Shoulder Anatomy and Biomechanics During Overhead Motions
James E. Tibone
Rick B. Cunningham
Patrick J. McMahon
INTRODUCTION
The goal in treating the overhead athlete is to eliminate pain and enable return to sports. Current knowledge of shoulder anatomy, biomechanics, and pathoanatomy has dramatically improved treatment of injuries in the overhead athlete. Full function is possible after treatment of common shoulder maladies, such as acromioclavicular joint osteoarthritis (1) and glenohumeral joint instability (2,3).
An enormous range of shoulder mobility is necessary in order to place one’s hand in the many positions demanded by tasks of everyday life. Simple shoulder motions necessitate coordinated actions at four separate articulations. Compared with other diarthrodial joints, there is little inherent bony stability in the glenohumeral joint. Instead, we rely on soft tissues to adequately restrain the shoulder while enabling a full range of motion. When these same soft tissue structures are injured, there can be a significant loss of function. This is especially true when the physiologic limits of the tissues are exceeded or impaired. This situation is less common in the general population, but it is a frequent finding in individuals who participate in competitive overhead athletics. In this population, the athlete repetitively demands the extremes of shoulder range of motion, which maximally stresses both the static and dynamic shoulder restraints, often leading to injury.
In this chapter, the anatomy and biomechanics of the shoulder in the overhead athlete are described. The stages of the throwing motion, including electromyographic (EMG) data, are reviewed for both the normal and the injured shoulder. Baseball pitching is explored in some detail, but swimming and tennis are also considered.
ANATOMY AND BIOMECHANICS: NORMAL AND PATHOLOGIC
Before reviewing shoulder anatomy and biomechanics as they apply to the overhead athlete, some definition of terms is necessary. Even though the term shoulder is often used in reference to the glenohumeral joint, normal shoulder function requires coordinated motion at four articulations: sternoclavicular, acromioclavicular, glenohumeral, and scapulothoracic. Furthermore, the shoulder is composed of 30 muscles, three bones (humerus, clavicle, and scapula), and the upper thorax. In this chapter, shoulder motion refers to the complex interaction of all these structures.
The actions between the humerus and the glenoid are described as follows. Small linear movements that take place between articular surfaces are translations. These translations can be in the anteroposterior, superoinferior, and mediolateral directions. When translation is excessive, there is increased laxity, and when this laxity becomes symptomatic, there is instability. In normal shoulders, motion is composed of small glenohumeral translations and large angular rotations between the humerus and the scapula (4,5). The rotation may be internal-external, adduction-abduction in the scapular plane, and adduction-abduction in the horizontal plane.
Overview of Anatomy Relevant to Throwing and Overhead Athletics
Glenohumeral Anatomy and Motion
The rotator cuff is composed of four muscles: the supraspinatus, subscapularis, infraspinatus, and teres minor. The supraspinatus has its origin on the posterosuperior scapula, superior to the scapular spine. It passes under the acromion,
through the supraspinatus outlet, and inserts on the greater tuberosity. The supraspinatus is active during the entire arc of scapular plane abduction. Intratendinous strain increases as the shoulder is abducted from 15 to 60 degrees (6). Paralysis of the suprascapular nerve results in an approximately 50% loss of abduction torque (7). Simulated supraspinatus retraction in a cadaveric study involving one third, two thirds, and the entire tendon resulted in losses of torque measuring 19%, 36%, and 58% respectively (8). Overuse activity has been thought to be a major contributing factor in the development of supraspinatus tendinopathy. A recent animal study supports this theory, demonstrating an increase in cellularity, a loss of normal collagen fiber organization, and a lower modulus of elasticity and maximum stress to failure (9).
through the supraspinatus outlet, and inserts on the greater tuberosity. The supraspinatus is active during the entire arc of scapular plane abduction. Intratendinous strain increases as the shoulder is abducted from 15 to 60 degrees (6). Paralysis of the suprascapular nerve results in an approximately 50% loss of abduction torque (7). Simulated supraspinatus retraction in a cadaveric study involving one third, two thirds, and the entire tendon resulted in losses of torque measuring 19%, 36%, and 58% respectively (8). Overuse activity has been thought to be a major contributing factor in the development of supraspinatus tendinopathy. A recent animal study supports this theory, demonstrating an increase in cellularity, a loss of normal collagen fiber organization, and a lower modulus of elasticity and maximum stress to failure (9).
The infraspinatus and teres minor muscles originate on the posterior scapula, inferior to the scapular spine, and insert on the posterior aspect of the greater tuberosity. Their tendinous insertions are not separate from each other or the supraspinatus tendon. In this way, the rotator cuff tendon differs morphologically from a typical tendon. It consists of an interdigitating confluence of collagen bundles from all of the rotator cuff muscles, forming a hood or aponeurosis over the humeral head (10). The infraspinatus and teres minor act together to externally rotate and extend the humerus. Together, these muscles contribute approximately 80% of the external rotation strength with the arm in an adducted position.
The subscapularis muscle arises from the anterior scapula and is the only muscle to insert on the lesser tuberosity. It acts to internally rotate and flex the humerus. The tendinous insertion of the subscapularis is continuous with lateral aspect of the anterior capsule; therefore, these two structures have been considered responsible for providing anterior glenohumeral stability (11,12).
The orientation of the shoulder muscles to the glenohumeral joint is such that the joint reaction force has a large component that acts perpendicular to the glenoid fossa (13) to compress the concave humeral head into the glenoid fossa. Labeled “concavity-compression” by Lippett and co-workers (14), this maintains anterior joint stability over a large range of shoulder motion (13, 14, 15). Likewise, simulated inactivity of the supraspinatus and subscapularis muscles yielded an 18% and 17% decrease, respectively, in the force needed to dislocate the joint (16). Glenohumeral joint compression through muscle contraction was also found to be responsible for resisting inferior translation of the humeral head; ligament tension and negative intraarticular pressure were less important (17). With traumatic instability, there is increased translation in functionally important arm positions, and dynamic stabilizers are often able to re-center the humeral head. However, in atraumatic instability, the head remains decentralized despite muscle activity (18).
The deltoid is the largest shoulder girdle muscle, and it envelops the proximal humerus. It has a tripennate origin at the clavicle, acromion, and scapular spine and inserts at the deltoid tubercle on the lateral aspect of the humeral shaft. The three muscle bellies of the deltoid have different functions. The anterior portion is primarily a forward flexor and abductor of the glenohumeral joint; the middle portion is an abductor; and the posterior portion extends the humerus. The middle and posterior heads provide more glenohumeral compression and less shear than does the anterior head of the deltoid (19). The deltoid is active throughout the entire arc of glenohumeral abduction. Paralysis of the axillary nerve results in a 50% loss of abduction torque (7). The deltoid muscle can fully abduct the glenohumeral joint with the supraspinatus muscle inactive.
The humeral head is approximately one third of a sphere, with the diameter being an average of 45 mm. The glenoid has a 35-mm vertical diameter and a 25-mm horizontal diameter (20, 21, 22, 23, 24, 25, 26). Relative to the plane of the scapula, the glenoid is angled superiorly and posteriorly approximately 5 degrees (5,25). This glenoid orientation offers little bony restraint to inferior instability with the arm at the side. The anterosuperior aspect of the glenoid is the area of maximum contact stresses in the normal shoulder (27).
The proximal humerus is made up of four anatomic parts: the articular surface, the greater tuberosity, the lesser tuberosity, and the diaphyseal shaft. The humeral head is angulated medially 45 degrees to the long axis of the humeral shaft, and on average it is retroverted 20-25 degrees relative to the transcondylar axis of the distal humerus (26). However, a study of asymptomatic college baseball players found that mean humeral retroversion was 36.6 degrees in the dominant extremity and 26 degrees in the nondominant extremity. This adaptive change in proximal humeral anatomy may account for the loss of internal rotation and gains in external rotation seen in pitchers’ dominant extremity more than would alterations in the soft tissues (28).
Between the two tuberosities is the intertubercular groove, in which lies the tendon of the long head of the biceps brachii. This tendon is held in place by the coracohumeral ligament, superior glenohumeral ligament, and the transverse humeral ligament. During abduction of the glenohumeral joint, the proximal humerus slides on the tendon of the long head of the biceps brachii. If the tendon ruptures, translation of the humeral head is increased (29). An EMG study suggests that, in a patient with traumatic unilateral anterior instability, the biceps muscle plays an active compensatory role in stabilizing the shoulder when it is in an abducted and externally rotated position (30).
Soslowsky and co-workers (31) used stereophotogrammetry to demonstrate that the radius of curvature of the humeral head and the glenoid are not statistically different. Additionally, they showed that together both surfaces approximate the surface of a sphere. However, this point is controversial, and others believe that the surfaces are not conforming and that the contact between them is variable (32,33).
In the glenohumeral joint, three different types of motion may occur: spinning, sliding, and rolling (33). Spinning occurs when the contact point on the glenoid remains the same while the humeral head contact point is changing. Sliding is pure translation of the humeral head on the articular surface of the glenoid. At the extremes of motion, and certainly in unstable joints, glenohumeral sliding occurs. In this circumstance, the contact point on the glenoid is moving, while that for the humerus remains the same. The third type of action, rolling, may also occur at the glenohumeral joint. Rolling is a combination of humeral head sliding and spinning relative to the glenoid such that the contact point changes on both the glenoid and the humeral head (33).
Capsuloligamentous and Labral Anatomy
Although originally disputed, it is now agreed that glenohumeral ligaments are consistently present, although some variation in their insertion sites has been described. The superior glenohumeral ligament (SGHL) arises at the supraglenoid tubercle just anterior to the long head of the biceps brachii (Fig. 2-1). If the glenoid has the markings of a clock with the 12 o’clock position superiorly and the 3 o’clock position anteriorly, then the origin of the SGHL would correspond to the area from the 12 o’clock to the 2 o’clock positions. The SGHL runs inferiorly and laterally to insert on the humerus, just superior to the lesser tuberosity. The SGHL along with the coracohumeral ligament is part of the rotator interval.
The middle glenohumeral ligament (MGHL) usually arises from the neck of the glenoid just inferior to the origin of the SGHL. The MGHL may also originate with the SGHL, with the SGHL and long biceps tendon, or with the long biceps tendon with an absent SGHL (34). Its fibers blend in with the subscapularis tendon before inserting on the lesser tuberosity. The presence of the MGHL is the most variable (35). In a magnetic resonance imaging (MRI) study of asymptomatic volunteers, it was found in only 79% of patients (36).
The inferior glenohumeral ligament (IGHL) is a complex structure. The IGHL has an anterior and a posterior band, with an axillary pouch between the two bands (37). In abduction and external rotation, the anterior band fans out, and the posterior band becomes cordlike. Likewise, with internal rotation, the posterior band fans out, and the anterior band appears cordlike. The posterior band originates between the 7 o’clock and 9 o’clock position from the glenoid. With the arm at the side, both the anterior and posterior bands pass through a 90-degree arc and insert on the humerus.
The anterior band of the IGHL arises between the 2 o’clock and the 4 o’clock position on the glenoid. In most people, the anterior band of the IGHL has its origin from
the labrum with some fibers extending to the glenoid neck; however, in some shoulders, the anterior band has its origin solely on the glenoid neck (38). In a cadaveric study simulating the late cocking phase of throwing, it was found that cutting the entire IGHL resulted in the greatest increase in external rotation; however, this increase in external rotation was not significantly different from sectioning the coracohumeral ligament. Furthermore, cutting the anterior band of the IGHL alone caused little increase in external rotation (39). In a cadaveric study simulating a first time anterior shoulder dislocation and Bankart lesion, it was found that there was little unrecoverable stretching of the IGHL (40). However, with repetitive loading of the inferior glenohumeral ligament, the ligament becomes lax with an unrecoverable increase in length of the ligament (41).
the labrum with some fibers extending to the glenoid neck; however, in some shoulders, the anterior band has its origin solely on the glenoid neck (38). In a cadaveric study simulating the late cocking phase of throwing, it was found that cutting the entire IGHL resulted in the greatest increase in external rotation; however, this increase in external rotation was not significantly different from sectioning the coracohumeral ligament. Furthermore, cutting the anterior band of the IGHL alone caused little increase in external rotation (39). In a cadaveric study simulating a first time anterior shoulder dislocation and Bankart lesion, it was found that there was little unrecoverable stretching of the IGHL (40). However, with repetitive loading of the inferior glenohumeral ligament, the ligament becomes lax with an unrecoverable increase in length of the ligament (41).
The labrum is located along the periphery of the glenoid and is the site of attachment of the capsuloligamentous structures and the long head of the biceps brachii tendon. Huber and Putz (42) showed that the tendon fibers of the long head of the biceps continued posteriorly as periarticular fiber bundles, thereby constituting the “labrum” in the posterior superior quadrant. The remaining labrum is composed of dense fibrous connective tissue with a small fibrocartilaginous transition zone at the anterior inferior attachment of the osseous glenoid rim (38,43,44). Several investigators have suggested that the labrum may act as a load-bearing structure for the humeral head and serve to increase the surface area of the glenoid (31,32). Howell and Galinat (45) showed that the labrum deepened the glenoid socket by nearly 50%. Lippett and co-workers have shown that removal of the labrum decreases the joint’s stability to shear stress by 20% (14). However, a recent cadaveric study found that the average contribution of the labrum to glenohumeral stability through concavity compression was only 10% (46).
Scapulothoracic Anatomy and Motion
The scapulothoracic muscles include the trapezius (upper, middle, and lower portions), levator scapulae, serratus anterior, pectoralis minor, and rhomboids. Postural support is provided by the levator scapulae and the upper trapezius. The middle trapezius and rhomboids retract the scapula, whereas the serratus anterior protracts the scapula. Upward rotation of the scapula is accomplished by the trapezius and serratus anterior. The upper trapezius and levator scapulae can also elevate the scapula. These muscles position the scapula on the thoracic cage in order to allow for maximum shoulder motion and stability.
Motion between the scapula and the thorax—the scapulothoracic articulation—is an integral part of normal shoulder function. Other than muscular attachments, the scapula is supported only by the acromioclavicular joint and the coracoclavicular ligaments. Because of this, the scapula is able to move in many directions. The relative motion of the scapulothoracic articulation and glenohumeral joint during abduction is termed the scapulothoracic rhythm. In the first 30 degrees of abduction, glenohumeral motion is greater than scapulothoracic motion by up to a 7:1 ratio (5,47). However, this ratio changes with the speed at which the arm is abducted; at high speeds, glenohumeral motion is more dominant than at slower speeds of abduction (48). Beyond 30 degrees, both joints contribute equally to shoulder abduction (5,47). Thus, over the entire arc of abduction, more motion occurs at the glenohumeral joint than the scapulothoracic joint. The overall mean ratio of glenohumeral to scapulothoracic motion is approximately 1.7:1 (49).
Normal scapular motion consists of substantial rotations around 3 axes, not simply upward rotation. In the resting position, the superior edge of the scapular spine is rotated anteriorly from the frontal plane a mean of 31 degrees (50). With the initiation of abduction, the scapula can translate medially or laterally, or in rare instances, oscillate on the chest wall (26). As the shoulder abducts, the scapula rotates not only in the plane of abduction, but also in a plane perpendicular to it. The superior edge of the scapula rotates anteriorly about 6 degrees during the first 90 degrees of shoulder abduction. Beyond 90 degrees, 16 degrees of posterior scapular rotation occurs (51).
Acromioclavicular and Sternoclavicular Anatomy and Motion
The sternoclavicular joint is stabilized by four ligaments and an intraarticular disk. The interclavicular ligament provides restraint to superior joint motion and is taut when the shoulder is at the side (52). Anterior and posterior motion is prevented by anterior and posterior capsular structures (5). The posterior capsule is the most important restraint for anterior and posterior translation of the sternoclavicular joint. The anterior capsule is a secondary restraint to anterior translation (53). Inferiorly, the joint is stabilized by the costoclavicular ligaments, which run obliquely and laterally from the first rib to the clavicle. The costoclavicular and interclavicular ligaments have little effect on anterior or posterior translation of the sternoclavicular joint (53).
At the acromioclavicular joint, superior motion of the distal clavicle is prevented by the coracoclavicular ligaments: the conoid and the trapezoid. The acromioclavicular capsule is the primary restraint to anterior and posterior motion, with the acromioclavicular ligament being a superior thickening of the capsule.
During shoulder abduction, the clavicle rotates approximately 30 degrees superiorly in the coronal plane. The clavicle also rotates anteriorly approximately 10 degrees as the shoulder is abducted from 0 to 40 degrees. There is an additional 15 degrees of anterior rotation of the clavicle when the shoulder is abducted from 130 to 180 degrees (26). The
large axial rotations initially reported by Inman and colleagues (26) have since been questioned. By placing pins in the clavicle and the acromion, Rockwood and Green (52). have shown that the clavicle rotates axially less than 10 degrees over the entire arc of shoulder abduction. This finding is supported by the clinical observation that fixing the clavicle to the scapula does not significantly limit shoulder motion.
large axial rotations initially reported by Inman and colleagues (26) have since been questioned. By placing pins in the clavicle and the acromion, Rockwood and Green (52). have shown that the clavicle rotates axially less than 10 degrees over the entire arc of shoulder abduction. This finding is supported by the clinical observation that fixing the clavicle to the scapula does not significantly limit shoulder motion.
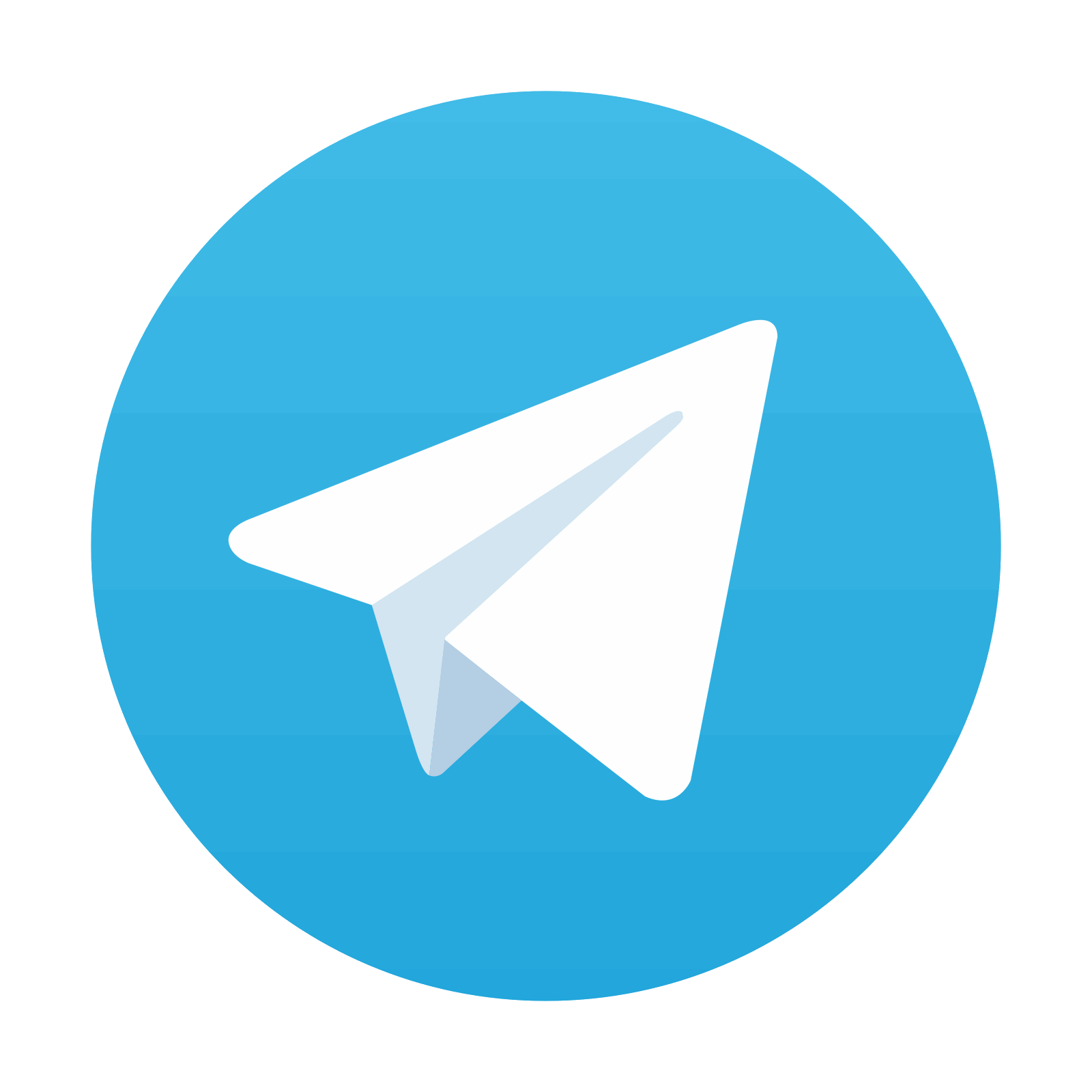
Stay updated, free articles. Join our Telegram channel

Full access? Get Clinical Tree
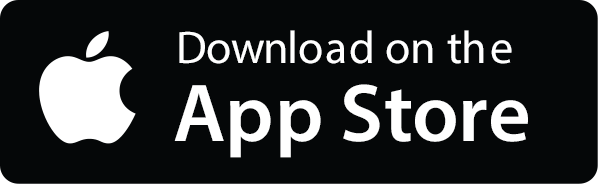
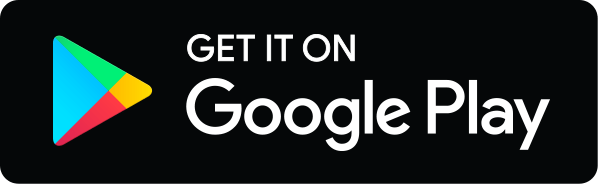
