2 Reverse Total Shoulder Arthroplasty: Biomechanics and Design Challenges
Abstract
Reverse total shoulder arthroplasty was developed to address glenohumeral arthritis subsequent to cuff tear arthropathy; however, current indications continue to evolve. The early biomechanical rationale for the procedure was based on Grammont’s early design concepts; yet, further research has expanded upon these principles to develop the components used today. Current focus on component design, implant positioning, and surgical technique has targeted the goal of optimizing function, while aiming to decrease previously foreseen complications. This includes meeting the demand for improved stability and increased longevity of the prosthesis in the high-demand patient. Still, in order to improve on the success of this procedure, a critical understanding of the biomechanics of reverse total shoulder arthroplasty is needed. In this chapter, we discuss the role of biomechanics and current concepts related to the functioning of reverse total shoulder arthroplasty.
2.1 Introduction
Reverse total shoulder arthroplasty (RTSA) has emerged as a powerful tool to treat pain and loss of function in patients with cuff tear arthropathy. 1 , 2 , 3 Recently, the role of RTSA has been expanded to treat other areas of complex shoulder reconstruction, including comminuted proximal humerus fractures, 2 , 4 revision arthroplasty, 5 , 6 , 7 and cases of irreparable rotator cuff tears. 8 , 9 While contemporary RTSA has expanded its indications and aimed to reduce complications, the biomechanics of RTSA still observe many of the early design principles.
Since the emergence of RTSA, the basis has been to utilize the deltoid force to generate motion of the prosthesis (► Fig. 2.1). Paul Grammont is credited with the novel design of a semiconstrained reverse design prosthesis that resulted in improved survivorship compared to previous implants. 3 , 10 , 11 Using the deltoid to replace a deficient rotator cuff, Grammont’s principles advocated for an inferior medialized center of rotation at the level of the glenoid surface, an inherently stable prosthesis, an effective lever arm of the deltoid throughout the arc range of motion, and a semiconstrained environment with the use of a large glenosphere. This design translated to a fixed center of rotation, with compressive forces being distributed to the glenoid–bone interface during active motion. While survivorship improved, the Grammont design and subsequent generations were still faced with complications related to scapular notching, 12 , 13 , 14 loss of external/internal rotation, 15 adduction deficit, 16 and instability. 17 , 18 In addition, reoperation may be up to 33% in the Grammont-style designs, which could be higher than its predecessors. 19 Today, more recent deigns have aimed to address these shortcomings by aiming to improve positioning, component fixation, and preservation of the native glenohumeral anatomy to improve biomechanics.

In this chapter, we discuss the biomechanics of the cuff-deficient shoulder and address the biomechanical rationale for RTSA—covering component design, implant positioning, range of motion, and contributions to stability. In addition, we discuss considerations of muscular function and look toward future directions of RTSA implant development.
2.2 Biomechanics of the Cuff-Deficient Shoulder
To understand the pathomechanics of the cuff-deficient shoulder, normal function of the rotator cuff should first be discussed. Critical to the biomechanics and stability of the shoulder, both static and dynamic stabilizers are necessary. 20 This includes osseous and capsulolabral restraints, coupled with the rotator cuff, biceps, and periscapular muscles. By allowing for rotation of the humerus and providing compressive stability, the rotator cuff provides a fulcrum for which the muscles of the shoulder girdle can act upon. This allows for concentric rotation of the humeral head, allowing for concavity compression at the mid- and end-range of motion. 21 In contrast, the cuff-deficient shoulder results in muscle imbalance and eventual superior migration of the humeral head (► Fig. 2.2). This can lead to acromial impingement as well as further fraying and degeneration of the remaining intact cuff. It can also lead to synovial thickening and calcium crystal formation, which may initiate a degradation process of both the chondral surfaces and rotator cuff. 22 Nonetheless, the summation of these mechanisms will inevitably lead to pathologic wear of the glenohumeral joint, leading to altered biomechanics and eccentric wear patterns. 23 , 24 Despite the pathomechanism of cuff tear arthropathy being detailed, natural history studies of rotator cuff tears are still limited. 25 , 26 A recent prospective study by Keener et al showed that 49% of asymptomatic tears would undergo enlargement along with 46% developing new pain at a median of 5.1 years. 27

2.3 Glenoid Positioning
The center of rotation of the native shoulder is dynamic throughout its arc range of motion, which is contrary to RTSA which maintains a fixed point. Therefore, the position of the glenoid component is critical to maximizing the lever arm while minimizing the destabilizing shear forces that are experienced. Many of the early constrained and semiconstrained RTSA designs created an increased lever arm that provided greater torque (► Fig. 2.3). The notion was to more closely re-create the native joint center; however, the earliest components resulted in component failure and short-term glenoid loosening. 28

Notably, glenosphere placement still remains a controversial topic today. One stance has been for greater lateral offset of the glenosphere to generate greater torque, along with achieving improved range of motion. 29 This positioning reduces the laxity of the remaining cuff muscles, and can improve cosmetic appearance. 10 , 30 However, this may potentially come at the consequence of increased baseplate motion, which has been linked to loosening, glenosphere migration, and fixation failure. 29 , 31 , 32 Using a Sawbones model, Gutiérrez et al had assessed implant design and center of rotation. 16 Investigating independent factors of position and surgical technique, it was found that lateralizing the center of rotation had the largest effect on increasing range of motion. Additionally, the improved benefits of lateralization have included the ability to more closely recreate the native anatomy and appropriate tensioning of the deltoid. Advances in baseplate fixation have also made lateralization a more attractive choice for component design.
In contrast, medicalization of the center of rotation can lead to impingement or scapular notching, but may minimize the shear forces created at the bone–implant interface. 33 Usually appearing within the first 6 to 14 weeks after surgery, factors related to scapular notching include implant design, glenosphere positioning, and pattern of glenoid wear. 34 It has been shown that notching can occur in up to 68% of cases, and may be more prevalent in those with preoperative superior erosion. 35
Inferior positioning may also help reduce notching, maintaining the center of rotation from the glenoid surface, while at the same time increasing the range of motion without impingement. 16 , 36 , 37 However, issues with distalization can include stretch on the brachial plexus or increased dead space that could theoretically lead to an environment for infection.
Eccentric positioning has also been trialed to improve motion, with specific benefits of increased adduction. 36 , 37 , 38 In support, De Biase et al retrospectively evaluated outcomes of eccentric glenospheres in 40 patients at a minimum of 2 years, which showed no implant-related complications and no evidence of scapular notching. 39
Positioning of the glenoid component will also affect the distribution of forces on the baseplate. Gutiérrez et al assessed the forces at the baseplate–bone interface of the glenosphere component with differing centers of rotation, placed either concentrically or eccentrically (lateral eccentric, inferior eccentric) to the baseplate, in three different tilt positions (superior, neutral, and inferior) (► Fig. 2.4). 40 Using a computer model, they found that the most uniform distribution of forces occurred with a lateralized or concentrically placed glenosphere with inferior tilt. In contrast, superior tilt has been shown to increase baseplate forces, thus leading to earlier loosening. 41 Edwards et al followed this with a prospective clinical study comparing those with inferiorly placed glenoid components at a neutral tilt or 10 degrees of inferior inclination. 42 Still, no observable benefits were found between groups in regard to functional outcomes or rate of scapular notching.

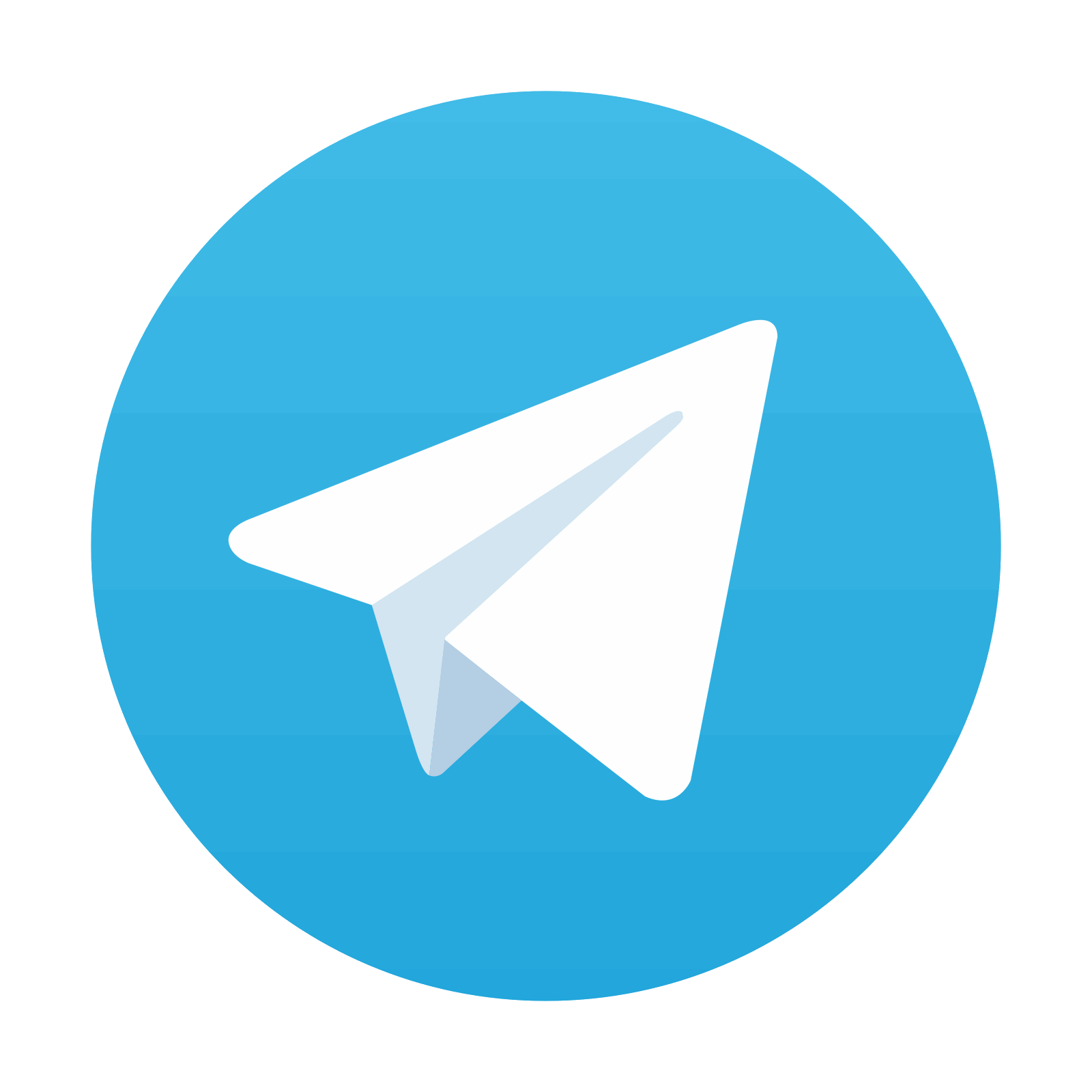
Stay updated, free articles. Join our Telegram channel

Full access? Get Clinical Tree
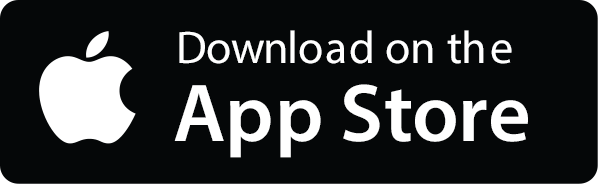
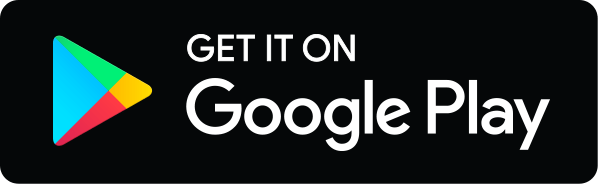