Fig. 2.1
Evaluation of glenoid orientation using a sphere fit protocol in a three-dimensional CT-model of the scapula
In arthritic shoulders, specific changes to glenoid orientation and morphology can be seen. While in patients with primary osteoarthritis, overall retroversion appears to be slightly bigger compared to normal shoulders, significantly less retroversion is seen in patients with cuff tear arthropathy. Patients with cuff tear arthropathy and grade III or IV fatty infiltration of the infraspinatus or teres minor have an average of 3.6° glenoid retroversion, compared to 14.1° in patients with primary osteoarthritis and no significant fatty infiltration of rotator cuff muscles. On the other hand, patients with cuff tear arthropathy and grade III or IV fatty infiltration of the infraspinatus or teres minor have significantly less inclination as compared to patients with no significant fatty infiltration [14].
Walsh classified the specific changes of glenoid morphology in osteoarthritic shoulders. He differentiated three types: Group A represents a glenoid with central erosion, Group B shows posterior, excentric erosion due to subluxation of the humeral head, and Group C is a dysplastic glenoid with retroversion bigger 25°. Group B is further subdivided in group B1 where posterior sclerosis, joint space narrowing and osteophytes are present and group B2, where a biconcave aspect of the glenoid fossa is seen (Fig. 2.2) [15].
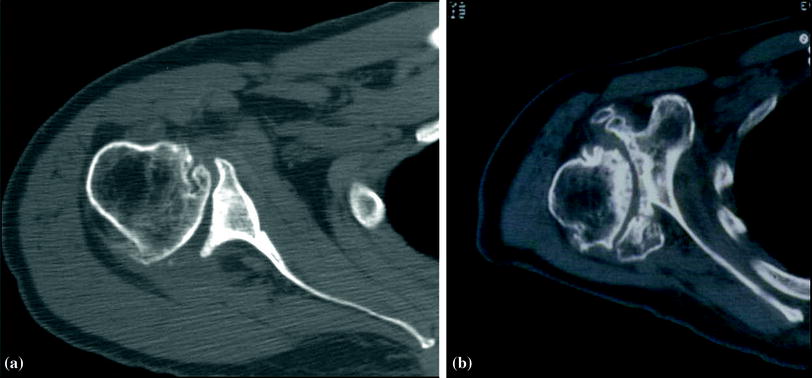
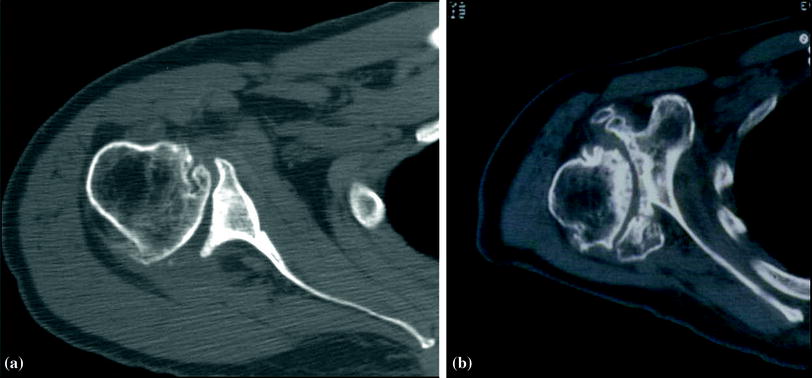
Fig. 2.2
Coronal CT-scan of arthritic shoulder with eccentric wear and biconcave-shaped glenoid (group B2 according to Walch) (a) and dysplastic glenoid with retroversion bigger 25° (Group C according to Walch) (b)
Anatomy of the proximal humerus is not less important, with a huge variety in all anatomic parameters seen in patients. The humeral head, which forms the ball of the glenohumeral joint, is more or less a true sphere. It has a mean diameter of 46–52 mm with a big range seen from 37 to 57 mm in healthy shoulders. There is a direct positive correlation between articular surface diameter and its height, which Boileau et al. defined as “the perpendicular distance from the articular margin to the apex of the diameter of curvature” [16]. Mean inclination angle as measured to the shaft axis is 130°, and its mean retroversion as measured to the transepicondylar axis is 18°. As the transepicondylar axis is difficult to determine in an intraoperative setting, the forearm axis can be taken as reference; however, in this case, a significant higher retroversion of 29° is noted [17]. Again both values show a high variance, with inclination angles varying between 120° and 135° and retroversion between −7° and 48°, respectively. Besides these angles, the humeral articular surface presents a posterior and medial offset relative to the shaft axis. The mean medial offset is 7 mm (3–11 mm), and the mean posterior offset is 2.6 mm (−1 to 6 mm).
Comparing the glenoidal and humeral articular surfaces, a mismatch in size is obvious: while overall glenoid surface area averages about 5–9 cm2, the humeral head surface area is about three times bigger [18, 19]. However, contact area is increased by the labrum, which forms a fibrous ring surrounding the glenoid. The bony shape of the glenoid has a slightly smaller curvature compared to the humeral head. This mismatch is partly compensated by the cartilage, and therefore, humeral head and glenoid surface curvature are more or less congruent, with the difference being <2 mm in the majority of patients [18].
The glenoid labrum and glenohumeral ligaments provide glenohumeral stability, while allowing an almost full circle range of motion. Therefore, their integrity, their position, and orientation are crucial. The glenoid labrum is a circumferential, fibrocartilaginous ring, attached to the glenoid rim. Superiorly, the long head of the biceps and the superior labrum form a complex known as the superior labrum anterior–posterior (SLAP). Lesions of the SLAP-complex can be a cause of shoulder pain especially in overhead athletes. These lesions were classified according to Snyder, who differentiates four subtypes. Maffet later described three more subtypes and extended the classification accordingly [20, 21]. Anteriorly, the superior and medial glenohumeral ligaments reach from the glenoid to the lesser tubercle and the inferior humeral neck. Beneath the medial glenohumeral ligament, the inferior glenohumeral ligament complex reaches downwards and laterally to its insertion on the proximal humerus. In contrast to the single-band structure of the superior and middle glenohumeral ligaments, the inferior ligament complex consists of two bands anteriorly and posteriorly and the axillary pouch in between [22].
The rotator cuff consists of four muscles. The supraspinatus (SSP) muscle originates from the supraspinatus fossa, tracks laterally underneath the acromion and finally inserts at the anterior–superior aspect of the greater tuberosity and in 20 % of patients also at the superior aspect of the lesser tuberosity [23]. Origin of the infraspinatus (ISP) muscle is the fossa infraspinatus on the dorsal aspect of the scapula. The muscle tracks superolateral and finds its insertion at the anterolateral aspect of the greater tuberosity. The footprint of the infraspinatus insertion is of trapezoidal shape and has a maximum length of about 10 mm mediolateral and 30 mm anteroposterior, respectively. Insertion of the teres minor is found at the most posterior and inferior facet of the greater tuberosity. Its origin is on the lateral margin of the scapula, just inferior and lateral to the infraspinatus muscle. Anteriorly, the subscapularis (SSC) muscles origin takes up almost the whole anterior scapula aspect. Its insertion at the lesser tuberosity is of trapezoidal shape, with a broad part superiorly and a thin, narrow part inferiorly. Overall dimensions of the tendinous insertion is about 18 mm width and 25 mm height [24], while muscular insertion is seen inferior to the lesser tuberosity, thus expanding the subscapularis insertion site distally [25].
The deltoid muscle is often referred to as the main engine of the shoulder. It has a triangular shape, with the base of the triangle being its origin on the lateral, anterior clavicle, the acromion, and the scapula spine [26]. Accordingly, the muscle consists of at least three parts: the anterior (clavicular), the lateral (middle) and the posterior (spinal) segments. However, some authors suggest an even greater segmentation, with four middle segments [27]. These segments find a wide insertion on the proximal, lateral humeral shaft. Distally, fascial connections with lateral intermuscular septum and the brachial fascia can be found, which will preserve deltoid function even in case of partial (anterior) release of the deltoid insertion [28]. Anteriorly, the deltoid fibers are adjacent to the pectoralis major muscle. This interval can easily be identified by the cephalic vein, which often will be surrounded by some fat tissue. Feeding vessels will radiate in the vein, with the majority of these vessels being laterally [29].
Biomechanics of Normal and Abnormal Shoulder Structures
The glenohumeral joint can be classified as an enarthrodial ball and socket joint. It allows polyaxial motion of the arm relative to the scapula. These motions can be described relative to the scapular plane in the following manner: humeral rotation around the sagittal axis is specified as abduction/adduction, rotation around the frontal axis, where the axis is directed in a medial–lateral direction, named flexion/extension, and rotational movement around a longitudinal-humeral axis is referred to as external/internal rotation.
Even though articular surfaces are more or less congruent, glenohumeral joint kinematics do not fully equate enarthrodial kinematics. Exact determination of this kinematics has been subject to numerous studies. However, some controversy exists, and results are difficult to compare due to the variety of methodological approaches. Recently, development of open MRI and three-dimensional biplanar fluoroscopy techniques promise a highly increased accuracy and more realistic representation of the actual in vivo situation. Sahara et al. found an overall 1.1 mm inferior humeral translation during abduction using a vertically open MRI-technique. In the anterior–posterior direction, there was a 2.4 mm anterior translation while abduction from 0° to 90° and a posterior translation of −1.4 mm in abduction angles of 90°–150° (Fig. 2.3) [30]. Gibhart et al. found slightly bigger values with maximum superior–inferior translation of 4.2 ± 2.3 mm and 5.1 ± 1.1 mm for anterior–posterior translation, respectively.
These kinematics are highly dependable on the interaction of the rotator cuff, the deltoid and the scapulothoracic muscles. The anterior (SSC) and posterior (ISP/Teres minor) cuff act as a transverse force couple generating compressive forces. These glenohumeral joint reaction forces reach 45–90 % of total body weight, with the maximum forces seen in full abduction position. As a result, the humeral head is maintained centrally in the glenoid fossa, unaffected by the superior translational forces of the deltoid muscle [31]. The superior cuff (SSP) does also contribute to concavity compression, especially at early abduction [32]. However, with isolated supraspinatus tears, maximum joint compression forces remain unchanged, while tears of the anterior or posterior rotator cuff result in significantly decreased compression forces [33]. This decrease in compression forces leads to the inability to withstand the superior translational forces of the deltoid and superior migration of the humeral head might occur.
Motion of the arm is initiated by both the rotator cuff and the deltoid muscle. The muscle’s capacity to generate a rotational force can be estimated by measurement of their moment arm respective to the particular axis. The deltoid muscle with its broad origin and big muscle mass is an effective abductor, external and internal rotator. When the arm is at the neutral, resting position, the lateral (middle) part of the deltoid has an abduction moment arm of about 16 mm, which is considerably smaller compared to the SSP abduction moment arm in this position (23 mm). However, with increasing abduction, this moment arm increases to 30–35 mm, making him the strongest abductor in this position. The bigger SSP moment arm at the resting position underlines its importance in initiating the abduction motion [34].
Humeral rotation is initiated by the anterior and posterior rotator cuff and deltoid portions. The subscapularis has an internal rotational moment arm of about 23 mm in the resting position making him the strongest internal rotator. Posteriorly, the Teres minor has a 17-mm external rotational moment arm and the infraspinatus 24-mm external rotation moment arm, respectively [35, 36]. The anterior and posterior subregions of the deltoid muscle contribute to rotational motion to a lesser degree in healthy shoulders, as their rotational moment arms are smaller with 4-mm external rotation moment arm for the posterior regions and 5-mm internal rotation moment arm for the anterior regions, respectively [37].
While the measurement of moment arms can give a rough estimation about the muscles capacity to generate rotational forces, other factors such as muscle tension and integrity should not be underestimated.
Joint and Muscle Forces in RSA
In RSA, the center of rotation (COR) is shifted medially and inferiorly. This shift affects biomechanical properties of the deltoid muscle and the remaining rotator cuff in various ways: First of all, the deltoid muscle gains tension. This tension is mandatory for prosthetic stability after RSA and does also increase sufficiency of the deltoid when initiating abduction. The total lengthening of the deltoid sums up to about 20 % compared to the normal shoulder [38]. In shoulders with cuff tear arthropathy, where there is a substantial cranialization of the humerus, this effect might even be more pronounced. The medialization of the COR does also affect the deltoids abduction moment arm: While in healthy shoulders, an overall moment arm in respect to the sagittal axis of 16–35 mm can be seen [37, 39], it increases to 50–60 mm following RSA [38, 39]. Additionally, the amount of deltoid muscle fibres positioned lateral to the COR, which therefore have the capacity to create abductional forces increases with medialization of the COR [40]. On the other hand, a decrease of fibres positioned antero- or posteromedial to the COR, which have the potential to create internal or external, rotational forces in healthy shoulders, can be seen.
Analogous to the COR, the humerus with the insertion sites of the rotator cuff is shifted medially and inferiorly in conventional RSA. These changes have a significant influence on the biomechanical properties of the remaining rotator cuff. In patients with cuff tear arthropathy, supra- and infraspinatus seem to be the most commonly involved, whereas teres minor and subscapularis will often remain intact [41, 42]. Even though these muscles are strong external and internal rotators in healthy shoulders and their integrity might be maintained after RSA, humeral rotation often remains compromised or even decreases postoperatively [41, 43, 44]. While the decrease of deltoid fibres with rotational potential after RSA might be one contributing factor to these findings, a change to the biomechanical properties of the remaining rotator cuff has been identified as the other. Following conventional RSA, rotational moment arms of Teres minor show a significant decrease of up to 25 %, whereas the SSC rotational moment arms decrease by up to 36 % [36]. Moreover, muscle tension of both muscles, especially at the resting position of the arm, declines. The overall decrease of muscle length after conventional RSA can be as high as 20 mm for the teres minor and 16 mm for the subscapularis. With increasing humeral abduction, the reduced muscle tension resolves, and the distal muscle segments might even have a minimal increased tension at abduction of 60° or more [36].
One potential way to address these disadvantageous biomechanical properties is to shift the COR laterally. Lateralization can be achieved by implant design or by interposition of an autologous bone block between the glenoid and the baseplate [2, 45]. While with these efforts a lateralization of 7–10 mm in comparison with the conventional RSA is achieved, the COR is still shifted medially compared to normal shoulders. In lateralized RSA, rotational moment arms are preserved and no significant decrease of muscle tension for either SSC or teres minor is observed (Figs. 2.4 and 2.5). Only the very distal regions of the SSC show a slight decrease of muscle tension when the arm is in a 0° abduction position (139 mm vs. 145 mm) [46].
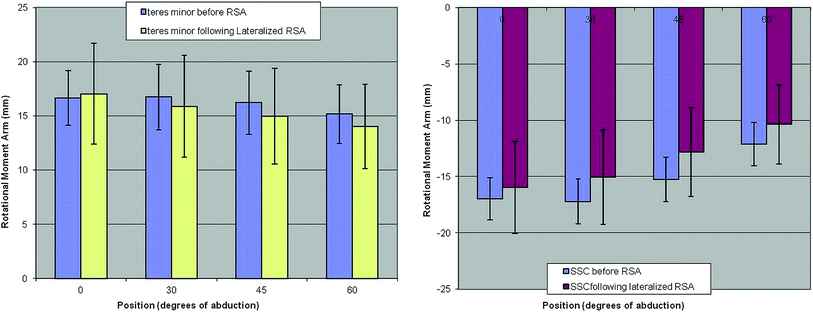
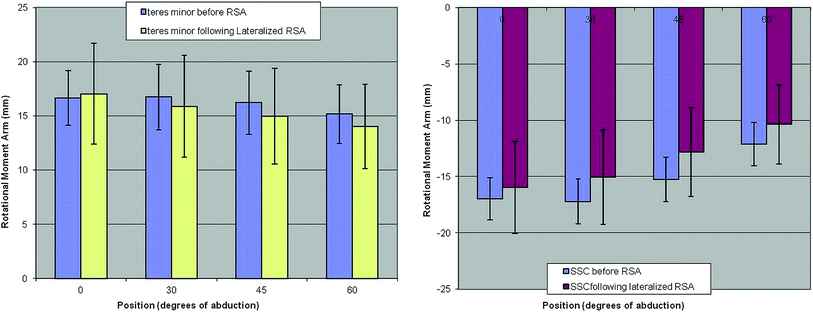
Fig. 2.4
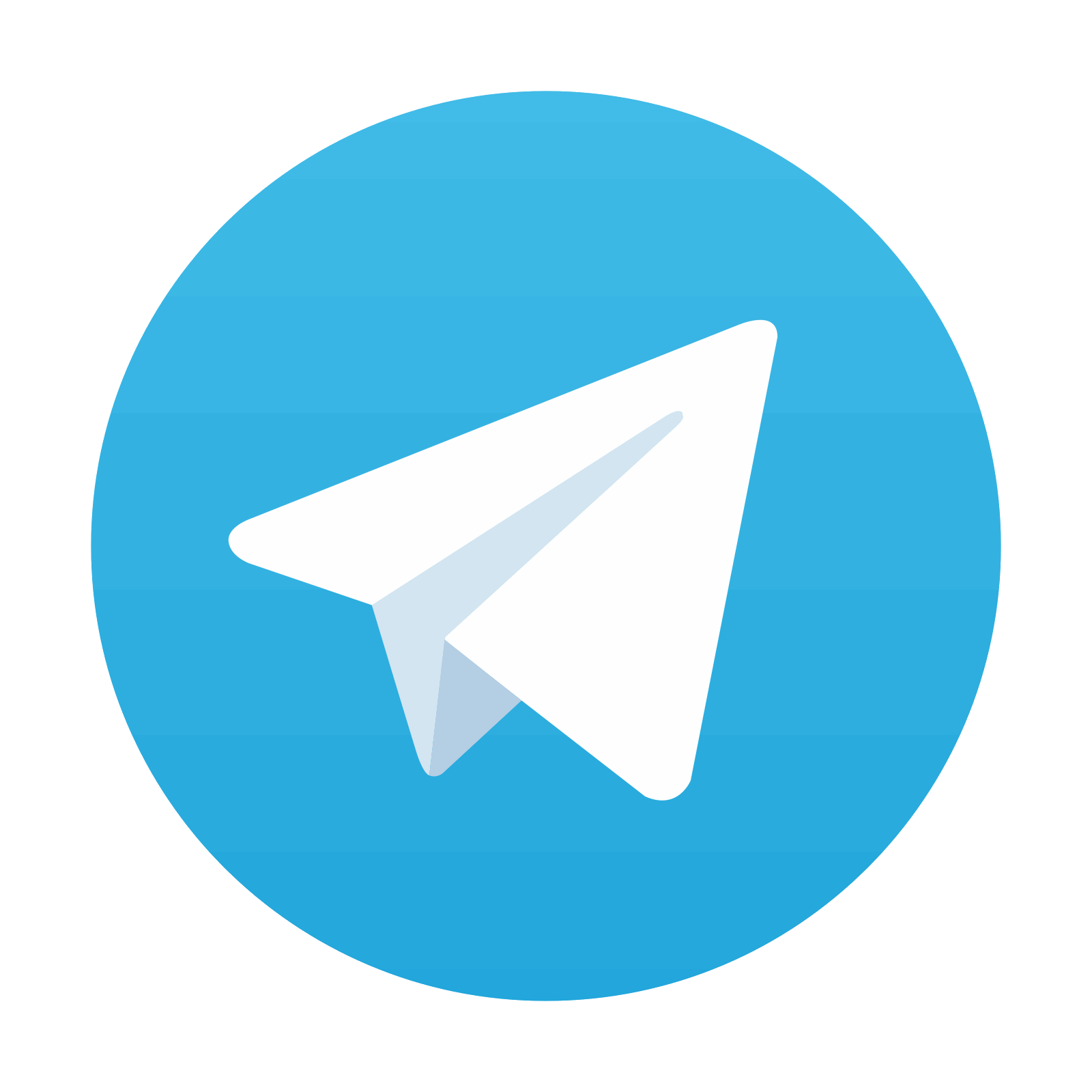
Rotational moment arms (mm) of teres minor and subscapularis before and after lateralized RSA. No significant difference is seen for middle region of teres minor and subscapularis
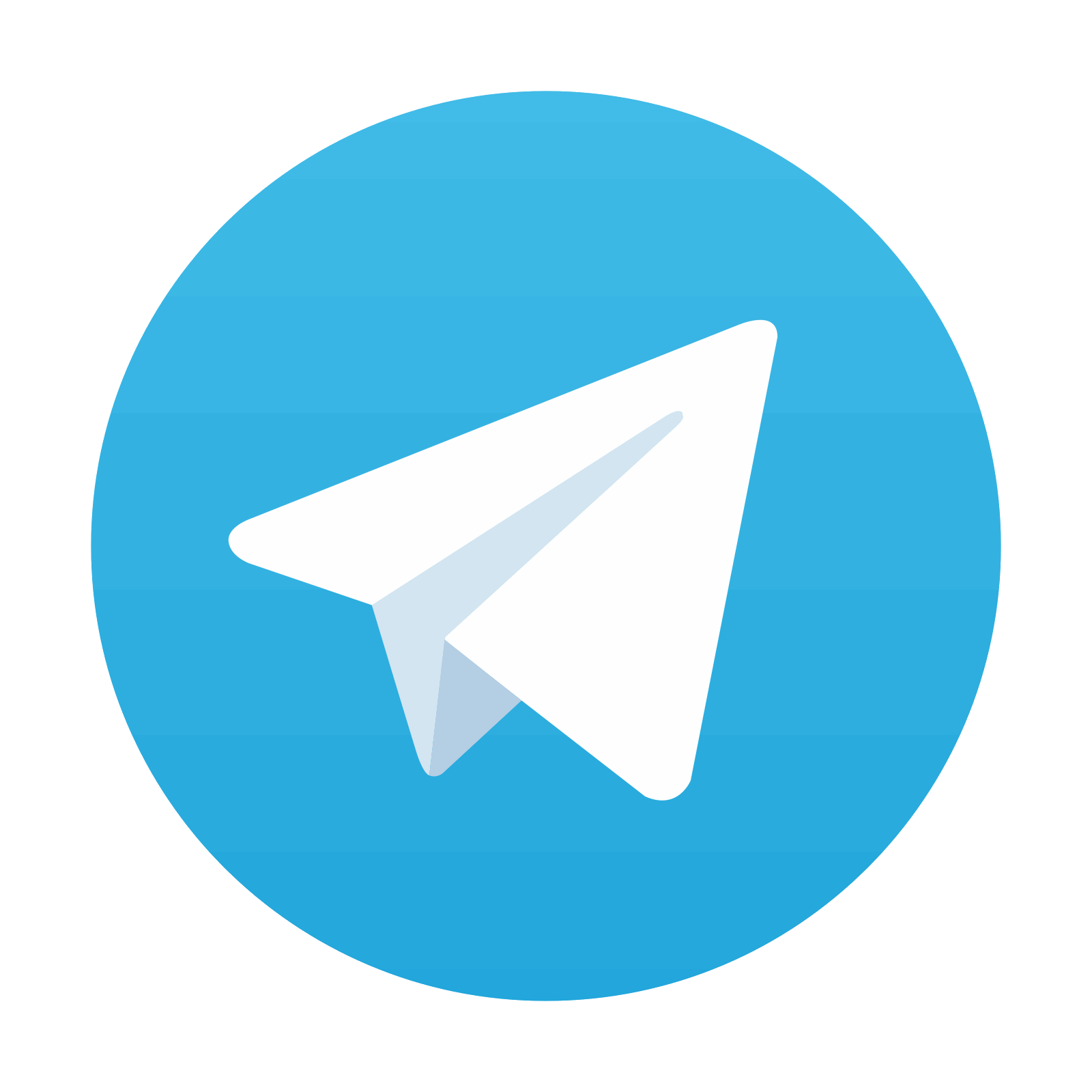
Stay updated, free articles. Join our Telegram channel

Full access? Get Clinical Tree
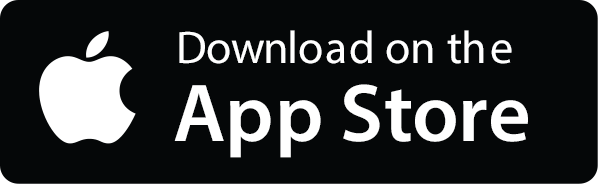
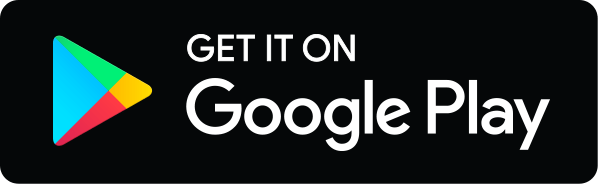
