Training to maximize RFD
Rate of force development is best enhanced by training explosive movements where the intention is to accelerate the resistance (either a bar, medicine ball, or the body) as quickly as possible. Slow heavy exercises increase strength (and therefore the maximum force athletes can produce), but do not increase the force development within the first 200ms of contraction, required by sport in conditioned athletes (Hakkinen and Komi 1985; Zatsiorsky 1995), although very heavy resistance exercise can increase RFD within the first 200ms, in individuals who have not previously participated in structured resistance training, via enhanced neural drive (Aagaard et al. 2002). It is worth noting, however, that all of these types of training play their role within a well developed periodised training programme.
Figure 13.2 represents the RFD characteristics of untrained, heavy-resistance trained, and explosive-ballistic trained athletes. Note that the heavy-resistance trained athletes have a higher maximum strength, but take up to 500ms to develop this strength. Explosive-ballistic trained athletes, however, can generate greater force within the 200ms timeframes typically encountered in sport. It is therefore essential that a complete rehabilitation programme prepares the athlete for such demands, rather than concentrating on isometric strength, or low velocity concentric strength as with most ‘traditional’ rehabilitation programmes. In a periodised model the heavy training should precede the explosive training.
Figure 13.2 Isometric rate of force development characteristics of untrained, heavy resistance trained, and explosive-ballistic trained athletes. Adapted from Hakkinen and Komi, (1985).
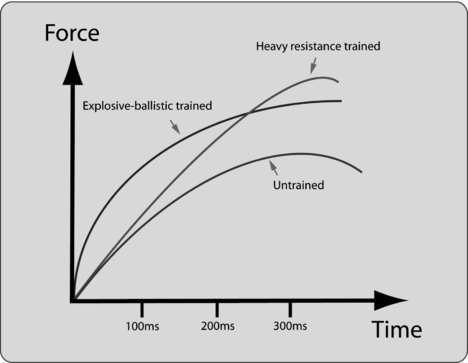
Although explosive-ballistic training is effective at improving rate of force development and strength in the initial stages of muscle action (up to 200ms), it is still possible to focus training even more accurately. For example, during a sprint, ground contact times, and therefore the time over which an athlete can generate useful force, change throughout the race. At the beginning of the race, when the athlete drives out of the blocks and accelerates for the first few strides, the ground contact time can be greater than 340 and 200ms respectively (Mero 1988), whereas when the athlete reaches peak running velocity, the contact time is nearer to 70–125ms.
This knowledge is crucial to help athletes focus training more specifically on their strengths and weaknesses. For example, if an athlete accelerates well for the first few metres but cannot compete at the faster speeds then they will benefit from training that focuses on shorter ground contact times (short response or reactivity training) such as plyometrics. If an athlete has a high peak velocity but is slow when accelerating then they should focus on strength and power development over a longer ground contact time (long response training) such as weighted jump squats, Olympic lifts, or single leg hopping and bounding. (Single leg hopping has a ground contact time of approximately 230ms according to Aura and Viitasalo (1989).) This has a particular relevance for team sports where the ability to accelerate over the first few metres may be far more important to success than peak running velocity. In soccer, sprints generally last between 12 and 22m (Bangsbo et al. 1991; Drust et al. 1998).
Likewise, long-jumpers and high-jumpers exhibit different ground contact times during the take off (125ms and 200ms respectively). Training for each should therefore focus on different aspects of strength development with the long-jumper placing a greater emphasis on training exercises with a shorter contact time (short response) and the high jumper focusing on exercises with a longer contact time (long response), especially prior to competition (see Figure 13.3.).
Figure 13.3 Representation of the change in ground contact time during a 100m race. Training should be tailored to the relative strengths and weaknesses of each athlete.
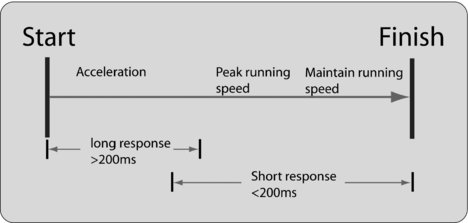
Short response training – reactivity training
Short response (reactivity) training is specifically designed to help athletes accept and produce forces over very short periods (typically 75–200ms). Short response training is therefore fundamental to maximising sprinting speed and jumping ability as these activities require athletes to accept and produce ground reaction forces quickly. The best type of training for reactivity is plyometrics, focusing on those with a short ground contact time.
Plyometric training involves very quick, light, yet powerful activities like quick feet, skips and low amplitude jumps. Emphasis should be placed on speed, maximising the rate of stretch, minimising ground contact time, and good technique, rather than height jumped, the height of the box jumped from and the length of the bound. Although the height of the subsequent jump does increase with increasing drop heights (due to an increase in the rate of stretch at landing) this only occurs up to a threshold height (Bosco and Komi 1979). Above this height, the rate of stretch is too high and the golgi tendon organ (GTO) reflex inhibits muscle contraction (Schmidtbleicher et al. 1988), lessening subsequent jump height. Training aimed at developing the reactive elements of muscle contraction should not use drop heights greater than this threshold. Although Kreighbaum and Katherine (1996) suggest a platform height of no more than 20cm to minimise the risk of injury, for many athletes the threshold occurs at heights much greater than this, although usually no higher than 60cm. It is also worth noting that plyometrics can be effectively performed in water (Robinson et al. 2004; Stemm and Jacobson 2007), therefore reducing the impact forces, due to the buoyancy of the water, and allowing plyometric training to be introduced to athletes at an earlier stage in their development/rehabilitation.
It is also interesting to note that plyometric training has been shown to have a beneficial effect on tendon and joint stiffness (Burgess et al. 2007; Kubo et al. 2007a), which may reduce the risk of injuries.
The type and intensity of exercises incorporated into the training session will determine the number of repetitions performed. For example, mini-hurdle jumps, where the emphasis is on minimising ground contact after a relatively low drop height, can be performed in multiple sets (5) of high quantity (25), high quality (rapid; short contact time) jumps. In contrast, full-hurdle jumps, where the athlete must clear a height of one metre with each jump, elicit a greater impact velocity and, therefore, higher impact forces on landing. To maintain the quality and intensity of movement, the number of repetitions should be kept low (four–eight). Higher intensity exercises (single leg hops, or single leg depth jumps in well-conditioned athletes) may be limited to one to four quality repetitions per set. See Table 13.1 for a summary.
Table 13.1 Short response training
It is essential to remember that plyometric training places a high neurological demand on the athlete and is therefore most effective when athletes are well rested.
Movements such as depth jumps, hopping, and bounding typically have longer ground contact times and come under the heading of long response training.
Long response training
Long-response training refers to the training of powerful explosive movements with a contact or application time of more than 200ms. By comparison with short response training long response training is characterised by greater amplitude of movement about the hip, knee, and ankle joints and also by the use of a greater external resistance. Whereas short-response training is dominated by quickness of movement, long response training is dominated more by strength.
Long response movements include activities ranging in speed from faster activities of around 200ms (bounding, tire pulling, single leg hopping and depth jumping) to longer activities of up to 500ms (un-weighted squat jumps, one legged jump-squats and weighted jump squats). This type of training can enhance force production, acceleration from a standing start and height jumped. Bosco (1982) found that the inclusion of depth jumps in training resulted in an increased jump height. Research has also demonstrated that including drop jumps (five jumps from a 60cm box) within a dynamic warm up result in an increase in subsequent power based activities (Hilfiker et al. 2007). It is essential that the training programme meets the physiological, biomechanical and metabolic requirements of the sport; these will be introduced later on in the chapter.
Isometric training
In contrast to the beneficial effects of explosive training compared with heavy strength training, research has demonstrated that isometric training can also result in increases in RFD, power and vertical jump height, which is attributed to increases in tendon stiffness (Kubo et al. 2001; Bojsen-Moller et al. 2005; Kubo et al. 2007b; Burgess et al. 2007). It is worth noting, however, that this type of isometric training must be performed with the intention of explosive movement, and should not be the sole method of training. It is generally incorporated into the ‘normal’ training session as a few additional sets.
A key concept to understand is the force velocity relationship of skeletal muscles, applied to training methodologies and the requirements of sporting activities (see Figures 13.4 and 13.15). This includes both concentric and eccentric muscle actions that are responsible for acceleration and deceleration respectively, which therefore corresponds to improvements in agility. It is essential that these concepts are applied when identifying the mechanics of the sport/activity and integrated into the exercise selection process.
Intensity
Overload
This is usually referred to as ‘progressive overload’. More often, however, a form of ‘fluctuating overload’ (Figure 13.5) is applied as part of a periodised training programme (Siff 2004). Failure to periodise in this manner by attempting an unlimited linear progression (Figure 13.6) will either result in stagnation and a plateau in training (Figure 13.7), or increase risk of injury due to lack of appropriate adaptation. See Chapter 9 for more detail on periodisation.
Overload and super-compensation
For adaptations to occur, the training stimulus must be at a level beyond that normally encountered. Training at a level or intensity that is beyond that normally encountered is called overload, and it is this overload that stimulates the adaptations that allow the athlete to tolerate an increased level of training stress.
Training causes physiological, biochemical and mechanical stresses. Immediately post training, these result in tissue damage and metabolic, neural and psychological fatigue, limiting performance and making the athlete (temporarily) less able to perform. (For a period post-training the athlete is actually less fit). Once training has finished, however, recovery begins. This process involves restoration of physiological and biochemical balance (homeostasis), repair of tissue and replenishment of muscle and liver fuel stores. Depending on the type, intensity and duration of the training – and the quality of rest and nutrition – full recovery can take from a few hours to several days (or weeks, depending on the intensity and duration of training).
Once pre-exercise levels have been restored, recovery is still not complete. The body continues to adapt, rebuilding and remodelling itself until a higher state of readiness is reached. For a short period of time, an athlete’s fitness is enhanced. This is known as super-compensation (adaptation). The time period during which super-compensation occurs provides a window of opportunity during which the athlete should train again. If the athlete trains during this period of super-compensation, the cycle is repeated and fitness continues to improve. If, however, the athlete does nothing during this key period then the body will re-adapt to the current, lower activity level. Any enhanced state-of-readiness or fitness that occurred as a result of super-compensation will be lost. Figure 13.8 illustrates the principle of super-compensation from one training session.
Timing
The timing of the next training session is vital and varies according to the recovery abilities of the athlete and the demands imposed by the previous session. For example, a well-conditioned athlete can recover from a long training run within 24 hours (excluding the skeletal system), whereas the same athlete may take 10 days to recover from a heavy resistance training session involving rapid eccentric activities and supra-maximal loads.
Once an athlete has adapted to a specific training load or stimulus (for example running five miles, three times per week at a constant pace), then further gains can only be made if the training load is increased. This is known as the principle of progressive overload. The runner (above) will not improve beyond the fitness level needed to run five miles, three times per week, at that constant pace. Once that level of fitness is achieved it will remain static. In order to improve their running ability further the stimulus must be increased. The runner could run faster, farther, more often, use interval training or run on a more challenging route.
The progressive increases in fitness due to repeated training sessions are illustrated in Figure 13.9 Note that each successive training session coincides with the point during recovery when gains from the previous session have been maximised – the ‘window of opportunity’.
Although the stimulus to improve comes from the training session itself, all improvements occur when the athlete rests. If the rest period is too short, and the athlete trains again before full recovery has occurred, then fitness will cease to improve and, if this cycle continues, may even decline (Figure 13.10). During such situations, athletes are more susceptible to overtraining syndrome, injury and illness. Adequate recovery is therefore essential to a successful training programme.
It should be noted that there are times when athletes may perform successive hard training sessions in a block, without allowing full recovery. For a few days, the athletes accumulate fatigue. This shocks the system and gives an opportunity – with sufficient recovery later – to stimulate further adaptations (Figure 13.11), and can also be used when ensuring that an athlete peaks for a specific competition (Pistilli et al. 2008). This is an advanced training method known as shock training (planned Overreaching) and should only be used by athletes who already have a solid training base. Careful observation and monitoring is required as shock training itself represents the initial stages of overtraining, a condition that is associated with both increased injury and illness risk and well as a reduction in performance. There is often a fine line between providing the optimum stimulus for adaptation and inducing a state of over-training and susceptibility to injury and illness.
Figure 13.11 Shock training / overreaching – repeated training sessions with inadequate recovery, followed by an extended rest period can lead to a jump in fitness levels.
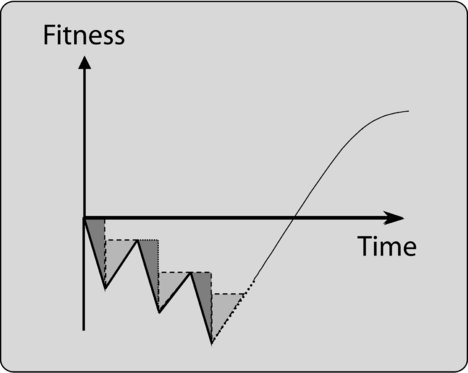
Adaptation potential
An athlete’s adaptation potential (or capacity for improvement) is a function of both their genetic makeup and their current level of fitness. Genetic makeup, an athlete’s ultimate potential for success, is fixed and therefore not responsive to training (Brzycki 1989). The major factor determining an athlete’s adaptation potential then is their current state of fitness relative to their genetic ceiling.
The primary training aim of all athletes should be to fulfil their genetic potential. This is never easy. As athletes get fitter and more skilled they find it increasingly more difficult to make further improvements – they get diminishing returns for their efforts. As they approach their genetic ceiling, further improvements become almost impossible (Figure 13.12).
Figure 13.12 Adaptation potential (adapted from Balyi 2001) – Novice athletes have a greater adaptation potential (capacity for improvement) than elite athletes.
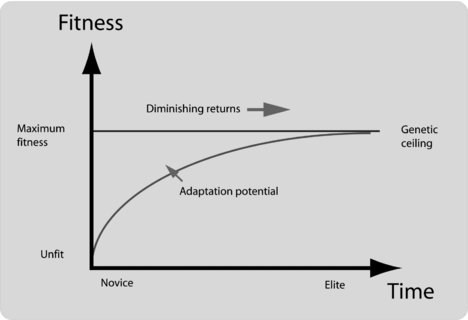
Conversely, athletes that are unfit have the greatest capacity to improve. For these athletes, increases in fitness occur rapidly in response to training. These rapid improvements, however, can occur independent of training quality. Even poorly designed training programmes will elicit improvements in the short term. In the medium term, however, such training will plateau, bad habits can form, and the athlete may not fulfil their true potential.
It is crucial; therefore, that athletes and coaches adopt the same, thorough and careful approach to training programme design with all athletes, whatever their age and training status. A well-designed programme in the early stages ultimately lays the foundation for future success.
Training parameters
The training parameters that contribute towards the overall training effect include: frequency, intensity, and duration of training sessions; the quality and quantity of rest between and during sessions; and specificity. Each of these parameters can be combined and manipulated within a training programme to elicit the desired training effect.(See Tables 13.2 and 13.3 for specific recommendations.)
Table 13.2 Definitions of training parameters
Training parameters | Definition | Example |
Frequency | How often | Training 3x per week; daily |
Intensity | How hard | Running at a speed of 10mph; lifting a resistance of 90% of maximum capacity |
Duration | How long (in terms of time, distance, or volume) | Cycling for 20 minutes; running for 10 miles; performing a number of sets/repetitions |
Rest | What an athlete does to recuperate. (Both quality and quantity are important.) | Two days between each session; 4 minutes between each set. Type: sleep; massage; active rest. Diet: high carbohydrate |
Specificity | Type of training | Focus on endurance; speed; sport specific movement patterns |
Table 13.3 General recommendations for the development of different fitness attributes
Rate of adaptation
Different fitness attributes respond to training at different rates. For example, improvements in flexibility can occur within days, whereas improvements in speed may take months. In addition, strength improvements can occur within weeks; sport specific endurance may take weeks and months; and overall work capacity will only improve gradually over a period of months and years. Athletes and coaches must also be aware that different body systems adapt at different rates following training (see Table 13.4). For example, with strength training, the rate of adaptation of tendons and bones is slow compared to the rate of adaptation of muscle. The consequences of this are that muscles get stronger quicker and pull on tendons that are yet to adapt to the stresses of training. This leads to the potential for injury.
Table 13.4 Adapatation rates following training
Fitness attribute | Rate of adaptation |
Flexibility | Days |
Strength | Weeks |
Sport specific endurance | Weeks to months |
Speed | Several months |
Work capacity | Months to years |
Detraining
All improvements in fitness are reversible. What an athlete can gain with training, they can lose with detraining. When athletes stop or reduce their training, their body systems adjust accordingly. This presents as a loss of strength and power (Narici et al. 1989; Hakkinen et al. 2000; Weir et al. 1997), a decrease in maximal aerobic capacity (Mujika and Padilla 2001), and a loss of coordination. Although detraining of strength and power is relatively slow (Colliander and Tesch 1992; Hortobagyi et al. 1993), particularly with the inclusion of some eccentric exercise (Housh et al. 1996), the detraining of endurance is more rapid with significant effects noticed in days and weeks (Coyle et al. 1986).
Overall, the de-training effects of a short-term break (1–2 weeks) are relatively small, especially if physical activity levels remain high, however longer or more frequent periods of inactivity have a greater cumulative impact on overall fitness. Thus, when athletes resume training after a layoff or injury, they cannot start at the level (intensity, duration or complexity) at which they left off. Care must therefore be taken when designing training sessions that occur after an injury or other long lay-off. In such cases, a gradual and progressive re-introduction to full training will carry fewer risks of re-injury and produce more sustainable gains than other more intense methods. Many athletes and coaches are keen to make up for lost time and consequently train too-hard, too-soon, risking both injury and possible over-training.
It is far better, therefore, to minimise fitness losses in the first place. To minimise fitness losses, injured athletes must examine strategies, within their treatment and rehabilitation, which enable them to continue to exercise. One such strategy may involve the use of alternative low-risk activities that do not stress the injury. For example, rowing, stationary cycling and deep water running are all excellent methods of maintaining general cardiovascular fitness when injured; strength training can be targeted towards key movements in non-injured areas; whilst proprioception training of the non-injured limb can provide useful crossover benefits to the injured limb.
Specificity
The most important element of functional conditioning is the principle of specificity. The principle of specificity dictates that training should match the demands of the sport. Given that sport consists of many components – with successful athletes needing to start, stop, twist, turn, run, jump, land, shuffle, push, pull, hit, bend, throw, catch, hop, accelerate, decelerate, slide, block, and barge, all within the fluid, dynamic, and unpredictable environment of sport, and all without getting injured – we are presented with a complex problem. It is also worth noting, however, that open kinetic chain exercises, such as leg flexion, have little effect on performance of sport specific movements (Augustsson et al. 1998; Blackburn and Morrissey 1998; Augustsson and Thomee 2000), but may help to address muscle imbalances.
Training that does not relate directly to the movements and activity patterns found in sport, is a waste. Unfortunately, many athletes still employ training methods that are non-sport specific. These methods are often prescriptive, predictable and sterile. Many are based on one-size-fits-all training systems reported to be the secret of another athlete’s success. Many lack sufficient variety, using repetitive schedules that soon lead to a plateau in performance. Most fail to account for the fluid, unpredictable and three-dimensional nature of sport. In essence, they fail to stimulate athletes in a truly sport specific way.
Despite the best intentions of coaches and athletes, too much conditioning time and energy is directed towards superfluous, non-specific activity. For example, when strength training, many athletes monitor their progress by how much they can squat or bench press, and focus training towards these exercises. Sport, however, typically requires strength to be expressed in a faster, more dynamic, and in a three-dimensional fashion. Although a relationship has been demonstrated between squat strength and both sprint speed and vertical jump height (Wilsoff et al. 2004), and heavy bench press appears to be an appropriate pre-load activity for performing complex training on functional movements (Matthews et al. 2009), squatting and bench press alone do not prepare an athlete for all movement patterns required in sport. Too much training time devoted to non-functional movements takes training focus away from sports specific activities and causes extra fatigue, forcing the body to recover from training that does have a direct affect on performance. However, the reverse is also true, where athletes spend too much time focusing on sports specific training and lack adequate strength and power development required to produce an increase in sports performance. Less sports specific movements, however, can provide a good solid base (general preparation phase) from which an athlete can progress to more specific conditioning (pre-competition and peaking phases) (see Chapter 9: An Introduction to Periodisation).
To condition athletes effectively, training must mimic the conditions encountered in sport. To achieve this, programme designers must: (1) analyse the demands of the sport; (2) identify the individual characteristics of the athlete (strengths and weaknesses; training history); and (3) tailor and prioritise training to allow each individual athlete to meet these specific demands.
It is essential that the training programme is specific in terms of the metabolic demands (energy systems) (see Chapter 3; Needs Analysis section) and mechanical demands (muscle action, force, velocity, range of motion).
Mechanical demands
The mechanical demands of sport determine the movements that athletes should train. Exercises that mimic the actual movements encountered in sport should be prioritised. By focusing on movement pattern specificity athletes can reinforce and condition the actual motor programmes used in skilled performance. These programmes control the precise order, timing, velocity, force application and muscle action to enable the muscles to produce a predetermined movement (Enoka 1994). The more practiced and efficient these programmes, the better the performance of the skill. For example, a rugby player who focuses practice on the foot patterns required to side step an opponent can enhance side-stepping performance by executing quicker, more efficient motor programmes. However, it is also worth noting that if the athlete does not have the eccentric strength to enable them to decelerate, or the power to re-accelerate in another direction practising footwork will have little effect.
The best training for movement pattern specificity is to practice the actual skills involved in sport, however, ensuring progressive overload in these activities is not possible. Repetition of the skill is possible for many sports; however there are sports and situations where it is not practical or safe to perform high volumes of the specific patterns, frequently. A triple-jumper or long-jumper, for example, who only trains by performing the actual jump skills would soon breakdown due to the constant repetition of intense impacts. For these athletes other, less intensive, methods should be used that mimic closely the actual movements involved. These methods focus on the ranges, speeds and forces encountered, allowing the development of fitness attributes in a functional way that may ultimately allow athletes to tolerate more actual (jumping) practice. For example, heavy force-acceptance strength training, fast force-acceptance strength training, dynamic control and stabilisation training, along with specific jumping drills will increase an athlete’s capacity to tolerate actual skill practice in the highly intensive triple jump.
The training methods that transfer best to actual sporting performance usually involve coordinated movements across multiple joints rather than strict isolation exercises. In sport, no muscle works in isolation. Isolating specific muscles, then, is non-functional; gains in strength, power, or endurance occur only in the trained muscle and fail to integrate with the whole movements required for sporting performance. Consideration of how to train movements, not muscles is essential. Training that focuses on the whole movement enhances functional performance more effectively than the training of isolated joint movements; integrate, don’t isolate.
For example: A sprint start requires rapid and forceful triple extension of the hip, knee, and ankle, along with rapid flexion at the shoulder on the same side and rapid extension on the opposite side, plus a torso that is rigid enough to control and transfer forces from one body segment to another. Athletes must therefore train movements that target these areas. Multi-joint all-body dynamic movements that are uni-lateral or cyclic (one legged jump squats; split jump lunges) are far more specific than single joint bi-lateral isolation exercises performed slowly (leg extensions; leg curls). Traditional basic strength exercises, like back-squats and squat-jumps, may not be cyclical, but allow the athlete to focus on generating maximal forces as explosively as possible, as required in the initial strides during a sprint start. They are sport specific in terms of being multi-joint triple extension exercises and forces generated, however they are non-sport specific in terms of their velocity and their bi-lateral nature. Typically, sporting movements involve standing or running, producing and accepting forces in multiple directions and planes, and at various speeds, all in a fluid and ever-changing environment. Training should mimic this. Exercises that require athletes to stand on their feet are always more specific to sport than exercises that require them to sit or lie. Sport is not played lying down. Equally, exercises that involve multiple joints resemble sporting movements far better than those involving single joints. As previously mentioned it is essential to note that single joint exercises have little effect on performance of sports specific movements (Augustsson et al. 1998; Blackburn and Morrissey, 1998; Augustsson and Thomee, 2000).
Focusing training towards the actual mechanics of the movements (magnitude of force, direction of force, velocity of movement, muscle actions involved, level of stability required, etc.) required by sport is clearly paramount to optimal conditioning. In sport, however, success rarely relies on the execution of one single discreet movement; there are always other movements, either preceding or following the current one. One focus (and often neglected area) of training is training athletes to transfer fluidly and efficiently from one movement to another. In sport, no movement ever stands alone. For example, a tennis serve appears to be a one-off stand-alone skill; however this is not the case. Players must be ready to sprint or lunge to any point on the court to intercept the return ball. The follow through from a serve will merge with the next skill, and the more efficiently this occurs the quicker a player can intercept and play the next shot. Equally, if a tennis player can explode laterally from a sideways lunge position (a forehand on the edge of the court) then they stand a better chance of reaching the next shot. If prior to this, however, they exhibit inefficient deceleration mechanics and poor force-acceptance going into the shot, then the whole movement will be slower.
Success in sport, then, requires athletes to train in a sport specific way. By analysing the movement patterns (force, velocity, muscle action, joint angles) involved in sports and replicating those in training, athletes can not only develop strength, speed, power, endurance, and flexibility in a truly functional way, but also integrate and perfect combinations of movements to replicate typical patterns of play.
As well as training to produce force we must also focus on the athlete’s ability to accept force. Sport requires athletes to reduce and absorb external forces often at high speeds, in three dimensions, and in an unpredictable fashion. Athletes must train for deceleration and force-acceptance as well as force production. This will prepare athletes to meet the varied stresses of sport and have a major impact on injury prevention.
For example: The hamstrings are typically trained concentrically using knee flexion exercises (leg curls) and hip extension exercises (Romanian deadlifts) (Figure 13.13). In function, however the hamstrings also act eccentrically to control and decelerate the limb (as in kicking a football) (Smith, 1999), act antagonistically to the rectus femoris to prevent hip flexion (as in squatting or jumping), and act eccentrically as an ACL agonist by preventing anterior tibial translation thereby reducing sheer forces and increasing knee stability (Aagaard et al. 1998; Li et al. 1999; Escamilla et al. 2001; Kingma et al. 2004; Ahmed et al. 2006). Movements that target these attributes may include jumps, one legged squats and squat jumps, and practice kicks increasing progressively in terms of both velocity and amplitude. Prior to this, however, the hamstrings must be appropriately conditioned / strengthened concentrically, and eccentrically at low velocities (for example, using Nordic hamstring lowers) (Figure 13.14).
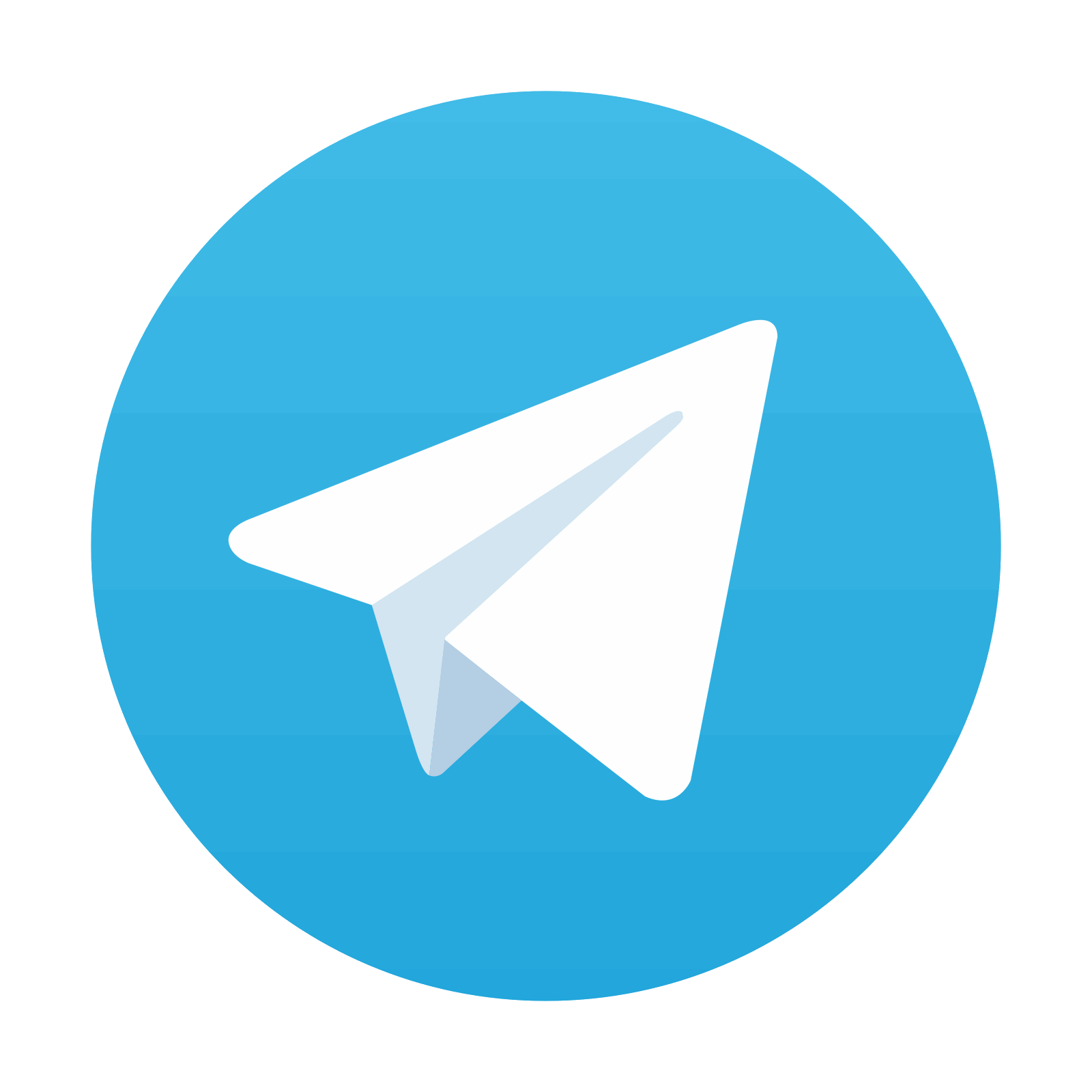
Stay updated, free articles. Join our Telegram channel

Full access? Get Clinical Tree
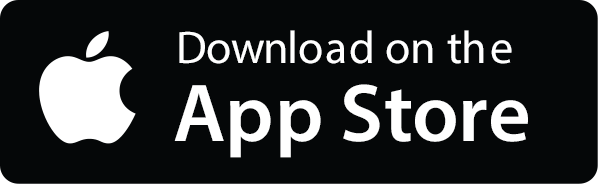
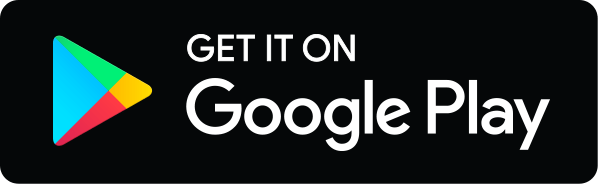