32 BURN INJURIES
Fire and burns are the fifth most common causes of unintentional injury-related deaths in the United States.1 According to the American Burn Association (ABA), approximately 1.1 million burn injuries require medical attention each year; 50,000 of these individuals require hospitalization and 4,500 die from their burn injuries.2,3 Approximately three fourths of fire-related civilian deaths and injuries are the consequence of house fires, resulting in 2,670 deaths, 14,050 injuries, and $6.1 billion in direct property damage.4 Of the approximately 6% of burn injury patients who do not survive, most have sustained an inhalation injury. Despite these sobering facts, overall mortality and morbidity rates from burn injuries have declined over the years; however, burn deaths, injuries, and disability from burn injury remain a substantial problem in our society.
Advances in burn prevention strategies, such as effective public education, better fire-retardant product design, smoke detectors, and enforcement of fire safety codes4,5 have decreased the overall prevalence and incidence of burn injuries. Survival after burn injury has also improved over the past few decades. Before the 1970s, a patient with a 50% total body surface area (TBSA) burn had a 40% chance of survival. Today a patient with a 75% TBSA burn has a 50% chance of survival.6 Factors enhancing patient survival may include more rapid response by emergency teams, efficiencies in transport to burn treatment facilities, advances in fluid resuscitation, improvements in wound coverage, better support of the hypermetabolic response to injury, advances in infection control practices, and improved treatment of inhalation injuries.3,6,7
Patients with burn injuries have special needs throughout the hospitalization. The ABA has established guidelines to determine which burn-injured patients should be transferred to a specialized burn center to maximize treatment effect and decrease patient morbidity and mortality rates (Box 32-1). Patients meeting the criteria outlined by the ABA should be transported to the nearest burn center.
BOX 32-1 American Burn Association Criteria for Burn Center Transfer and Referral
Patients meeting the following criteria should be transferred or admitted to a burn unit:
• Second-degree burns greater than 10% TBSA
• Full-thickness burns greater than 5% TBSA
• Any burn involving the face, hands, feet, eyes, ears, or perineum
• Any burn that may result in cosmetic or functional disability
• Circumferential burns of the chest or extremities
• Inhalation injury and associated trauma
• Electrical burns, including lightning injury
• Significant comorbid conditions (diabetes mellitus, chronic obstructive pulmonary disease, cardiac disease)
PATHOPHYSIOLOGIC FEATURES IF BURN INJURY
TISSUE INJURY
In a burn, tissue injury results from coagulation of cellular protein in response to heat produced by thermal, chemical, electrical, or radiation energy. The depth of coagulative necrosis of the tissue depends on the heat generated by the causative agent and the length of contact with the tissues (Figure 32-1).7 Thermal energy is the most common cause of burn injury. Common sources of thermal burns are flame, steam, scald, and contact with hot objects or surfaces. Inhalation injury is a type thermal injury caused by superheated gases that enter the airway when burn victims are injured in an enclosed space.
Electrical injury is produced by the conversion of electrical energy into heat and from the direct physicochemical effects of electrical current on tissue. The amount of current flowing in a circuit is directly proportional to voltage and inversely proportional to resistance. The quantity of heat produced by an electric current is related to the amount of voltage, the resistance of the conductor, and the duration of contact. Each type of tissue within the body absorbs the heat energy according to its own electrical resistance. Electrical current proceeds down the path of least resistance, which is through nerves, blood vessels, and muscles, sparing the skin except at the entry and exit points of the current.8 Thus, most injuries caused by this mechanism consist of internal deep tissue damage, impairing the ability to accurately determine the true extent of injury.
Regardless of the causative agent, the resulting tissue damage creates an injury pattern. Burn wounds can be conceptualized as having three zones (Figure 32-2) representing damage to the tissues resulting from transfer of heat. The central zone of coagulation is an area of irreversible tissue necrosis, or full-thickness burn. Immediately surrounding the necrotic zone is the zone of stasis, characterized by impaired blood flow.7,9 This zone may not show areas of coagulation initially but may progress to tissue necrosis if adequate tissue perfusion is not restored to the area during the resuscitation period. The zone of stasis is the area of greatest concern because this area can convert to a deeper wound, creating a larger burn injury and loss of tissue. The outer zone of hyperemia has sustained minimal tissue injury and usually heals rapidly.
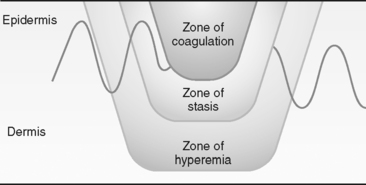
FIGURE 32-2 Zones of thermal injury. Zones of injury represent damage to tissue resulting from transfer of heat.
(From Wolf SE, Herndon DN: Burns. In Townsend CM, Beauchamp RD, Evers BM et al, editors: Sabiston textbook of surgery: the biological basis of modern surgical practice, 17th ed, Philadelphia, 2004, W. B. Saunders, p. 571.)
When a burn injury occurs, local mediators such as histamine, serotonin, kinins, arachidonic acid metabolites, xanthine oxidase products, complement, cytokines, and catecholamines are released by the body,6,7,10,11 resulting in arteriolar and venular dilation, increased microvascular permeability, and decreased perfusion. Subsequent extravasation of proteins from the intravascular space increases tissue oncotic pressure, creating edema.12 Increased levels of the profound vasoconstrictor thromboxane A2 are found in the plasma and wounds of burn patients. This compound may compromise perfusion, thus enlarging the zone of stasis and converting a partial-thickness wound to a full-thickness injury.7,10 Concurrently during tissue injury, the coagulation system is activated, causing platelet aggregation. Polymorphonuclear neutrophil (PMN) leukocytes and macrophages are present and release multiple cytokines that are essential to wound healing. The summation of the activation of the intense inflammatory response is vascular stasis and rapid formation of tissue edema.
EXTENT AND DEPTH OF INJURY
Two important concepts in the clinical diagnosis and management of burn injuries are the extent and depth of burn injury. The extent of burn refers to the total surface area of injured tissue. This is usually calculated as percentage of TBSA by use of either the Berkow,13 Lund-Browder,14 or rule-of-nines formula. With any of the burn calculation assessment tools, the clinician’s goal is to estimate the amount of tissue damaged by the exchange of thermal energy.
The Berkow and the Lund-Browder charts are more accurate assessment tools. They divide the body into multiple areas and take into consideration changes in the contribution of the head and legs from infancy to adulthood (Figure 32-3).
The rule of nines is easy to remember and provides a rapid, gross estimate of the extent of burn.15 With this method, the body is divided into seven areas that represent 9% or multiples of 9% of the body surface area, with the remaining area, the genitalia, representing 1% of TBSA (Figure 32-4).
The last method of determining the extent of TBSA injured uses the size of the patient’s hand, assuming that the palmar surface of the hand is roughly 1% of TBSA. Visualizing the patient’s hand covering the burn wound approximates the amount of body surface area involved.11
The concept of depth of burn injury is an important predictor of survival and overall morbidity, to include surgical management, functional outcome, and cosmesis. Descriptions of the depth of burn are often confusing because a variety of nomenclature is used (Table 32-1). In general the burn wound depth describes tissue damage according to the anatomic thickness of the skin involved, as determined by the clinician (Figure 32-5).7 As a general rule, the more superficial the burn wound, the more rapidly the wound heals. When a burn injury is assessed, skin layers are not visible to the naked eye. Thus, when the depth of a burn injury is determined, it is helpful to remember that the dermis is the layer that will bleed.16 Superficial and full-thickness burns do not bleed because the epidermis and subcutaneous layer are avascular. The greater the tissue loss (e.g., deep partial-thickness and full-thickness injury), the greater the likelihood that surgical grafting will be needed to optimize healing, limit infection, and maximize cosmesis.
TISSUE INJURY AND THE IMMUNOLOGIC RESPONSE
In the past decade, much knowledge has been amassed about the relationship of tissue injury and immunologic response to burn injury. Research into the histochemical response to tissue injury continues to elucidate the mechanisms of inflammation, infection, acute respiratory distress syndrome (ARDS), sepsis, systemic inflammatory response syndrome (SIRS), and multiple organ dysfunction syndrome (MODS).17,18 Tissue injury related to burns refers not only to the local response of the coagulation produced by heat but also to the local and systemic responses that lead to inflammation, immunocompromise, fluid shifts, and ultimately MODS if proper treatment is not provided. Certain risk factors, such as age 50 years or older, a full-thickness burn of 30% or greater TBSA, male sex, inhalation injury, and infection (sepsis), have an increased association with MODS in burn victims.18–21 In addition, severe compromise to the immune system occurs in burns of greater than 40% TBSA,11 overwhelming all cellular components of the immune system.7 Research continues to enhance the understanding of and treatments for local and systemic inflammatory processes that result in fluid shifts and altered tissue perfusion (burn shock) (Figure 32-6).
The pathophysiologic effects related to burn injury (greater than 20% TBSA)22 and acute inflammatory processes are both local and systemic. The microcirculation compromise is greatest 12 to 24 hours after a burn is sustained.6,21 The goals of therapy are to control the exaggerated inflammatory cytokine cascade response and restore the microcirculatory perfusion of tissues.17 Collectively, inflammatory mediators produce vasoconstriction, vasodilation, increased capillary permeability, activation of the coagulation cascade, and progressive and rapid edema formation with altered microcirculatory perfusion. These complex interactions of inflammatory mediators are tightly interwoven in both physiologic and pathophysiologic states. Mediators leave the confines of the local tissue injury and move to the systemic circulation, causing alterations in organ function remote from the site of injury.17 In large burn injuries, cytokine activities appear to create a state of exaggerated or reactivated inflammation, causing distant organ involvement (e.g., ARDS, SIRS, and eventually MODS).17
In summary, the exaggerated activation of the inflammatory cascades produces microvasculature-induced cardiopulmonary changes marked by loss of plasma volume, increased peripheral vascular resistance, and subsequent decreased cardiac output. Renal blood flow is altered, resulting in renal dysfunction. Activation of the stress response causes mild respiratory alkalosis and hypoxemia, which is complicated by increased pulmonary capillary permeability, resulting in decreased pulmonary compliance and function. Metabolic changes are highlighted by an early depression followed by marked and sustained increases in resting energy expenditure, lipolysis, proteolysis, and oxygen consumption.22 Eventually, generalized impairment of host defenses, depression of immunoglobulins, and fatigued immune response make the burned patient prone to infections. Promising advances in the treatment of burn sepsis exist with use of venovenous continuous renal replacement therapy and continuous plasma filtration-coupled adsorption.21 These therapies may be effective in removing vasoactive circulating cytokines and endotoxins.21,23
METABOLIC RESPONSE TO TISSUE INJURY
The metabolic response to burn injury has been studied extensively. As early as 1930, Cuthbertson24 described the biphasic metabolic response to injury. He noted increased fluid shifts, urinary nitrogen losses, and losses of other intracellular substances such as potassium and phosphorus. In subsequent years the studies of the catabolic response to injury documented increased oxygen consumption, negative nitrogen balance, exaggerated hypermetabolism and catabolism, excessive muscle wasting, and weight loss in burned patients. The degree of catabolism that occurs with burn injury has been found to correlate with increasing morbidity and mortality rates25; thus, early interventions in treatment attempted to attenuate the hypermetabolic response associated with burn injury. The intensity of the metabolic changes in burn patients is directly related to the extent of injury.22,26 Metabolic demands increase by an estimated 30% in patients with an injury covering 20% of the TBSA or more and by 100% in patients with burns covering 50% of the TBSA or more.22 Other factors associated with burn management, such as extensive dressing changes, thermoregulation, pain, infection, presence of inhalation injury, and sepsis also significantly increase metabolic response and energy expenditure needs.22,25,26
The resting energy expenditure (REE) after a burn injury can be as much as 200% greater than normal,7 creating a hypermetabolic drive. Burn hypermetabolism is the result of multiple mechanisms associated with hormonal changes. After a burn injury, inflammatory mediators stimulate the hypothalamus, increasing the body’s central thermoregulatory set point and altering endocrine function. Levels of catecholamines, cortisol, and glucagon are markedly elevated, initiating the catabolic response seen in burn patients. The presence of catecholamines increases the rates of glycogenolysis and hepatic gluconeogenesis and promotes lipolysis and peripheral insulin resistance.27 The exaggerated metabolic response and excessive mobilization of glucose for energy requirements are needed for wound healing. When adequate glucose cannot be supplied, the result is excessive protein catabolism.22,26 Thus, early nutritional interventions, with particular emphasis on meeting the REE, cannot be overemphasized. Recent research indicates that β-blockade with an agent such as propranolol, which reduces tachycardia by 15% to 20%, eases the hypermetabolic state induced by a large burn injury. The REE is lowered and muscle catabolism is reduced, thus improving patient outcomes.7,20,25,27–29
The sympathetic response seems to be the major determinant of the metabolic response to burn injury. The sympathetic nervous system can be stimulated by a variety of responses, including lower-than-normal environmental temperatures, pain, psychologic responses, and the body’s response to inflammatory mediators. It would seem that the use of nutritional replacement formulas based only on the size of the burn injury may not be accurate. Caution must be used in the application of standard nutritional replacement formulas; nutritional goals and end points should be reassessed and adjusted more often as the burn wound heals. Chapter 17 provides a thorough discussion of nutritional assessment parameters and formulas that may be used to guide nutritional interventions for burn-injured patients.
Optimally, nutritional replacement therapy in the burn patient should be based on frequent measurements of oxygen consumption obtained with the use of a metabolic cart. (See Chapter 17 for a discussion of the advantages and disadvantages of metabolic carts.) Nutritional formulas7,22 vary as much as resuscitation formulas, and there is little consensus as to which is most appropriate. In part, this lack of consensus has to do with the great variability in nutritional needs from patient to patient and for the same patient over time. This is why actual measurement of oxygen consumption allows more accurate nutritional repletion.22
MANAGEMENT OF THE PATIENT WITH A BURN INJURY
PHYSIOLOGICAL RESPONSE: CHANGES IN HEMODYNAMICS
The body’s initial response to a burn injury leads to a shift in fluids from the vascular space into the interstitial and intracellular spaces. When the burn involves large areas of skin (e.g., more than 20% TBSA), the hemodynamic and inflammatory response is an overall systemic response, with fluids shifting into interstitial spaces throughout the body. This massive fluid shift may lead to hypovolemic shock. To prevent shock, fluid resuscitation after burn injury is maintained for 24 to 48 hours after injury.30,31 The challenge during fluid resuscitation is to restore adequate circulating volume that prevents complications from underperfusion of organs and burned tissue without overresuscitating and creating problems from excessive volume replacement.
A number of formulas have been suggested for optimal fluid replacement after a burn injury. No formula is precisely prescriptive in fluid resuscitation, but the formulas provide guidelines for fluid replacement on the basis of TBSA burned and end-organ assessment parameters. All formulas calculate the amount of fluid replacement from the time of the burn injury and estimated TBSA burn injury size. Some of the early formulas, such as the Evans formula and the original Brooke formula, recommended a mixture of sodiumcontaining fluids and colloid because both sodium-rich fluids and plasma proteins are lost during fluid shifts with burn injury. The use of colloids is controversial. The SAFE (Saline versus Albumin Fluid Evaluation) study found no significant differences between groups of patients treated with colloid or normal saline solution for fluid resuscitation.32 In burninjured patients, the use of colloids in the first 12 hours has been associated with less favorable outcomes; therefore, albumin administration is not recommended until at least 12 hours after the burn.21 The Baxter (Parkland) formula supports the concept that plasma given in the first 24 hours is no more effective than Ringer’s lactate solution alone in maintaining normal plasma volume. The Monafo and Warden formulas focus on the administration of hypertonic saline solution as a means of limiting edema and they require a lower total amount of fluids than do other formulas. Caution is warranted in the administration of hypertonic solutions because the concentrations of hypertonic solutions are not standardized and have been associated with an increased incidence of morbidity and poor outcomes. Hypertonic solutions should be used by experienced providers to avoid complications associated with administration of these concentrated fluids.21 The Demling formula incorporates the use of low-molecular-weight dextran in resuscitation formulas, with the goal of preventing edema in nonburned tissues. The consensus formula33 combines concepts, using crystalloid and colloid administration at various times in the fluid resuscitation process. Table 32-2 outlines the available formulas for calculating the fluid resuscitation requirements for burned patients. No matter what formula is used, it must be viewed only as a guideline.21,31 Fluids should be carefully titrated by 20% increments34 to physiologic end points,21 and overresuscitation should be avoided.
TABLE 32-2 Fluid Resuscitation Formulas
Baxter (Parkland) formula | |
Consensus formula | Adults: Lactated Ringer’s solution (2-4 ml/kg/% TBSA) Children: Lactated Ringer’s solution(3-4 ml/kg/% TBSA), half given over first 8 hours; remaining half given over next 16 hours Adults: Colloid-containing fluid (0.3-0.5 ml/kg/% TBSA) and electrolyte-free fluid to maintain adequate urine output Children: Colloid-containing fluid (0.3-0.5 ml/kg/% TBSA) and half normal saline solution to maintain adequate urine output |
Modified Brooke formula | |
Monafo formula (hypertonic saline solution) | |
Modified Warden formula (hypertonic saline solution) for burns <40% TBSA | 180 mEq/L Na+ (mix 50 mEq/L NaHCO3 in lactated Ringer’s solution), 4 ml/kg/% TBSA; adjust to maintain urine output of 30-50 ml/hr Lactated Ringer’s solution at rate to maintain urine output of 30-50 ml/hr |
Dextran (Demling) formula |
Kidney function is monitored by measuring urine output, urine specific gravity, the urine glucose level, and the urine electrolyte content. Hourly urine volume from 0.5 to 1 ml/kg is often cited as reflecting effective renal perfusion; however, this urine output range has not been supported by clinical trials. To date, clinical trials have not revealed ideal urine output measurements for evaluating renal and other end-organ perfusion during burn shock resuscitation.21,35 In evaluating urine output as evidence of adequate perfusion, the practitioner should keep in mind that diuresis can occur as a result of glucosuria in response to stress or as a response to hypertonic saline solution or dextran administration during resuscitation; therefore, additional end point parameters should be used to validate the adequacy of the fluid resuscitation.21
Because of the early release of catecholamines and venoconstriction during burn shock states, blood pressure may be artificially elevated in relation to the degree of hypovolemia.21 Additionally, noninvasive blood pressure measurements may be inaccurate because of significant edema formation in the interstitium. Thus, trends obtained from frequent monitoring of blood pressure may not reflect the status of resuscitation. Pulse pressure may be a more accurate assessment of tissue perfusion pressures. Pulse pressure is easy to measure and correlates well with stroke volume. It is obtained by subtracting diastolic pressure from systolic pressure. Narrowing or decreased pulse pressure (less than 25 mm Hg) is an earlier indicator of shock than is a decline in systolic blood pressure.21
Frequent monitoring of the heart rate may be useful in assessing and monitoring the cardiovascular response to resuscitation. The heart rate is elevated during the initial phase of burn injury because of catecholamine release. Changes in heart rate, or decreases in heart rate, can be used to gauge effective management of resuscitation.20 In an elderly patient or a patient with cardiac disease, however, the heart rate may not increase as the patient becomes hypovolemic; thus, heart rate is a less reliable measure of resuscitation in these patients, and trends in increased heart rates need to be evaluated.
Other cardiovascular parameters that may be monitored include central venous pressure, pulmonary artery wedge pressure (PAWP), mixed or central venous oxygen saturation,36 and cardiac output. Filling pressures are often very low for the first 24 hours after a burn, and any efforts to improve filling pressures during this period may result in overresuscitation. As long as other measurements of tissue perfusion are within normal ranges, the temptation to improve filling pressures should be resisted.21 Likewise, cardiac outputs are often very low in the first 24 hours and then trend upward over the second and third 24 hours until they are one and a half to two times normal. This is thought to result from the hypermetabolic response observed in patients with larger burns. Thus, the use of invasive monitoring devices, such as pulmonary artery (PA) catheters for measurement of PAWP and cardiac output, adds little to the ability to monitor resuscitation and may increase long-term morbidity because of the increased risk of infectious complications. However, in the elderly patient, the cardiac patient, or the patient with severe inhalation injury, the use of a PA catheter or monitoring of central venous pressures to guide fluid resuscitation is advised.6,21 Controversy continues to surround the concept of hyperdynamic resuscitation guided by oxygen transport and consumption parameters, which is not a current standard of practice.21
Acid-base balance is another indicator of the effectiveness of fluid resuscitation. A base deficit and elevated serum lactate levels occur in hypoxic conditions and states of poor tissue perfusion. Base deficit manifests as metabolic acidosis and corrects to normal with adequate resuscitation. A base deficit of 6 mEq/L or greater is an indicator of shock at 24 hours after the burn, and elevated serum lactate levels have been associated with increased mortality rates.18,21,30 The base deficit may remain elevated in the presence of adequate urine output and mean arterial pressures. Thus, base deficit and serum lactate have been found to be better markers of effective resuscitation.21,30
Fluid requirements are increased when patients also have inhalation injury, require mechanical ventilation, have increased full-thickness injury, have electrical burn injury, experience a delay in resuscitation, and are dehydrated.31,34 Maximizing fluid resuscitation to improve tissue perfusion without overresuscitating remains the challenge. Complications of significantly increased volume resuscitation include compartment syndrome of the extremities or abdomen. Patients with circumferential deep and full-thickness wounds are at the greatest risk for development of compartment pressure complications. Compartment syndrome may be an early problem because fluid shifts and maximal edema occur during fluid resuscitation. Neurovascular assessment and direct compartment tissue pressures should be assessed in patients with circumferential extremity burns. Tissue compartment pressures greater than 20 mm Hg indicate compromised perfusion and pressures greater than 40 mm Hg suggest significantly compromised perfusion. In these cases, escharotomy, fasciotomy, or both are usually needed to release the pressure and expand the tissue compartment. Abdominal compartment syndrome (ACS) has been associated with a mortality rate of 60% to 100%. Although the incidence of ACS is not well described in the burn literature, it may be associated with concurrent blast injury and gross fluid overresuscitation.37,38 Estimated fluid resuscitation requirements should be recalculated 12 hours after the burn injury and, if the volume calculated for fluid replacement exceeds 6 ml/kg/% TBSA, interventions to minimize complications of overresuscitation should be implemented. Some interventions that may be considered are as follow:
• Initiate 5% albumin described in the Modified Brooke Formula.
• Monitor bladder pressures every 4 hours for increased abdominal pressure greater than 20 mm Hg.
• Monitor central venous pressures, pulmonary artery pressures, and cardiac output to trend effectiveness of resuscitative efforts.
NUTRITIONAL MANAGEMENT
The nutritional needs of most burn patients can be met with a mixture of glucose, essential amino acids, and fats. Glucose is the primary source of energy for wound healing, and supplementation with lipids prevents severe hyperglycemia.39 In addition to the major nutrients, vitamins and minerals are important in wound healing. As mentioned previously, multiple formulas exist for estimating the nutritional needs of the burned patient. Hart et al40 have found that increasing caloric requirements relative to energy expenditure does not preserve lean body mass and that overfeeding is associated with increased fat mass, increased need for ventilatory support, and lengthened intensive care unit stay. These researchers recommend nutritional intake of no more than 1.4 × REE kcal/day to prevent catabolism.40 Regardless of which formula is used, several principles need to be followed:
• Nutritional assessment and reassessment must be frequent and continual throughout the course of the healing process.
• Enteral tube feedings should begin within 24 hours after the burn to decrease production of catabolic hormones, improve nitrogen balance, and maintain gut mucosal integrity.11,39
• Increased protein consumption is critical to survival and rehabilitation of the burned patient.39
• Anabolic hormone (e.g., oxandrolone) administration should be considered to attenuate muscle wasting and improve net protein balance.20,41
As a part of nutritional management, electrolyte balances should be evaluated and imbalances corrected. Frequent measurement of serum sodium, potassium, chloride, phosphorus, calcium, and magnesium levels is necessary to prevent major electrolyte derangements. In addition, deficiencies in trace metals may occur in the burn patient; thus it is recommended that zinc, copper, manganese, and chromium levels be measured periodically and replaced if found deficient. Zinc is an important cofactor in wound healing and tends to be deficient after burn injury. Glutamine is a free amino acid that provides fuel for lymphocytes, macrophages, and fibroblasts. Glutamine supplementation has been shown to reduce the incidence of gram-negative bacteremia in severely burned patients42 and to minimize the loss of lean muscle mass in burn-injured patients.22 Nutritional support teams should be used to evaluate the continuing and changing nutritional needs of the burn injured patient. All efforts to maximize nutritional status of the burn patient are necessary for successful recovery, wound healing, and rehabilitation. See Chapter 17 for further discussions on nutritional interventions.
MANAGEMENT OF PULMONARY INJURY
Pulmonary injury may result from inhaling the byproducts of smoke or from a systemic process related to SIRS or MODS. Inhalation injury may or may not injure lung tissue directly. One possible component of inhalation injury is carbon monoxide intoxication. Carbon monoxide does not affect the lining of the lung but competes with oxygen for uptake by hemoglobin, thus acting as an asphyxiant. Because hemoglobin has 200 times more affinity for carbon monoxide than for oxygen, carbon monoxide replaces oxygen, reducing oxygen delivery to tissues. This may lead to severe anoxia and brain injury. In addition, carbon monoxide combines with myoglobin in muscle cells and the cytochrome oxidase system of the brain, producing muscle weakness and coma, respectively. The initial effects of muscle weakness and confusion from decreased oxygen uptake occur within about 5 minutes of exposure and may contribute to the person’s inability to escape from the fire. The long-term neurologic effects occasionally associated with smoke inhalation are most likely related to both prolonged anoxia and inhibition of the cytochrome oxidase system of the brain. A carboxyhemoglobin (COHb) level above 20% at the time of exposure will cause neurologic effects; a level above 60% may be fatal.43 Carbon monoxide has a half-life of 4 hours if the patient breathes room air and 1 hour if the patient is breathing 100% oxygen. COHb levels measured in the emergency department must be interpreted in relation to the time after exposure and the concentration of oxygen administered to the patient since the exposure.43 Thus, a person with 25% COHb after breathing 100% oxygen for 1 hour probably had a level of about 50% COHb at the time of exposure. Treatment for suspected COHb exposure is 100% oxygen until measured serum levels of COHb are less than 10%.44 In addition, caution must be exercised with the use of pulse oximetry equipment as an evaluative tool for oxygen saturation. Pulse oximetry is inaccurate in the presence of elevated COHb.
Other components of inhalation injury are upper airway injury and chemical injury to the lung parenchyma. Upper airway injury is the result of inhaling superheated air, which may cause blisters and edema in the supraglottic area around the vocal cords. This may cause upper airway occlusion and is best treated with early endotracheal intubation. Tracheobronchial and parenchymal lung injuries are caused by the inhalation of a variety of chemicals (e.g., oxides of sulfur and nitrogen, aldehydes, and acrolein) that are byproducts of combustion. Although the exact mechanics of injury may differ, thermal and chemical insult to the major airways and lung parenchyma cause the ciliated epithelial cells to separate from the basement membrane and the systemic and bronchial circulation of the lung to dilate, with protein and water shifts increasing interstitial edema.7,45 The tracheobronchial casts formed by the deposition of proteins and fibrin in the airways are difficult to remove with standard suction techniques, requiring bronchoscopic lavage for removal.7 The loss of the mucociliary system results in ineffective bacterial clearing and predisposes the patient to pulmonary infections and sepsis.46 Thus a patient with mild inhalation injury who maintains a strong cough reflex should be extubated as soon as possible to facilitate clearing the airway and reduce the risk of nosocomial pneumonia.7,46 Damage to type II pneumocytes44,45 decreases surfactant production, causing the development of microatelectasis, increased airway pressures, and ventilation-perfusion mismatches. Hemorrhagic tracheobronchitis, increased interstitial edema, and decreased macrophage function are additional sequelae seen with inhalation injury. Sloughing of the airway mucosa and intrapulmonary hemorrhage causes mechanical obstruction that can be removed with inhaled bronchodilators and mechanical bronchoscopic clearing.44,47 Treatment with aerosolized heparin alone or with N-acetylcysteine may facilitate removal of the copious secretions associated with inhalation injury. The addition of β-blockers attenuates the bronchoconstriction associated with the injury and use of aerosolized heparin.7,43,47,48 Management efforts are focused on limiting the inflammatory response and fluid sequestration in an effort to avoid the complications of ARDS and pneumonia.
As mentioned, most of the 6% of all burned patients who do not survive have had an inhalation injury. Diagnosis of inhalation injury is based on the patient’s presentation (Box 32-2), history of injury, and the findings on fiberoptic bronchoscopy or, if available, a xenon 133 ventilationperfusion scan. A chest radiograph is helpful in establishing baseline data but typically does not assist in the early diagnosis of inhalation injury.
BOX 32-2 Characteristics Suggesting Inhalation Injury
• Burn injury occurred in a closed space
• Edema and redness of the oropharynx/nasopharynx
• Tachypnea, wheezing, stridor
• Carbonaceous sputum, singed nasal and facial hair
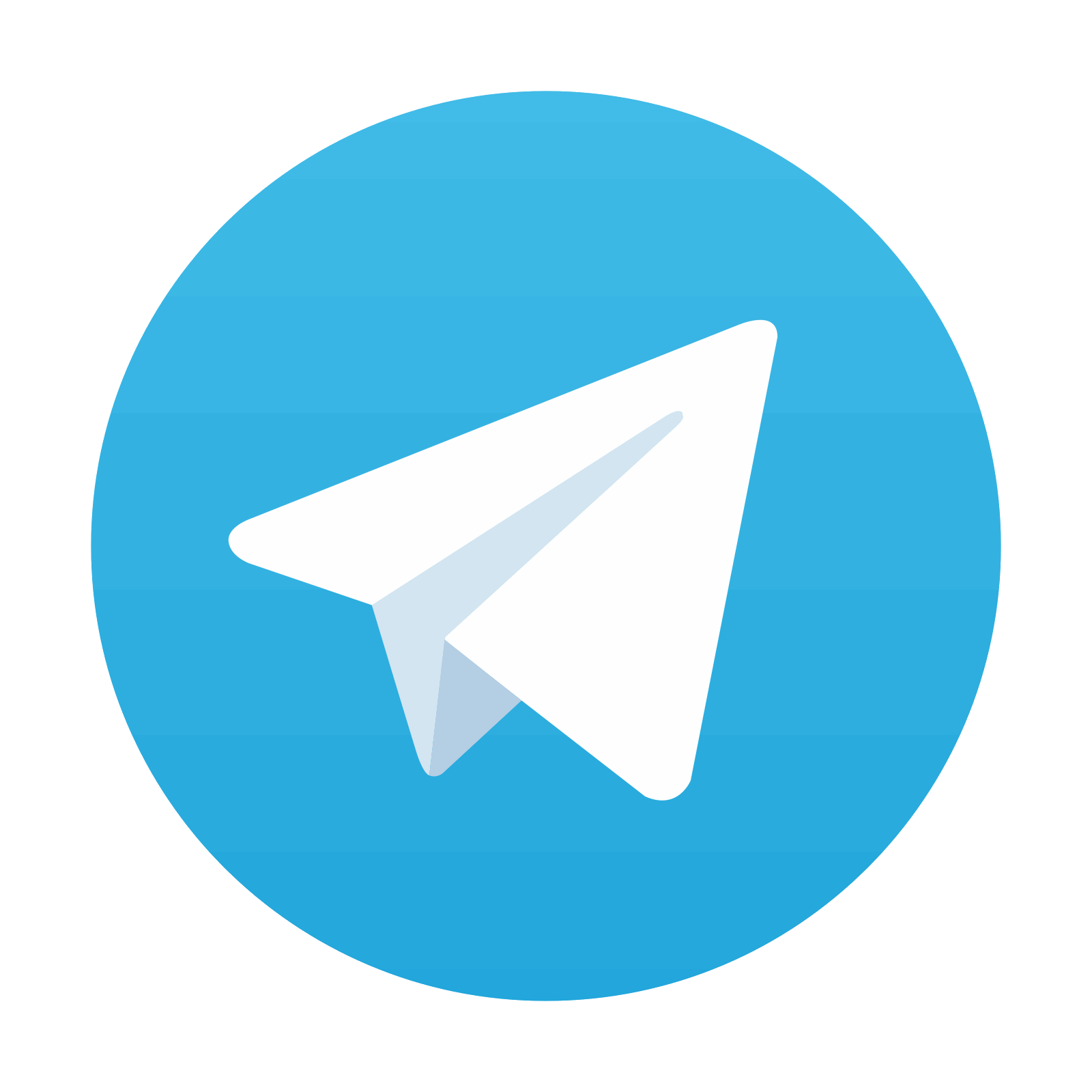
Stay updated, free articles. Join our Telegram channel

Full access? Get Clinical Tree
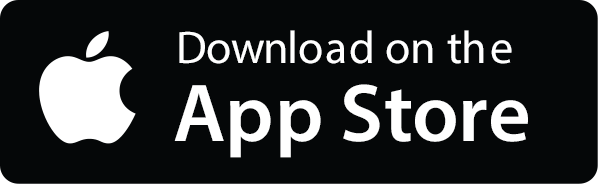
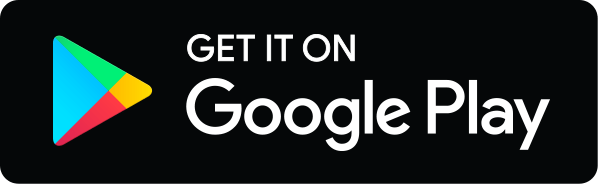