23 SPINAL CORD INJURIES
Spinal cord injury (SCI) was first described in the literature in 2500 BC by Egyptian surgeons as “an ailment not to be treated.”1 This sentiment was echoed for the next several thousand years. It was not until the early 1900s when Dr. Alfred Reginald Allen developed an experimental SCI model that permitted reproducible injury that “modern-day” understanding of spinal cord injury was elicited. This model allowed Allen to study the outcomes that resulted when the spinal cord was subjected to varying forces. These experiments led to his hypothesis that traumatic SCI created two types of injury: (1) direct injury to the axons at the time of impact (primary SCI) and (2) a response of the affected nervous tissues to the initial injury that occurs over time and causes delayed cellular damage (secondary SCI). Research has consistently supported the concept of primary and secondary injury. The potential to interrupt this secondary response and prevent worsening outcome prompted early, aggressive treatment of SCI.2,3
A combination of many events in the late 1960s and early 1970s led the way in changing the approach to management of acute spinal injury. New technologies, such as the intraoperative microscope and neuroradiologic diagnostics, and the development of modern intensive care units have all contributed to survivability.2 Introduction of the military model for triaging and rapid transport of trauma victims and the understanding of the multisystem effects of SCI enabled many more paralyzed individuals to survive their injury. This demonstrated both the ability to successfully treat such injuries and highlighted the need for appropriate SCI rehabilitation. The pioneering work of Sir Ludwig Guttmann identified the need to provide comprehensive multidisciplinary care from the onset of SCI through rehabilitation so that individuals can return as productive members of society. Guttmann’s holistic approach serves as the model for modern-day SCI care.
EPIDEMIOLOGY
It is estimated that there are approximately 40 new cases of SCI per million U.S. citizens each year; this translates to 11,000 new cases per year.3–6 Researchers estimate that an additional 4800 victims of SCI die before reaching the hospital.3 It is currently estimated that between 250,000 and 400,000 individuals in the United States are living today with SCI or dysfunction.4–6 This broad range reflects the difficulty in capturing accurate data caused by the complexity of ICD-9 codes and lack of discharge coding and reporting.4 Recent funding within several states may support improved data collection.
While the incidence of SCI is relatively low, it is considered a high-cost disability. Depending upon age at the time of injury, average lifetime direct cost is estimated at $1.6 to $2.8 million for high tetraplegics (C1-4), $1 to $1.6 million for low tetraplegics (C5-8), and $450,000 to $1 million for paraplegics.5 A conservative estimate of the economic burden of SCI in the United States is $7.13 billion per year.6,7 This includes the cost of newly injured persons, acute and rehabilitation care, home environment adaptation, and care for chronic issues such as pressure sores, which alone cost on average $1.2 billion yearly.8 This does not reflect indirect costs, such as loss of wages, fringe benefits, and productivity, which represent an additional $2.6 billion dollars annually.6,7
In 1973, the National Spinal Cord Injury Database was established at the University of Alabama at Birmingham. This registry captures approximately 13% of new SCI cases per year.4 Because this group is representative of the SCI population at large, it has contributed significantly to our ability to compile incidence, prevalence, and demographic information on SCI. Since 2000, the average age at the time of SCI has risen to 37.6, up from 28.7 in the 1970s.4,8 This trend reflects the median age increase in the population at large since the 1970s. Males continue to sustain SCIS more often than females by about a 4-to-1 margin, and the primary etiology remains motor vehicle crashes. Demographics are showing shifts in several areas: (1) an increase in the proportion of those injured who are older than age 60, again matching a rise in the median age of the general population; (2) a rise in the proportion of injuries among African-Americans (increasing from 13% in the 1970s to 22% since 2000) and Hispanics (increasing from 6% in the 1970s to 12.6% since 2000), paralleling the increase of these racial and ethnic groups in the composition of the population at large; (3) a rise in the incidence of falls, especially as age increases; and (4) more SCIs due to violent events, particularly in young African-American males.4,8,9
In years past, the average life expectancy of a person sustaining an SCI was less than 1 year. With the improvements made in prehospital, critical, and long-term care, life expectancies for persons with SCI that survive the first year are continuing to increase.8,9 Because patients are living longer, more of them will receive Medicare and disability income. We can expect a continued increase in the annual expenditure for SCI-related health care.7 There also has been a shift in the leading causes of death for patients with SCIs. Historically, the leading cause of death was renal failure, although with significant advances in urologic management, this etiology has declined. The most common causes of death have become pneumonia, heart disease (54% were sudden, unexplained heart attacks), and septicemia, most frequently related to pressure ulcers, urinary tract infections, or pneumonias.4,9,10 Recent prospective mortality studies have disputed the long-held belief that level and extent of SCI, age at injury, and calendar year of injury are directly related to mortality.10 Interestingly, comorbidities affecting mortality rates are the same as those without SCI: heart disease, diabetes, pulmonary function abnormalities, and cigarette smoking.10,11 It is crucial that care during the cycle of trauma be focused on limiting complications to achieve maximum recovery and sustain optimal health.
PREVENTION
There are three general strategies to prevent injuries: persuasion, legal requirements, and provision of automatic protection. Persuasion is the most difficult strategy to apply effectively. The Think First Foundation (www.ThinkFirst.org), developed and supported by the American Association of Neurologic Surgeons and the Congress of Neurological Surgeons, is one group that assists communities in providing programs to educate children and adolescents about prevention of brain and spinal injuries. Also, changes in laws creating safer vehicles and more effective restraint systems and enforcement of these laws can help protect the public. Mandatory safety equipment for high-impact sports, particularly improved helmets and neck rolls, can help prevent SCI. Aggressive campaigns, such as those sponsored by Mothers Against Drunk Drivers (MADD), provide media attention and public education regarding the dangers of high-risk behaviors and promote social reform, which can be effective in reducing SCIs. The number one cause of SCI in persons age 65 and older is falls.4,5,11,12 Statistically, one third of persons older than age 65 will sustain a fall resulting in a significant injury. Of this group, two thirds will sustain a second fall within the same year.12 Fall prevention programs for the elderly should start with risk assessment of both the person and the living environment. The Centers for Disease Control and Prevention (CDC) and the National Center for Injury Prevention have published the U.S. Fall Prevention Programs for Seniors that provides a listing of available comprehensive programs in addition to the Senior Fall Prevention Tool Kit. Raising public awareness, providing education, using passive protective mechanisms, and enacting social and legal reform can be effective prevention methods.
BASIC SPINAL ANATOMY
THE BONY STRUCTURES
The spinal, or vertebral, column consists of 33 vertebrae (Figure 23-1) separated and cushioned by fibrocartilaginous pads called intervertebral disks. The vertebrae and disks are joined by ligaments.
The majority of the vertebrae have similar anatomic features. Each vertebra can be divided into two sections: an anterior body, the segment that faces toward the front of a person, and the posterior arch (Figure 23-2). The opening created between the body and the arch is the spinal canal (vertebral foramen), where the spinal cord, its coverings, and blood vessels pass. The arch is a series of fused bony parts. Starting at the midpoint is the spinous process. These are the points that can be seen or felt protruding along the midline of the back. Moving laterally in either direction are more distinct bony prominences called the transverse processes with superior and inferior articular processes or facets. These are joined to the spinous process by the lamina. The transverse processes are connected to the vertebral body by the pedicles. When the vertebrae are aligned in a column, the inferior articular processes, which project downward, meet the upward protruding superior articular processes of the vertebrae directly below. Between these processes and the bodies and disks an opening exists—the intervertebral foramen, through which peripheral nerves pass.
Of special interest are the first cervical (C1) (atlas) and second cervical (C2) (axis) vertebrae (Figure 23-3). The first cervical vertebra is called the atlas because it literally supports the “globe” of the head. By articulating with the skull, C1 provides 50% of the normal motion of the neck, mainly flexion and extension. It is an unusual vertebra in that it lacks a vertebral body. The odontoid, a bony projection jutting upward from the C2 vertebral body, protrudes through the anterior arch of C1. The odontoid process forms the pivot on which the atlas and skull rotate (hence the term axis). The sacrum and coccyx are also unique because the vertebral bodies of these areas are fused together.
The intervertebral disks, made up of an inner gelatinous material (nucleus pulposus) surrounded by cartilaginous fibers (annulus fibrosus), confer a degree of flexibility to the spine. They act as shock absorbers by temporarily flattening and bulging from between the vertebrae when they are compressed. The vertebrae and disks are held in alignment by ligaments (Figure 23-4). The ligaments prevent extreme flexion and extension of the spine. The anterior and posterior longitudinal ligaments join the bodies of adjacent vertebrae. whereas the strong ligamenta flava join the lamina. The ligamentum nuchae extends from the skull through the spinous process of the seventh cervical vertebra. Below this level, supraspinal ligaments join the spinous processes. Adjacent spinous processes are joined from their roots to their ends by interspinal ligaments.
THE SPINAL CORD
Spinal nerve roots exit from the cord at each intervertebral foramen. There are a total of 31 pairs of spinal nerves: 8 cervical, 12 thoracic, 5 lumbar, 5 sacral, and 1 coccygeal. Each spinal nerve has a dorsal (posterior) root and a ventral (anterior) root (Figure 23-5). The dorsal root consists of sensory or afferent fibers, which carry impulses from the body to the cord. The dorsal root contains a spinal ganglion, which is located outside the cord but within the intervertebral foramen. The ganglion contains the cell bodies of the sensory neurons. The ventral root consists of the motor, or efferent, fibers. The cell bodies of the motor neurons are located within the ventral horn (anterior gray matter column) of the cord. These fibers carry the impulses from the cord to the appropriate effector site.
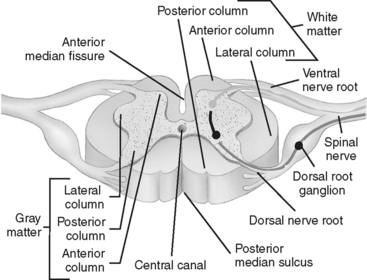
FIGURE 23-5 Transverse section of spinal cord.
(From Ozuna JM: Nursing assessment nervous system. In Lewis SM, Heitkemper MM, Dirksen SR, editors: Medical Surgical Nursing Assessment and Management of Clinical Problems, ed 6, St. Louis, 2004, Mosby.)
GRAY AND WHITE MATTER
The substance of the spinal cord is divided into two types: gray matter and white matter.
The gray (unmyelinated) matter is centrally located in an H or butterfly-shaped pattern. It is composed of cell bodies and their axons and dendrites. It can be divided into three areas, columns, or horns: the anterior, the intermediolateral, and the posterior. The anterior horn provides the motor component of the spinal nerves, often referred to as the final common pathway. The intermediolateral horn contains cell bodies, which give rise to the preganglionic sympathetic fibers of T1-L2 and the preganglionic parasympathetic fibers of the sacral segments of the cord. The posterior horn contains axons from peripheral sensory neurons. These may terminate there or ascend or descend in the white matter.
The white (myelinated) matter surrounds the gray matter of the cord and is divided into large fiber bundles called columns or funiculi. There are three columns: the posterior, the lateral, and the anterior. Each column contains ascending sensory tracts and descending motor tracts (Figure 23-6).
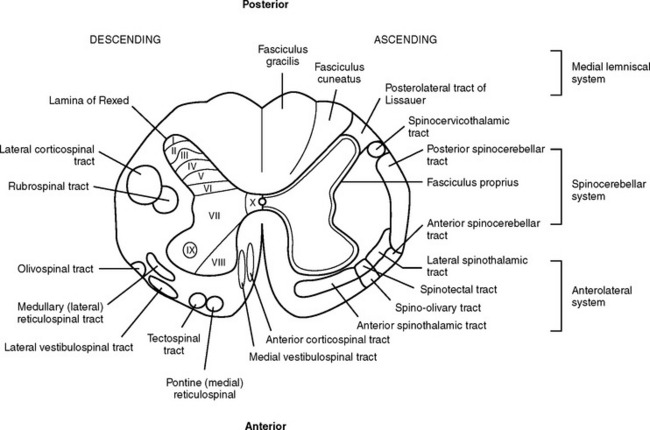
FIGURE 23-6 Cross-section of spinal cord detailing major descending (motor) and ascending (sensory) tracts.
The major ascending tracts include the posterior (dorsal) columns, the spinocerebellar tracts, and the spinothalamic tracts. The posterior column mediates proprioception, vibration, two-point discrimination, deep pressure, and touch.13 These columns ascend on the ipsilateral side of the spinal cord, where they enter and decussate in the medulla and become the internal arcuate fibers of the medial lemniscus; these fibers ascend through the thalamus to the cerebral cortex.14 There are many well-identified ascending tracts of the lateral column. The most significant of these are the spinocerebellar tracts and the spinothalamic tracts. The spinocerebellar tracts carry information on position sense and body movement (unconscious proprioception) necessary for coordination of body movement from the extremities and trunk to the cerebellum. The spinothalamic tracts carry information from the periphery to the thalamus. The anterior spinothalamic tracts transmit light touch and pressure impulses, whereas the lateral spinothalamic tract mediates most pain and temperature sensations. The spinothalamic tracts decussate almost at the level of entrance into the spinal cord and ascend in the contralateral anterolateral system to the thalamus. The anterior column tracts are primarily involved with motor function, posture reflexes, light touch, and pressure.
The descending tracts or motor pathways can be divided into two categories: upper motor neurons (UMNs) and lower motor neurons (LMNs). UMNs originate and terminate within the central nervous system (CNS) and include all neurons or nerve cells, which modulate the motor output of the anterior horn cell of the spinal cord. The motor modulators that constitute the majority of the UMNs include the cerebral cortex, cerebellum, basal ganglia, red nuclei, vestibular nuclei, and reticular formation. Any lesion or trauma that disrupts these areas and their modulation of the LMNs will create an upper motor neuron deficit. This deficit is characterized by paralysis, hypertonicity in affected muscle groups, and hyperreflexia.
In summary, the primary motor tracts are located in the anterior and anterolateral portion of the spinal cord, and the primary sensory tracts are located in the posterior and posterolateral section of the cord (see Figure 23-6). This generalization helps provide an understanding of incomplete spinal cord syndromes.
SPINAL BLOOD SUPPLY
Branches from the terminal portion of the vertebral artery unite at the level of the foramen magnum to form the anterior spinal artery (Figure 23-7). The artery descends down the median ventral aspect of the spinal cord, supplying blood to its anterior two thirds. The remaining blood supply is provided by the two posterior spinal arteries, which descend down the posterolateral aspect of the spinal cord from their vertebral artery source. As these three vessels descend the cord, they receive additional perfusion from branches of the cervical, intercostal, lumbar, and sacral arteries. The large artery of Adamkiewicz, which arises from the aorta, enters the cord at the second lumbar ventral root level to supply blood to most of the lower third of the spinal cord.13
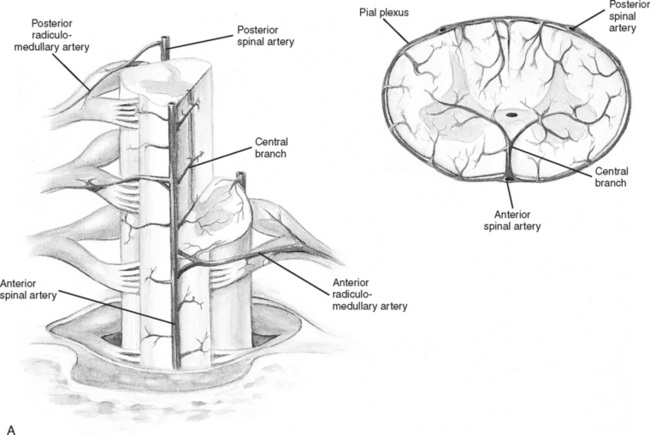
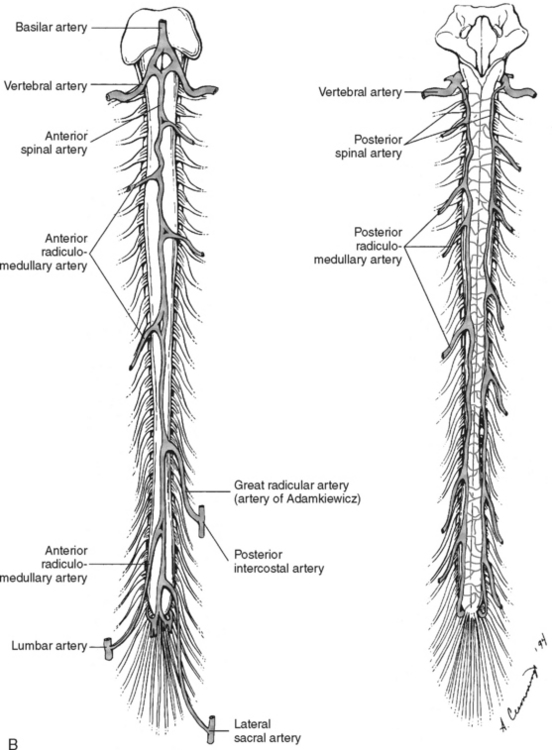
FIGURE 23-7 Arterial circulation of the cord. A, Cross-sectional views. B, Anterior and posterior views.
(From Darby SA. General anatomy of the spinal cord. In Cramer GD, Darby SA, editors: Basic and Clinical Anatomy of the Spine, Spinal Cord and ANS, ed 2, St. Louis, 2005, Elsevier Mosby.)
Venous drainage of the spinal cord consists of two main systems: the intrinsic system and the extrinsic system. The intrinsic, or intradural system, closely follows the arterial system to drain blood from the spinal cord emptying into the venous plexus surrounding the cord (venous vasa corona). The extrinsic, or extradural, system forms a series of plexus from the craniooccipital junction to the sacral region. All venous drainage ultimately ends up in the vena cava.14
THE AUTONOMIC NERVOUS SYSTEM
The autonomic nervous system (ANS) controls and regulates the functioning of the involuntary muscles and glands of the major body systems. It is regulated by the hypothalamus, which is located at the crossroads of the ascending and descending spinal tracts in the diencephalon. The ANS is divided into two antagonistic branches: the sympathetic branch (SNS) and the parasympathetic branch (PNS).
The SNS controls functions commonly referred to as the “fight or flight” response. It exits the spine in the thoracolumbar area and prepares the body to respond to stressful situations. The parasympathetic branch is responsible for energy conservation and system relaxation. The parasympathetic system, including the third, seventh, ninth, and tenth cranial nerves, exits the spine at the cervicosacral level. This information is important in understanding the systemic consequences of a spinal cord injury (Figure 23-8 and Table 23-1).
TABLE 23-1 Autonomic Nervous System: Effects on Various Effector Sites
Effector Organ | Sympathetic Influence | Parasympathetic Influence |
---|---|---|
Eye | ||
Pupil | Dilation (mydriasis) | Constriction (miosis) |
Glands | ||
Lacrimal | Decreased | Increased |
Nasal | Decreased | Increased |
Salivary | Decreased | Increased |
Sweat | Increased | None |
Heart | Increased rate | Decreased rate |
Increased conduction velocity | Decreased contractility | |
Increased contractility | ||
Blood vessels | ||
Coronary | Vasodilation | Minimal dilation |
Skeletal | Vasodilation | None |
Abdominal viscera | Vasoconstriction | None |
Cutaneous | Vasoconstriction | None |
Blood pressure | Increased | Decreased |
Lungs | Bronchodilation | Bronchoconstriction |
Gastrointestinal | ||
Motility | Decreased peristalsis | Increased peristalsis |
Sphincter | Increased tone | Relaxation |
Secretions | Inhibition | Stimulation |
Bladder | Decreased detrusor tone | Increased detrusor tone |
Sex organs | Ejaculation | Erection |
Skin | ||
Pilomotor muscles | Excited (contraction) | None |
Modified from Guyton AC, Hall JE: Textbook of Medical Physiology, ed 9, Philadelphia, 1996, W.B. Saunders.
MECHANISM OF INJURY
The primary mechanisms that result in spinal injury are hyperflexion, hyperextension, axial loading (vertical compression), rotation, and penetrating trauma (Figure 23-9). Many injuries involve an exaggerated movement in one direction (e.g., hyperflexion), but usually there is a degree of accompanying injury on the opposite side (e.g., extension) as the articulated spine responds to the extreme motion. Forces that cause injury may impact the spinal column simultaneously or in succession.15 Severity of injury is directly related to the magnitude of the force(s); in other words, the greater the force, the greater the injury.16
HYPERFLEXION
Hyperflexion injury occurs when a portion of the spine receives a force, direct or indirect, exerted toward the anterior surface of the vertebral body, causing flexion beyond the normal range of motion. This is often associated with head-on collisions. On impact, the driver’s head continues forward, and with sufficient speed and force, the weight of the head forces the chin to the chest, causing a hyperflexion injury. In hyperflexion injuries the most flexible levels of the spine absorb this energy and act as fulcrums. This extreme force and stretch can cause an anterior vertebral compression (wedge) fracture and possible intervertebral disk herniation.16 Posterior ligaments are stretched and may tear, allowing the vertebrae to sublux. Subluxation of the vertebral column disrupts the continuity of the spinal canal, stretching and impinging on the spinal cord and altering spinal cord blood flow. Disk matter can also extrude into the spinal canal toward the cord.
HYPEREXTENSION
Hyperextension injury results when there is extreme extension of the spinal column, such as in a fall, when the chin strikes an immovable object, or in a rear-end collision. Whiplash is a mild form of this mechanism. Once again the extreme force is absorbed by the flexible points of the spine, producing a stretch or tear of the anterior longitudinal ligaments, possible fracture, and subluxation of the vertebrae and rupture of the disks. If the integrity of the spinal canal is compromised, the spinal cord may be damaged. Injury also occurs when the hyperextended spinal cord is stretched excessively so that it is compressed by the ligamentum flavum, causing cord contusion and ischemia.13,17 Persons with preexisting conditions, such as degenerative cervical stenosis, are at greater risk for developing SCI as the result of hyperextension because of the presence of osteophytes and narrowing of the spinal canal.17
AXIAL LOADING
Axial loading, also referred to as vertical compression, occurs when sufficient force is exerted vertically through the spinal column. The vertebral bodies and intervertebral disks attempt to absorb the energy and literally burst. This is known as a compression or burst fracture. Bone fragments and disk matter are sent in all directions, including into the spinal canal.13 The imploding bone can tear and compress the spinal cord, which it surrounds. Additional “buckling” of the cervical spine involving a combination of flexion and extension at various vertebral levels may also occur.18 Injury from this mechanism often occurs with shallow diving or head-first tackling and generally results in significant SCI.
ROTATIONAL INJURIES
Rotational injuries are the result of twisting the spine. Lateral flexion of the cervical spine is accompanied by axial rotation. Rotational forces may stretch and rupture the posterior ligaments, dislocate the facets, and may cause compression fracture of the bony structure.15,16 This injury may occur as a result of a side impact motor vehicle crash, particularly when occupants are unrestrained, or when force is exerted to the side of the head or jaw, such as a “hook” blow in boxing.
SPINAL COLUMN INJURIES
SOFT TISSUE INJURIES
Injuries can affect the soft tissues that surround and support the spine, including the muscles, disks, and ligaments. Under an extreme load, the central nucleus can burst through the surrounding cartilaginous fibers, the annulus fibrosus, producing a herniated disk. Disk injury can compress and injure the spinal cord or nerve roots. Disruption of spinal ligaments can threaten vertebral column stability, allowing vertebrae to dislocate and injure the underlying spinal cord. Whiplash is a common term used to describe a hyperextension injury of the neck that causes stress and strain injury to neck ligaments and muscles.13 Symptoms, including headache, stiff neck, neck and shoulder pain, muscle spasm, and limitation of movement, are thought to be caused by the stretching, microhemorrhage, and edema incurred by the neck muscles.13 The results of physical examination are usually normal except for the above symptoms, and radiologic findings are negative. Palliative treatment measures generally include mild analgesics, nonsteroidal antiinflammatory drugs (NSAIDs), heat therapy, muscle relaxants, and exercise regimens.13,19,20 Cervical collars, although controversial, may be used.13,19,21,22
VERTEBRAL INJURIES
Wedge Fracture
Wedge (compression) fracture occurs when the anterior portion of the vertebral body becomes compressed by the force exerted on it by adjacent vertebrae.16,17 If the posterior elements remain intact, the fracture is stable. The spinal cord may or may not be spared. It is most often associated with axial loading and hyperflexion injury.13,15–17
Burst Fracture
Burst fracture results from vertical forces directed onto the spinal column that shatter the vertebral body and are frequently associated with intervertebral disk rupture. Bone fragments and disk material may be driven into the spinal canal, producing serious spinal cord compromise.13 Management is based on characteristics and stability of the fracture and presence or absence of neurologic deficit. Patients without deficit may be conservatively managed with bracing or casting.17,23–25
Teardrop Fracture
Teardrop fracture, usually associated with a compression-flexion force, occurs when a small fragment of bone from the anterior edge of the vertebra breaks off.16,17 The vertebral body may be dislocated posteriorly into the spinal cord. In addition, interspinous separation (distraction) posteriorly and fractures of the lamina and spinous processes may occur.16 Surgical intervention is required to remove the fragment and stabilize the spine.
Dislocation
Dislocation injury, a result of distractive-flexion force, occurs when one vertebrae subluxates over another, often resulting in unilateral or bilateral facet dislocation. The injury is staged based on the degree of vertebral involvement (fracture and displacement) and ligamentous disruption.16,17 Accompanying ligamentous injury ranges from stretch or strain to complete rupture of the stabilizing ligature. Management is dependent upon severity of fracture and degree of displacement.17
Fracture-Dislocation
Fracture-dislocation is a term used to convey the presence of a vertebral break in combination with displacement of the vertebral body. It is associated with ligamentous injury, possible disk herniation, and usually involves spinal cord injury. Fracture-dislocation injury results from shearing force in combination with another injury mechanism, such as axial rotation or flexion.16,17 This complex and unstable injury usually requires surgical fixation for stabilization.
High Cervical Fracture
Atlantooccipital dislocation (AOD) results when the occiput is avulsed from the atlas. Injury to the spinal cord and brainstem occur, causing this injury to be fatal in most cases. In addition to bony disruption and neural damage, impingement of the vertebral artery may occur. Surviving patients may have variable motor and sensory deficits, cranial nerve defects, and cardiopulmonary instability. Management of these injuries is directed toward immediate stabilization and reduction of the spine.26,27 Early fusion and application of a halo apparatus are recommended.26,27
A Jefferson fracture is the result of vertical compression of the atlas ring. As the vertebra absorbs the force, it is split, or burst, into several parts. The fractures permit widening of the vertebral foramen, and therefore the spinal cord usually remains undamaged. However, any movement of the head can displace a fragment and sever the cord, which often results in death. Literature generally supports conservative management with a rigid stabilization, such as a halo apparatus, for up to 12 weeks, if the transverse ligament is intact.28,29 Patients with ligamentous rupture may require surgical intervention with subsequent rigid stabilization.28,29
An odontoid or dens fracture of the C2 vertebrae is a rather common traumatic cervical injury.29–31 It usually results from multiple extreme forces, including compression, extension, rotation, and flexion.30,31 These fractures can be classified according to location of the dens fracture (Figure 23-10). The majority of these fractures are not associated with neurologic deficit. Odontoid fractures are best visualized radiographically with lateral or open-mouth views of the cervical spine. Management is dictated by the type of fracture and degree of dens displacement.30 External cervical immobilization may be used initially to manage type I, II, or III fractures; however, surgical fixation should be considered if a type II or III fracture is comminuted, significantly displaced, or alignment cannot be obtained and maintained with cervical immobilization.29,30 Persons older than age 50 with type II injuries should be considered for a combination of surgical and external stabilization.30
Traumatic spondylolisthesis of the axis, commonly referred to as hangman’s fracture, is characterized by fractures through the neural arch and pedicles of the axis (C2) with possible displacement of C2 on C3. Given the large vertebral foramen of the high cervical spine and because the anterior and posterior elements generally separate, the majority of these fractures are without neurologic impingement. Treatment will vary depending on the severity of vertebral angulation, distraction, and facet dislocation.30 Many fractures can be treated conservatively with external immobilization.30,31
SPINAL CORD INJURIES
The concept of primary and secondary injuries, first proposed more than 80 years ago, has emerged as an explanation for the phenomenon of delayed neural injury.32–34 A primary injury is the mechanical disruption that occurs to the spinal cord substance at the time of injury, whereas the progressive pathologic responses that occur subsequently over hours to days are known as secondary injury.13,32–35 A number of factors, such as hypoxia and hypoperfusion, are known to trigger or exacerbate secondary SCI and worsen outcome. Although there is little that can be done to change the circumstances of the initial trauma, the outcome of the secondary injury may be amenable to therapeutic intervention. The intrinsic responses occurring during SCI have been well documented.32–35 Understanding the cascade of events that ultimately lead to cellular death (necrosis) and the initiation of apoptosis is key to understanding the use of investigational agents to interrupt the sequence of biochemical interactions and improve neurologic outcomes (Figure 23-11).
Classification by Type of Injury
Types of SCI include concussion, contusion, laceration, transection, hemorrhage, and vascular disruption. A concussion of the spinal cord causes a temporary loss of function lasting no more than 24 to 48 hours. It produces a transient dysfunction of the spinal cord from jarring forces. A contusion of the spinal cord is actual bruising of the neural substance. It includes the intrinsic responses to bleeding, including edema, compression, ischemia, and possibly infarction. The severity of this injury depends on its size, location, and the physiologic response to the bleeding. A laceration is an actual tear in the cord, which results in permanent injury. Surrounding contusion and the normal physiologic response to injury accompany a tear. A transection is a complete severing of the neural elements. Complete transection results in the spinal cord being physically separated into two distinct pieces; this is a rare finding. However, in functional terms, a complete transection is frequently the result of significant injury. Hemorrhage into or around the spinal cord can compress the neural substance and initiate the intrinsic cascade. This may produce ischemia and neurologic deficits. Vascular disruption to the spinal cord causes a loss of blood flow to the neural substance and results in ischemic changes. The duration of ischemia is directly related to the neurologic outcome.32–34
Classification by Function
SCI can be classified as either complete or incomplete, according to functional outcome. In complete SCI, there is loss of all voluntary muscle control and sensation at and below the level of the lesion. Per the American Spinal Injury Association (ASIA), a complete SCI is defined as a loss of all voluntary muscle control and sensation in the sacral segments (S4-5); this definition simplifies classification of patients with asymmetric presentation or zones of partial preservation. Clinical implications depend on the level of injury. Injuries to the cervical spine, especially at and above C4, are typically the most life threatening secondary to the potential loss of diaphragmatic innervation and respiratory failure (Figure 23-12). Several functional outcome scales exist; the ASIA impairment scale, which uses an alphabetical system of A (complete) through E (normal), and the Functional Independence Measure (FIM), which assigns numeric value to specific functional tasks, are two of the most commonly used. Functional outcomes are covered in greater detail in the rehabilitation phase.
Classification by Spinal Cord Injury Clinical Syndrome
Anterior Cord Syndrome.
Anterior cord syndrome is usually associated with flexion-dislocation injuries and may be associated with acute disk herniation.13 An injury to the anterior part of the spinal cord or interruption of blood supplied by the anterior spinal artery results in ischemia to the anterior gray horn and the anterolateral columns of the white matter. There is usually complete motor loss accompanied by a loss of pain, temperature, and touch sensation at and below the level of the lesion. Light touch, proprioception, and vibration senses are preserved.13,35 (Figure 23-13, A).
< div class='tao-gold-member'>
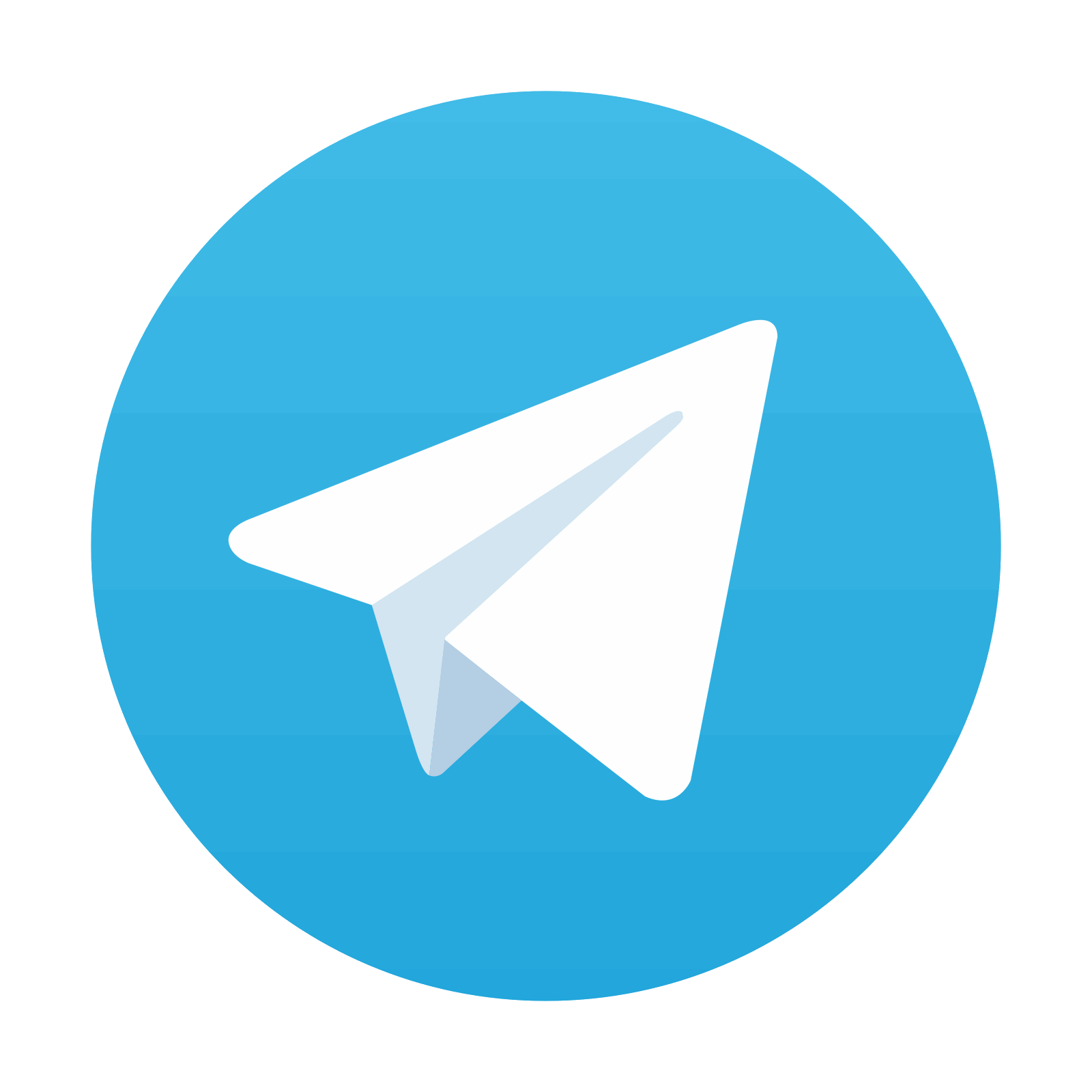
Stay updated, free articles. Join our Telegram channel

Full access? Get Clinical Tree
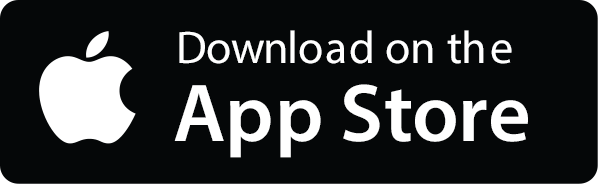
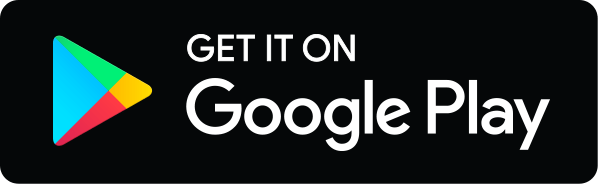