Spasticity and Paralytic Disorders
R. David Warren
Spasticity was defined by Lance as a motion disorder characterized by a velocity-dependent increase in tonic stretch reflexes (muscle tone) with exaggerated tendon jerks resulting from hyperexcitability of the stretch reflex as one component of the upper motor neuron syndrome (1). This chapter attempts to give some insight into the causes of spasticity. Even though spasticity affects the upper extremities as well as the lower extremities, the focus is on how it affects the lower extremity with regard to diagnosis, and rationale for establishing a treatment plan, either conservative (therapy, pharmacologic) or surgical.
In reality, spasticity is difficult to define comprehensively presumably because the neurobiology remains largely a mystery (2). In fact, Young (2) states that although spasticity is difficult to define, neurologists recognize spasticity when they see it—at least they think they do. Spasticity as a term is widely used by clinicians to describe abnormalities in patients with lesions of the central nervous system, but the signs and symptoms found in this group vary widely from patient to patient as does the response to any given therapy (3). What is frequently forgotten is that spasticity is but one component of the upper motor neuron syndrome and many confuse the other findings of upper motor neuron syndrome and use them interchangeably with spasticity (4).
Upper motor neuron syndrome is characterized by impaired motor control, muscle paresis, and muscle spasticity. This net imbalance of muscle forces across joints can lead to both dynamic and static joint deformities (5). Some authors believe spasticity is such a strongly integrated part of upper motor neuron syndrome that it cannot be separated from the other components (6).
Upper motor neuron syndrome is the result of damage to the descending motor pathways at the cortical, brainstem, or spinal cord level (7). Some of the more common causes are stroke, cerebral palsy, spinal cord injury, multiple sclerosis, and traumatic brain injury. This results in both positive and negative findings. The positive findings are athetosis, clonus, dystonia, primitive reflexes, rigidity, and spasticity (4). This is the result of the release of a more or less intact motor subsystem for precise rostral control (2). The negative findings are fatigue, impaired coordination, impaired motor control, impaired motor planning, and muscle weakness (4). This is thought to be a direct result of disconnecting lower motor centers from higher motor centers (2). It is difficult, if not inadequate, to discuss the single positive sign of spasticity in the absence of addressing its overlap of similarities with other findings of upper motor neuron syndrome (4).
To adequately discuss upper motor neuron syndrome, a consensus on terminology needs to be achieved. An interdisciplinary workshop sponsored by the National Institutes of Health in 2001 defined the terms spasticity, dystonia, and rigidity (8). Spasticity was defined as hypertonia with one or both of the following present:
Resistance to externally imposed movement that increases with increasing speed of stretch and varies with the direction of joint movement
Resistance to externally imposed movement increases above a threshold speed or joint angle (8)
Dystonia is defined as a movement disorder in which “involuntary sustained or intermittent muscle contractions cause twisting and repetitive movements, abnormal postures, or both” (8). And finally, rigidity is defined as hypertonia with all the following present:
Resistance to externally imposed joint movement is present at very low speeds of movement, does not depend on imposed speed, and does not exhibit a speed or angle threshold.
Simultaneous cocontraction of agonists and antagonists may occur and is reflected in an immediate resistance to a reversal of the direction of movement about a joint.
The limb does not tend to return to a particular fixed posture or extreme joint angle.
Voluntary activity in distant muscle groups does not lead to involuntary movements about the rigid joints, although the rigidity may worsen (8).
To confuse the picture, in clinical practice these terms are often used interchangeably.
PATHOPHYSIOLOGY
The basic neurophysiologic mechanism(s) that produce spasticity remain largely unidentified or not yet clearly identified (2,9,10). Spasticity is one of several abnormalities of muscle tone, resulting from a lesion anywhere along the corticospinal (pyramidal) tracts (4). Because of their size, most lesions of the central nervous system damage structures other than the corticospinal pathway: They also usually affect various extrapyramidal structures in the spinal cord, brainstem, basal ganglia, or cortex. Practically every patient with the upper motor neuron syndrome has a combination of spasticity due to damage to the
corticospinal (pyramidal) system and rigidity (also defined as dystonia) due to lesions affecting the extrapyramidal system (3). Depending on the site and extent of the central nervous system damage, a number of manifestations of spasticity occur. They include the Babinski response, a velocity-dependent increase in tonic stretch reflexes (increased “muscle tone”), exaggerated phasic stretch reflexes (increased “tendon jerks”), hyperactive cutaneous reflexes (“flexor spasms”), increased autonomic reflexes (“mass reflex”), and abnormal posture (“dystonia”) (11).
corticospinal (pyramidal) system and rigidity (also defined as dystonia) due to lesions affecting the extrapyramidal system (3). Depending on the site and extent of the central nervous system damage, a number of manifestations of spasticity occur. They include the Babinski response, a velocity-dependent increase in tonic stretch reflexes (increased “muscle tone”), exaggerated phasic stretch reflexes (increased “tendon jerks”), hyperactive cutaneous reflexes (“flexor spasms”), increased autonomic reflexes (“mass reflex”), and abnormal posture (“dystonia”) (11).
Some of the proposed mechanisms of spasticity include fusimotor hyperactivity, hyperexcitable motor neurons, abnormal excitability of the spinal segmental and intersegmental interneurons from loss of supraspinal influences (inhibitory and excitatory), and changes in the muscles themselves (12).
Many of the concepts about the pathophysiologic nature of spasticity were based on deductions drawn from experiments on decerebrate cats because some of the phenomena observed superficially resembled those seen in patients with spasticity (13). Because of these observations, hyperactivity of the fusimotor system has been regarded as the major cause of increased muscle tone seen in human spasticity (14). More recent studies failed to support this theory. In fact, microneurographic studies (recordings of single muscle spindle afferents) have failed to produce evidence of overactivity of the afferent spindle discharges or of the fusimotor system in patients with spasticity (15,16 and 17). The burden of proof is therefore on anyone who continues to suggest that spastic hyperreflexia is due to fusimotor hyperactivity (3).
Hyperexcitability of motor neurons themselves is another proposed mechanism of spasticity. It is postulated that spinal cord transection changes the excitability of motor neurons (13). This seems to be reinforced by enhanced H/M ratios (18) and F-wave amplitudes (19). Hyperexcitability of the motor neurons themselves, the result of either a primary change in the membrane properties or to change in membrane potential secondary to alterations of synaptic input, may account for some aspects of spasticity (2). Substantial alterations in the mechanisms that control transmitter release from the presynaptic terminals of primary afferent fibers have been demonstrated. For example, in patients with spasticity there is evidence of a change in the excitability of, and release of transmitter from, afferent terminals (presynaptic inhibition) (13). This results in presynaptic inhibition affecting the afferent fibers from muscle spindles that appears inadequate (20). Reduction in the descending inhibition as a result of the upper motor neuron lesion may also result in changes in the numbers and kinds of synaptic endings on motor neurons (2). Once again, however, the information available from animal experiments is conflicting (21).
Early works of Sherrington and others revealed that the exaggerated motor response of the spastic patient originates from changes in the way in which segmental spinal cord circuitry handles information from a variety of sources, including proprioceptive, exteroceptive, and suprasegmental descending input (22). A principal current hypothesis states that spasticity is caused by long-term reductions in inhibition rather than permanent increases in excitation per se, but the differences between these alternatives are not always operationally clear and they need not be mutually exclusive (2). Among the types of inhibition currently recognized are recurrent Renshaw inhibition, reciprocal Ia inhibition, presynaptic inhibition of Ia terminals, nonreciprocal Ib inhibition, and inhibition of group II afferents (2).
Renshaw cells are small inhibitory interneurons in the anterior horns of the spinal cord that act to form a negative feedback mechanism in the motor neuron pool that stimulated them in the first place. They are controlled by activity descending from supraspinal areas. Hence, a lesion higher in the central nervous system might reduce their tonic background excitation, thereby decreasing their inhibitory activity (3). In studies by Katz and Pierrot-Deseilligny, they did not find that changes in recurrent inhibition were responsible for the hyperexcitability of the stretch reflex, which characterizes human spasticity. They did, however, find that paralysis of the supraspinal control of Renshaw cells, which normally accompanies voluntary movements, could account in part for two difficulties exhibited by spastic patients: in grading the strength of a muscular contraction and in regulating reciprocal Ia inhibition according to the requirements of voluntary movement (23).
Reciprocal Ia inhibition is a complex mechanism to inactivate the antagonistic muscle then the agonist contracts. There are Ia inhibitory interneurons in the gray matter of the spinal cord. They are excited by descending pathways from descending supraspinal pathways as well as by Ia input from the muscle spindles in the contracting muscle. These interneurons then send their axons to antagonistic motor neuron pools where they inhibit both the spinal motor neurons and Ia interneurons belonging to the antagonistic motor neuron pool (3). During normal human movement, several mechanisms contribute to increased transmission in Ia interneurons, which mediate reciprocal inhibition. First is activity mediated by descending supraspinal influences that begins even before the onset of contraction. Next is the powerful Ia discharge coming back from the contracting muscle. Third are descending supraspinal influences that decrease activity in Renshaw cells, reducing their inhibition of inhibitory interneurons (3). In the spastic patient, some or all of these mechanisms may be deficient (23).
Presynaptic Ia inhibition is the result of the release of gammaaminobutyric acid from inhibitory axons that then act on the receptor sites of the Ia terminal. These inhibitory interneurons that release gamma-aminobutyric acid are driven in part by impulses descending through the spinal cord. Suprasegmental lesions therefore reduce presynaptic inhibition by reducing descending excitation of those inhibitory interneurons (3).
Ib fibers are large myelinated afferent fibers from Golgi tendon organs that are in series with muscle fibers and are activated by contraction of the muscle. Impulses from the Ib fibers impinge upon homonymous and synergistic motor neurons after passing through short inhibitory interneurons. As with presynaptic inhibition, a supraspinal lesion may interfere with descending excitation of Ib inhibitory interneurons, which would reduce Ib inhibition and favor excitation of the stretch reflex. This remains hypothetical (3).
Group II medium-diameter myelinated fibers arise from endings of intrafusal fibers within muscle spindles. In animal models, activity of Group II fibers tends to excite flexor motor neurons and inhibit extensor motor neurons. If the discharge in spastic patients was reduced, there would be less inhibition of extensor motor neurons and therefore a greater tendency toward stretch reflexes in those muscles. This remains theoretical from the work in animals and has yet to be conclusively proven in humans (3).
Changes in the muscles themselves become apparent after spasticity has been present for a period of time. As spasticity becomes more established, another factor begins to play a role
in hypertonia (24). It has been suggested that in the case of hypertonia in the leg muscles, changes occur in the mechanical properties of spastic muscle (25). Other studies have shown that changes occur in tendon compliance and the relationship of tendons to their respective muscle mass. Animal studies have demonstrated that a loss of sarcomeres occurs when a joint is maintained in flexion (26). In children with cerebral palsy, collagen deposition in the muscles affects movement and correlates with the severity of their clinical status (27).
in hypertonia (24). It has been suggested that in the case of hypertonia in the leg muscles, changes occur in the mechanical properties of spastic muscle (25). Other studies have shown that changes occur in tendon compliance and the relationship of tendons to their respective muscle mass. Animal studies have demonstrated that a loss of sarcomeres occurs when a joint is maintained in flexion (26). In children with cerebral palsy, collagen deposition in the muscles affects movement and correlates with the severity of their clinical status (27).
CONDITIONS WITH SPASTICITY AS A COMPONENT
This section gives a basic overview of the conditions or disease states that may have spasticity as a component in either the upper or lower extremity. It is not intended as an in-depth review of the condition or disease state, but rather these more commonly seen entities will be reviewed in relation to their propensity to produce spasticity in the lower extremity as a sequela. These include stroke (cerebrovascular accident), cerebral palsy, spinal cord injury, multiple sclerosis, and traumatic brain injury.
STROKE (CEREBROVASCULAR ACCIDENT)
Stroke is defined as a neurologic deficit lasting more than 24 hours caused by reduced blood flow in a particular artery supplying the brain. It is characterized by a sudden onset of clinical signs related to the site in the brain where the morbid process occurs (28). The most common artery affected is the middle cerebral artery. This results in significant damage to the cerebral cortex. Damage to the pyramidal tract and its accompanying pyramidal (corticoreticulospinal) fibers gives rise to the upper motor neuron syndrome (29).
In the classic stroke involving the middle cerebral artery, the affected limbs are completely paralyzed, with loss of tendon reflexes (30). Initially, some 80% of stroke patients experience motor impairments of the contralateral limb(s) (29,31). The longer the limb remains flaccid, the poorer the prognosis for recovery (30). In most cases, the first changes noted in the flaccid limb are hyperactive tendon reflexes and increased resistance to passive movement, that is, spasticity. Spasticity is most pronounced distally but progresses to involve all muscles and, in the case of the lower extremity, most prominently the extensors and adductors (30). Spasticity after a stroke has been shown to peak at 1 to 3 months, with some patients who were not initially spastic now spastic and some who were initially spastic now with normal muscle tone (32). One study reported that 39% of patients with first ever stroke were spastic after 12 months (33). In general, neurologic return tends to be complete within 6 months of injury (30).
When movement begins to return, it does so with synergistic patterns. Return of voluntary lower extremity movement begins proximally with hip flexion and proceeds distally. Flexor synergies are the first to develop and are usually followed by, or overlap with, extension synergies. As voluntary movement increases, spasticity decreases in a proximal-to-distal distribution with increase of individual muscle group movement independent of synergistic patterns and gradual return of individual muscle speed, coordination, and endurance. While recovery proceeds in an orderly and sometimes overlapping manner, it may stop permanently at any stage (30).
Lower extremity spasticity following a stroke takes on certain patterns. The pelvis is elevated and the hip is extended, adducted, and internally rotated with the knee extended and the ankle in plantar flexion (30,33). If the patient has no functionally useful voluntary limb movement, spasticity can maintain an abnormal resting limb posture leading to contracture formation.
CEREBRAL PALSY
Cerebral palsy has been defined as a nonprogressive condition affecting those parts of the brain that control movements and postures. It is clear, therefore, that the term cerebral palsy does not refer to a single disease entity but rather represents a combination of motor disorders, sensory deficits, and mental impairment, varying from mild to severe, caused by injury to the central nervous system in the perinatal period (34).
The incidence of cerebral palsy is said to be 4.2 per 1,000 live-born infants according to the large collaborative perinatal study published in the United States in 1978 (35). The prevalence of moderately severe to severe cerebral palsy is from 1.5 to 2.5 per 1,000 live births (36). More than 100,000 Americans under the age of 18 years are estimated to have some degree of neurologic disability attributed to cerebral palsy (37). Contrary to initial expectations, with improvements in perinatal medicine including the use of fetal monitoring and cesarean section, the prevalence of cerebral palsy has not decreased (38). At present, there seems to be little we can do to reduce the prevalence cerebral palsy (39).
Cerebral palsy is classified based on topographical as well as neuromuscular deficit. The topographical breakdown is diplegia (30% to 40%), being the most common, with hemiplegia (20% to 30%) and quadriplegia (10% to 15%) comprising the bulk of the remainder. Monoplegia and triplegia are relatively uncommon (40). Neuromuscular deficit classification is comprised of spastic, dyskinetic (inclusive of choreoathetoid and dystonic), ataxic, hypotonic, and mixed. Spastic cerebral palsy is far and away the most common and accounts for 70% to 75% of all cases, with dyskinetic (10% to 15%) and ataxic (<5%) accounting for the remainder (40).
Pathophysiology
Two kinds of lesions are felt to be responsible for the bulk of cerebral palsy cases. The first type is hemorrhagic, that is, subependymal and intraventricular hemorrhages with possible parenchymal extension or development of hemorrhagic hydrocephalus (41). They are most common in premature infants of less than 32 weeks gestation (35). They originate in the thalamostriate groove at the level of the head of the caudate nucleus or slightly posterior to the foramen of Monro (41). The second type is hypoxic-ischemic, that is, periventricular leukomalacia with and without cyst formation (35). Periventricular leukomalacia is found in border zones between penetrating branches of the major cerebral arteries, most commonly at the trigone of the lateral ventricles or around the foramen of Monro, and often occurs in conjunction with hemorrhagic lesions (41). Since the pyramidal tracts to the lower extremities traverse the internal capsule closest to the lateral ventricles, both lesions can lead to the clinical picture of spastic diplegia, but periventricular leukomalacia is more commonly found in spastic quadriparesis and is generally
associated with more severe handicap (35). It has been estimated that the cause of cerebral palsy occurred 38.5% of the time in the prenatal period, 46.3% in the natal period, and 15.2% postnatal (42). Premature birth was the most common natal cause, with meningoencephalitis, trauma, and occlusion of a cerebral artery or vein as some more common causes postnatally (36).
associated with more severe handicap (35). It has been estimated that the cause of cerebral palsy occurred 38.5% of the time in the prenatal period, 46.3% in the natal period, and 15.2% postnatal (42). Premature birth was the most common natal cause, with meningoencephalitis, trauma, and occlusion of a cerebral artery or vein as some more common causes postnatally (36).
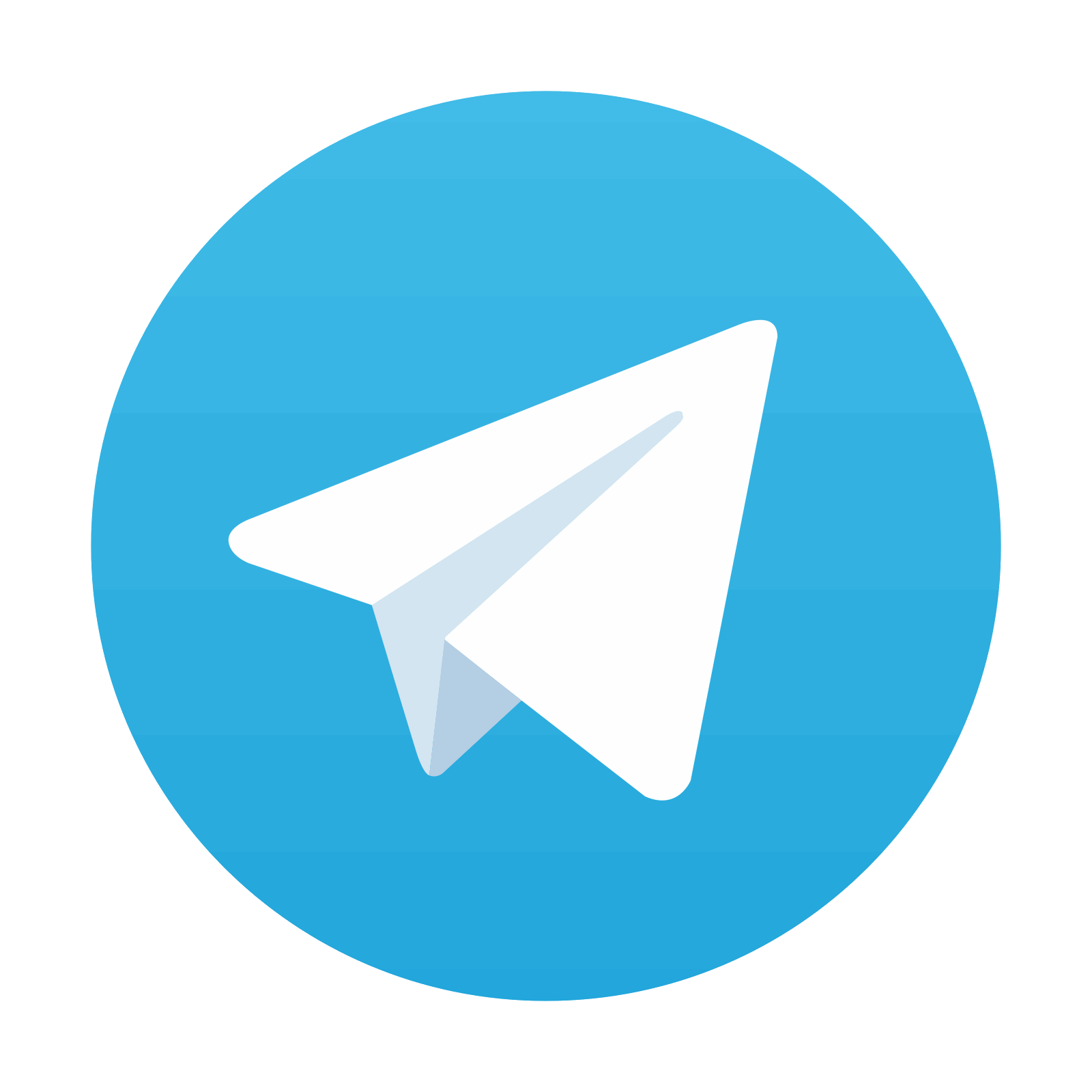
Stay updated, free articles. Join our Telegram channel

Full access? Get Clinical Tree
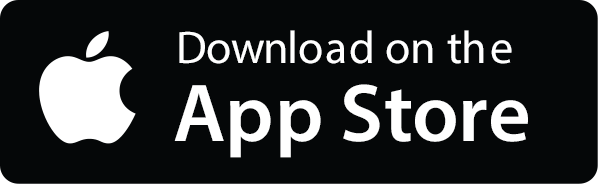
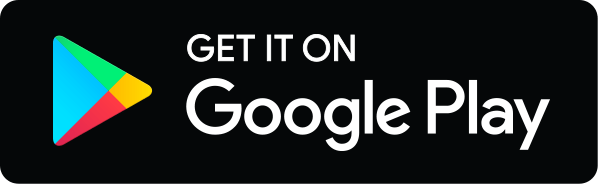
