess et al. 2009b), possibly reflecting the changing hormonal influences on tendon being less disparate between the genders with age and more similarities in lifestyle.
At the levels of loading seen routinely, tendon may suffer micro damage at levels of strain seen normally, that is below 10%, leading to accumulated deterioration in tendon (see section on Chronic tendon injury). Normal ranges of strain under conditions of maximal isometric contraction for human tendon structures are within the ranges of 6–14%. The range of normalised elasticity (Young’s modulus) for tendon is approximately 0.8–2 GPa. This property represents the normalised (corrected for differences in tendon length and cross section) stiffness of the tissue and is the gradient of the stress-strain curve.
As tendons are also elastic they tend to stretch when muscle contraction takes place. If, for example, the external load is very heavy, then as the muscle contracts the tendon may stretch initially, the action of the tendon here could be thought of as similar to a spring being stretched and energy stored. This may be functionally important, an example of this could be where an athlete is sprinting or jumping and the tendon is being subjected to stretch-shortening cycles. This enables the movement to be carried out with higher efficiency than if the tendon did not stretch and then shorten, in that the tendon is initially storing energy during the stretch, to be released during the shortening or recoil period. It has been reported that tendon has the ability to return ∼93% of its stored energy during the recoil phase, the rest being lost as heat energy (Alexander 2002). This particular property of tendon is perhaps useful where for example cyclical movements are being carried out with changes in kinetic and potential energy or to increase the power component as in jumping. The tendon can act to help smooth out these changes and store some of the energy as elastic strain energy to be returned to the system when required, reducing the overall work required for the muscles (i.e. running). However, where high rates of force development are required, tendon compliance could be seen as potentially detrimental to optimal performance.
The mechanical properties of the tendon, in particular the stiffness, can also be seen to potentially affect the characteristics of muscle contraction. A muscle’s contractile ability in part is described by its length tension curve, by which the number of elements that generate muscle force (cross bridges) are increased or decreased dependant on the muscle length (see Figure 5.5). Here it can be seen that if the tendon is compliant and stretches under load, the muscle will shorten, and this could affect its ability to generate force. As can be seen from Figure 5.5, if the muscle is starting from a position near maximal force and the tendon stretches, the muscle will shorten moving down the force generating curve.
The position where the tendon attaches to bone can have functional implications. A muscle can only extend or shorten over a given range; the points at which tendon attaches to the bone can thus affect the movement of the limb to which the muscle–tendon complex is attached. This can be seen to be useful from a functional standpoint where for example force is preferred over range of movement and visa versa (see Figure 5.6 for example). Here, where the tendon attaches closer to the joint centre of rotation as in Figure 5.6A, the limb being affected can move further for a given muscle shortening than where the tendon attaches further from the rotation centre (Figure 5.6B). This is at a cost of reduced torque generating ability.
Figure 5.6 Differences in limb range of movement with tendon attachment. A: Tendon attaches closer to joint centre of rotation. B: Tendon attaches further from centre of rotation than A.
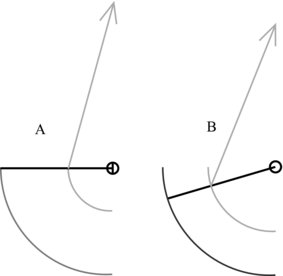
Tendon injury and its management
Tendon injury can be debilitating in so far as a damaged tendon will likely reduce the capacity for transfer of forces via the muscle to the bone. This has obvious implications for a range of activities ranging from normal everyday movement to elite level athletic performances where perhaps high levels of force and rates of force development are prerequisite.
Injury to the tendon can be characterised as acute resulting in either a catastrophic rupture or a less major tear of the tendon tissue. Alternatively the tendon may suffer chronic pathological changes which may be inflammatory or not even detectable. These factors can affect the tendon at numerous sites along its length. There appears to be no set consensus on description for the degenerative or pathological changes to tendon (see Table 5.2 for descriptors, taken from Wang et al. 2006).
Table 5.2 Description for degeneration or pathological changes in tendon
Term | Description | Reference |
Tendinitis or tendonitis | Inflammation of the tendon | Clancy et al. (1976); Almekinders and Temple (1998); Curwin and Stanish (1984); Schepsis and Leach (1987) |
Tendinosis | Asymptomatic tendon degeneration | Puddu et al. (1976); Järvinen et al. (1997) |
Tendinopathy | Generic description for tendon disorders | Khan et al. (2002); Maffulli et al. (1998) |
Paratenonitis | Inflammation of the paratenon | Maffulli et al. (1998); Jozsa and Kannus (1997) |
Peritendinitis | Inflammation of the peritendon | Maffulli et al. (1998); Jozsa and Kannus (1997) |
Spontaneous tendon rupture | Tendon rupture without any predisposing symptoms | Kannus and Jozsa (1991) |
Partial rupture | Incomplete tendon tear | Karlsson et al. (1992); Karlsson et al. (1991) |
Enthesopathy | Tendon-bone junction disorders | Maffulli et al. (1998); Kvist (1991) |
With regard to acute tendon injuries, although a number of these could be identified due to extrinsic factors, such as direct contact with external body, or accidental unaccustomed loading such as extreme shear, the majority of acute tendon injuries show prior predisposing factors. These potentially contributory factors can be thought of as chronic inflammatory or accumulative degenerative conditions and may be undetected prior to the acute trauma.
Acute tendon injury
Immediate tendon injury
This can be perhaps best described as either complete rupture of the tendinous structure or partial rupture. Complete rupture will be self-evident and accompanied by loss of function, pain and swelling usually following the incident. On inspection it may be obvious that rupture has occurred in that a gap may be seen where the tendon would normally be. More specific diagnosis of rupture can be carried out using imaging techniques, such as ultrasound (see Figure 5.7) or magnetic resonance imaging. Tendon injuries of this nature tend to be unilateral and of those reported for the achilles, 75% were related specifically to sport (Jozsa et al. 1989 cited in Leppilahti and Orava 1998). The tendons mostly seen to be suffering rupture are those bearing higher functional loads, such as the achilles and patellar tendons. Site of tendon rupture has also been previously suggested to be associated with hypovascular regions of the tendon. For example, achilles tendon ruptures typically occur 2–6 cm proximal to the insertion site at the calcaneus. This area along with the insertion site is also known to be poorly supplied with blood (Carr and Norris 1989; Niculescu and Matusz 1988).
Figure 5.7 Saggital view of achilles tendon rupture indicated by arrows (from imaging consultant.com).
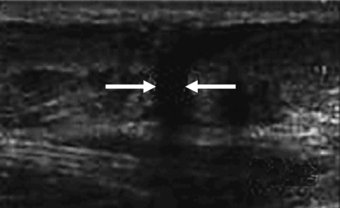
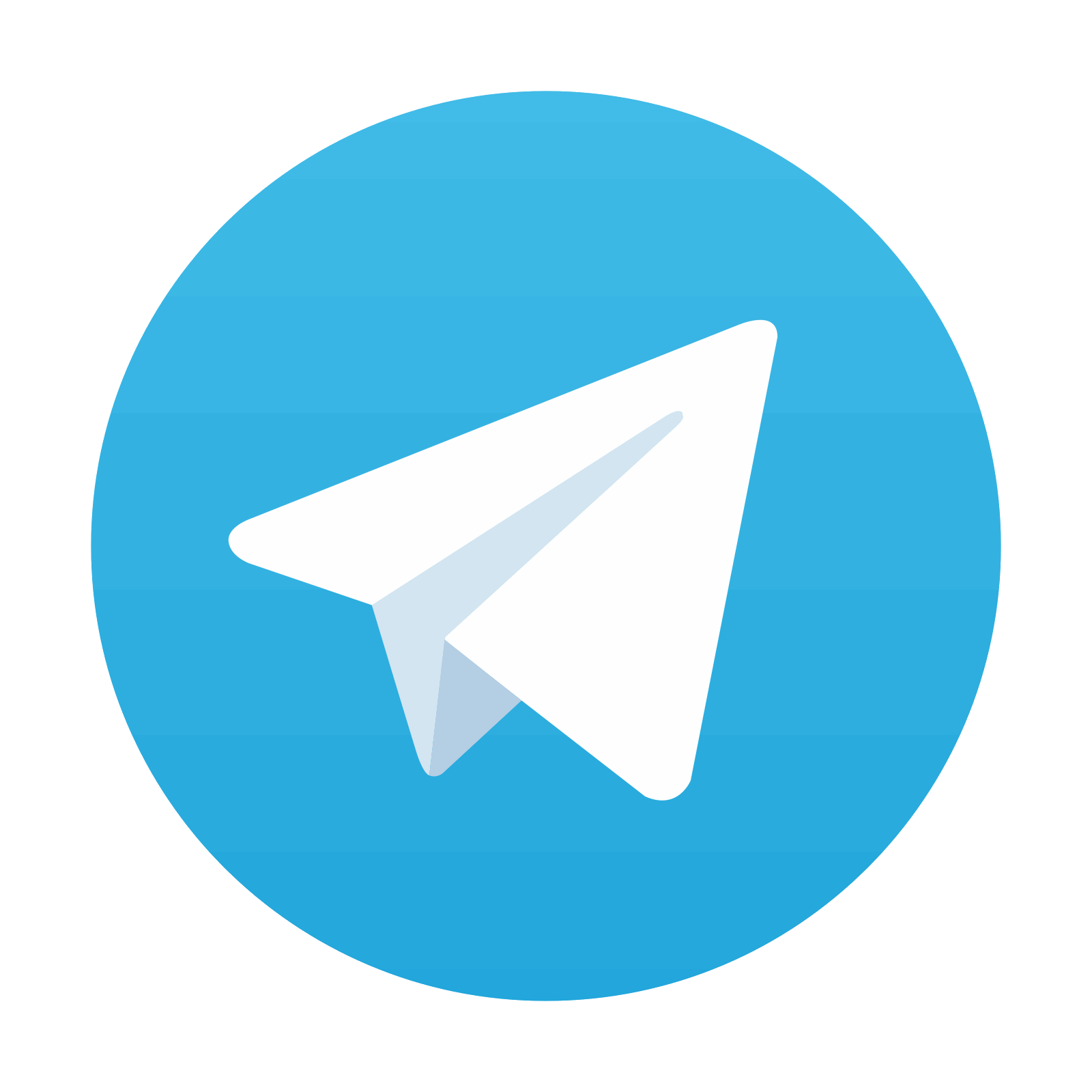
Stay updated, free articles. Join our Telegram channel

Full access? Get Clinical Tree
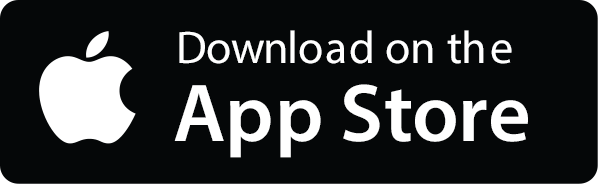
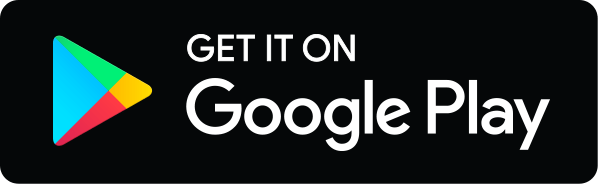