CHAPTER 8 Sciatica and Nerve Root Pain in Disc Herniation and Spinal Stenosis
A Basic Science Review and Clinical Perspective
The clinical symptoms seen in association with lumbar disc herniation and spinal stenosis1,2 are based on pathophysiologic involvement of spinal nerve roots. There has been an increasing interest in this topic during the past decade, and more recent research has been aimed at defining basic pathophysiologic events at the tissue, cellular, or subcellular level that are involved in the generation of sciatica and nerve root pain. This chapter reviews the current knowledge about these mechanisms and discusses these mechanisms in relation to the clinical features of lumbar disc herniation and spinal stenosis.
Pathophysiologic Mechanisms in Relation to Clinical Symptoms
The symptoms of nerve root pathophysiology may be divided into two main categories: pain and nerve dysfunction.2 Nerve root pain is typically radiating in nature and is usually related to a specific nerve root or roots. Nerve dysfunction may be present in motor and sensory modalities, producing motor weakness and sensory disturbances. One may assume that pain and nerve dysfunction are due to different pathophysiologic events, but they are tightly linked through mechanisms that are discussed in this chapter.
Two specific mechanisms at the “tissue level” may be defined: (1) mechanical deformation of the nerve roots and (2) biologic or biochemical activity of the disc tissue with effects on the roots. The mechanical deformation theory is the oldest concept of nerve root injury induced by herniated disc tissue and dates back to the turn of the 20th century with clinical observations on injuries in the lumbosacral junction with subsequent leg pain and includes the more recent seminal observations of Mixter and Barr.1–5 The theory that biologic activity of the disc tissue may injure the nerve roots was demonstrated experimentally in 1993.6 The experimental knowledge regarding these two mechanisms is discussed separately.
Mechanical Effects on Nerve Roots
Enclosed by the vertebral bones, the spinal nerve roots are relatively well protected from external trauma. The nerve roots do not possess the same amounts and organization of protective connective tissue sheaths as do the peripheral nerves, however. The spinal nerve roots may be particularly sensitive to mechanical deformation secondary to intraspinal disorders, such as disc herniations and protrusions, spinal stenosis, degenerative disorders, and tumors.7–9 There has been moderate research interest in the past regarding nerve root compression. Gelfan and Tarlov10 in 1956 and Sharpless11 in 1975 performed some initial experiments on the effects of compression on nerve impulse conduction. Although no calibration was performed on the compression devices used, the results of both studies indicated that nerve roots were more susceptible to compression than peripheral nerves. Interest in nerve root pathophysiology has increased considerably more recently, and numerous studies are reviewed here.
Experimental Compression of Nerve Roots
In 1991, a model was presented that for the first time allowed for experimental, graded compression of cauda equina nerve roots at known pressure levels.7,8 In this model, the cauda equina of pigs was compressed by an inflatable balloon that was fixed to the spine (Fig. 8–1). The cauda equina could also be observed through the translucent balloon. This model made it possible to study the flow in the intrinsic nerve root blood vessels at various pressure levels12 because the blood flow and vessel diameters of the intrinsic vessels could be observed simultaneously through the balloon with the use of a vital microscope. The average occlusion pressure for the arterioles was found to be slightly below and directly related to the systolic blood pressure. The blood flow in the capillary networks was intimately dependent on the blood flow of the adjacent venules. This finding corroborates the assumption that venular stasis may induce capillary stasis and changes in the microcirculation of the nerve tissue, which has been suggested as one mechanism in carpal tunnel syndrome.13 The mean occlusion pressures for the venules showed large variations. A pressure of 5 to 10 mm Hg was found to be sufficient for inducing venular occlusion. Because of retrograde stasis, it is assumed that the capillary blood flow also would be affected in such situations.
In the same experimental setup, the effects of gradual decompression, after initial acute compression maintained for only a short while, were studied.14 The average pressure for starting the blood flow was seen to be slightly lower at decompression than at compression for arterioles, capillaries, and venules. With this protocol, there was not a full restoration of the blood flow, however, until the compression was reduced from 5 to 0 mm Hg. This observation stresses further the previous impression that vascular impairment is present even at low pressure levels.
Because the nutrition of the nerve root is affected, a compression-induced impairment of the vasculature may be one mechanism for nerve root dysfunction. The nerve roots also have a considerable nutritional supply, however, via diffusion from the cerebrospinal fluid.15 To assess the compression-induced effects on the total contribution to the nerve roots, an experiment was designed in which 3H-labeled methylglucose was allowed to be transported to the nerve tissue in the compressed segment via the blood vessels and via the cerebrospinal fluid diffusion after systemic injection.16 The results showed that no compensatory mechanism from cerebrospinal fluid diffusion could be expected at the low pressure levels. On the contrary, 10 mm Hg compression was sufficient to induce a 20% to 30% reduction of the transport of methylglucose to the nerve roots compared with control.
It is known from experimental studies on peripheral nerves that compression also may induce an increase in the vascular permeability, leading to intraneural edema formation.17 Such edema may increase the endoneurial fluid pressure, which may impair the endoneurial capillary blood flow and jeopardize the nutrition of the nerve roots.18–20 Because the edema usually persists for some time after the removal of a compressive agent, edema may negatively affect the nerve root for a longer period than the compression itself. The presence of intraneural edema is also related to subsequent formation of intraneural fibrosis21 and may contribute to the slow recovery seen in some patients with nerve compression disorders. To assess if intraneural edema also may form in nerve roots as the result of compression, the distribution of Evans blue–labeled albumin in the nerve tissue was analyzed after compression at various pressures and at various durations.22 The study showed that edema was formed even at low-pressure levels. The predominant location was at the edges of the compression zone.
The function of the nerve roots has been studied by direct electrical stimulation and recordings either on the nerve itself or in the corresponding muscular segments.23–26 During a 2-hour compression period, a critical pressure level for inducing a reduction of minimal alveolar pressure or amplitude was between 50 mm Hg and 75 mm Hg. Higher pressure levels (100 to 200 mm Hg) induced a total conduction block with varying degrees of recovery after compression release. To study the effects of compression on sensory nerve fibers, the electrodes in the sacrum were instead used to record a compound nerve action potential after stimulating the sensory nerves in the tail (i.e., distal to the compression zone). The results showed that the sensory fibers were slightly more susceptible to compression than the motor fibers.25,26 Also, the nerve roots were more susceptible to compression injury if the blood pressure was reduced pharmacologically.24 This finding further implies the importance of the blood supply to maintain the functional properties of the nerve roots.
Onset Rate of Compression
A rapid onset rate of less than 1 second has been found to induce more pronounced edema formation,22 methylglucose transport,16 and impulse propagation23 than a slow onset rate of approximately 20 seconds. Regarding methylglucose transport, the results show that the levels within the compression zone are more pronounced at a rapid onset rate than at a slow onset rate at corresponding pressure levels. There was also a striking difference between the two onset rates when considering the segments outside the compression zones. In the slow onset series, the levels approached baseline values closer to the compression zone than in the rapid onset series; this may indicate the presence of a more pronounced edge-zone edema in the rapid onset series, with a subsequent reduction of the nutritional transport also in the nerve tissue adjacent to the compression zone.
For the rapid onset compression, which is likely to be more closely related to spine trauma or disc herniation than to spinal stenosis, it has been seen that a pressure of 600 mm Hg maintained only for 1 second is sufficient to induce a gradual impairment of nerve conduction during the 2 hours studied after the compression was ended.27 Overall, the mechanisms for these pronounced differences between the different onset rates are unclear, but they may be related to differences in displacement rates of the compressed nerve tissue toward the uncompressed parts, owing to the viscoelastic properties of the nerve tissue.9 Such phenomena may lead not only to structural damage to the nerve fibers but also to structural changes in the blood vessels with subsequent edema formation. The gradual formation of intraneural edema may also be closely related to the described observations of a gradually increasing difference in nerve conduction impairment between the two onset rates.22,23 In the case of spinal stenosis, the rate may be a great deal slower, and pain or nerve dysfunction may not be seen until after considerable ischemic injury.
Double or Multiple Levels of Nerve Root Compression
Patients with double or multiple levels of spinal stenosis seem to have more pronounced symptoms than patients with stenosis only at one level.28 The presented model was modified to address this clinical issue. Using two balloons at two adjacent disc levels, which resulted in a 10-mm uncompressed nerve segment between the balloons, induced a much more pronounced impairment of nerve impulse conduction than had been previously found at corresponding pressure levels.29 A pressure of 10 mm Hg in two balloons induced a 60% reduction of nerve impulse amplitude during 2 hours of compression, whereas 50 mm Hg in one balloon showed no reduction.
The mechanism for the difference between single and double compression may not simply be based on the fact that the nerve impulses have to pass more than one compression zone at double level compression. There may also be a mechanism based on the local vascular anatomy of the nerve roots. In contrast to peripheral nerves, there are no regional nutritive arteries from surrounding structures to the intraneural vascular system in spinal nerve roots.7,30–33 Compression at two levels might induce a nutritionally impaired region between the two compression sites. In this way the segment affected by the compression would be widened from one balloon diameter (10 mm) to two balloon diameters, including the nerve segment (30 mm) in between. This hypothesis was partly confirmed in an experiment on continuous analyses of the total blood flow in the uncompressed nerve segment located between two compression balloons. The results showed that a 64% reduction of total blood flow in the uncompressed segment was induced when both balloons were inflated to 10 mm Hg.34 At a pressure close to the systemic blood pressure, there was complete ischemia in the nerve segment. Data from a study on the nutritional transport to the nerve tissue in double-level compression showed that there is a reduction of this transport to the uncompressed nerve segment located between the two compression balloons that was similar to the reduction within the two compression sites.35 There is experimental evidence that the nutrition to the nerve segment located between two compression sites in nerve roots is severely impaired, although this nerve segment itself is uncompressed.
Regarding nerve conduction, it was also evident that the effects were enhanced if the distance between the compression balloons was increased from one vertebral segment to two vertebral segments.29 This was not the case, however, in the nutritional transport study where the methylglucose levels in the compression zones and in the uncompressed intermediate segment were similar between double compression over one and two vertebral segments.35 This similarity indicates that the nutrition to the uncompressed nerve segment located between two compression sites is affected almost to the same extent as at the compression sites, regardless of the distance between the compression sites but that functional impairment may be directly related to the distance between the two compression sites. The impairment of the nutrition to the nerve segment between the two compression balloons seems to be a more important mechanism than the fact that the nerve impulses have to overcome two compression sites in double-level compression.
By using electrical nerve root stimulation to increase metabolic rate and simulate a walking situation in the double-level compression model, an initial short-term increase in cauda equina blood flow was seen that rapidly decreased.36 Such observations further support the pathophysiologic significance of double-level cauda equina compression in spinal stenosis.
Chronic Experimental Nerve Root Compression
Delamarter and colleagues37 presented a model on the dog cauda equina in which they applied a constricting plastic band. The band was tightened around the thecal sac to induce a 25%, 50%, or 75% reduction of the cross-sectional area. The band was left in its place for various times. Analyses were performed and showed structural and functional changes that were proportional to the degree of constriction.
To induce a slower onset and more controlled compression, Cornefjord and colleagues38 used a constrictor to compress the nerve roots in the pig. The constrictor was initially intended for inducing vascular occlusion in experimental ischemic conditions in dogs. The constrictor consists of an outer metal shell that on the inside is covered with a material called ameroid that expands when in contact with fluids. Because of the metal shell, the ameroid expands inward with a maximum of expansion after 2 weeks, resulting in a compression of a nerve root placed in the central opening of the constrictor. Compression of the first sacral nerve root in the pig resulted in a significant reduction of nerve conduction velocity and axonal injuries.38 It has also been found that there is an increase in substance P in the nerve root and the dorsal root ganglion after such compression.39 Substance P is a neurotransmitter that is related to pain transmission. The study may provide experimental evidence that compression of nerve roots produces pain. The constrictor model has also been used to study blood flow changes in the nerve root vasculature.40 It could be observed that the blood flow is not reduced just outside the compression zone but significantly reduced in parts of the nerve roots located inside the constrictor.
One important aspect in clinical nerve root compression conditions is that the compression level is probably unstable and varies as the result of changes in posture and movements.41,42 In 1995, Konno and colleagues43 introduced a model in which the pressure could be changed after some time of initial chronic compression. An inflatable balloon was introduced under the lamina of the seventh lumbar vertebrae in the dog. The normal anatomy and the effects of acute compression using compressed air were first evaluated in previous studies.44 By inflating the balloon at a known pressure slowly over 1 hour with a viscous substance that would harden in the balloon, a compression of the cauda equina could be induced with a known initial pressure level. The compression was verified by myelography. Because the balloon under the lamina comprised a twin set of balloons, the second balloon component could be connected to a compressed air device and could be used to add compression to the already chronically compressed cauda equina.
Spinal Stenosis: Experimental-Clinical Correlation
If nerve compression is of an extremely low onset rate as in spinal stenosis, there may be an adaptation of the nerve tissue to the applied pressure. In cadaveric experiments, Schönström and colleagues45 found that when a hose clamp was tightened around a human cadaveric cauda equina specimen there was a critical cross-sectional area of the dural sac when the first signs of pressure increase among nerve roots were recorded by a catheter placed in the compression zone. This cross-sectional area was approximately 75 mm2, which was also found to correlate with a corresponding measurement on computed tomography (CT) in patients with spinal stenosis.46 When the hose clamp was tightened further, the pressure increased. Owing to creep phenomena in the nerve tissue, the pressure decreased with time, however. When the pressure did not normalize within 10 minutes, the “sustained size” was registered and was found to be in the range of 45 to 50 mm2.45 This study indicates that even in acute compression there is an adaptation of the nerve tissue to the applied pressure. From a longer perspective, this probably means that the nerve may also be reorganized in its microstructural elements, which would result in a nerve with a smaller diameter. Under such circumstances, with gradually decreasing nerve diameter, the nerve pressure acting on the nerve would be reduced to some degree.
There is a correlation between the animal experimental observations regarding critical pressures for functional and nutritional changes in nerve roots under compression on one side and the measurements of pressure levels among nerve roots in human cadaveric lumbar spines after experimental constriction of the dural sac. An acute pressure increase among cauda equina nerve roots to 50 mm Hg was induced when the cross-sectional area of the dural sac was reduced to 63 mm2, and a pressure of 100 mm Hg was induced at a cross-sectional area of 57 mm2.45 Such pressure levels correlate with in vivo observations regarding physiologic changes in cauda equina nerve roots after experimental compression.7,12,22
Epidural pressure measurements have been performed, evaluating the relationship between epidural pressure and posture.42 It was found that the local epidural pressure at the stenotic level was low in lying and sitting postures and high in standing postures. Pressure was increased with extension but decreased with flexion of the spine. The highest epidural pressure, 117 mm Hg, was found in standing with extension. Measurements have also been reported regarding changes in epidural pressure during walking in patients with lumbar spinal stenosis.47 The pressure changed during walking with a wave pattern of increase and decrease. Such observations correlate with the previously mentioned experimental observations regarding intermittent cauda equina compression.41
Mechanical Nerve Root Deformation and Pain
Some experimental observations indicate that mechanical nerve root deformation per se may induce impulses that cause pain. Howe and colleagues48 found that mechanical stimulation of nerve roots or peripheral nerves resulted in nerve impulses of short duration and that these impulses were prolonged if the nerve tissue had been exposed to mechanical irritation by a chromic gut ligature for 2 to 4 weeks. Corresponding results were obtained in an in vitro system using rabbit nerve roots.49 In this setup, it was also evident that the dorsal root ganglion was more susceptible to mechanical stimulation than the nerve roots. The dorsal root ganglion has elicited special interest in this regard, and an increase in the level of neurotransmitters related to pain transmission has been found in the dorsal root ganglion in response to whole-body vibration of rabbits.50 A similar increase has been seen in the dorsal root ganglion and nerve root after local constriction of the same nerve root.39 In vivo models of pain behavior have shown that mechanical nerve deformation superimposed on inflammation is painful, whereas either factor alone might not cause severe pain.51–56 The magnitude of nerve root compression pressure (measured intraoperatively) correlates with neurologic deficit but not with degree of straight-leg raising test.57
Neuropathologic Changes and Pain
There is considerable research evidence regarding the relationship of pain to neuropathologic changes.58 Much of what is known has been studied in relationship to mechanical and inflammatory injury of the sciatic nerve in the rat. Entrapment of a peripheral nerve produces pathologic change in proportion to the degree of compression and its duration,59 as is known to be the case for nerve root compression. In an electron microscopic study,59 minor degrees of nerve compression were associated with ischemic injury to Schwann cells, resulting in their necrosis and in demyelination. Severe nerve compression was associated with injury to the axon, resulting in wallerian degeneration.
Subsequent experiments established the relationship of pain to these forms of neuropathologic change.60 These studies established that mild levels of ischemia producing demyelination were generally not painful, whereas severe ischemia-producing wallerian degeneration resulted in hyperalgesia. The pathology of the chronic constriction injury model of neuropathic pain is based on this relationship and the added insult of inflammation caused by the chromic gut ligatures used to compress the nerve.61 It is now recognized that the cytokine-driven processes of wallerian degeneration are the dominant neuropathologic factors linking nerve injury and pain60,62,63 and that the degree and extent of wallerian degeneration relate directly to the magnitude and duration of hyperalgesia.64
Biologic and Biochemical Effects on Nerve Roots
The clinical picture of sciatica with a characteristic distribution of pain and nerve dysfunction but in the absence of herniated disc material at radiologic examination and at surgery has indicated that mechanical nerve root compression may not be the only factor that is responsible for sciatic pain. It has been suggested that the disc tissue per se may have some injurious properties.9 Not until 1993 was it confirmed experimentally, however, that local, epidural application of autologous nucleus pulposus with no mechanical deformation induces significant changes in structure and function of the adjacent nerve roots.6
Biologic Effects of Nucleus Pulposus
In 1993, Olmarker and colleagues6 published a study that showed that autologous nucleus pulposus can induce a reduction in nerve conduction velocity and light microscopic structural changes in a pig cauda equina model of nerve root injury. These axonal changes had a focal distribution, however, and the quantity of injured axons was too low to be responsible for the significant neurophysiologic dysfunction observed. A follow-up study of areas of the nerve roots exposed to nucleus pulposus that appeared to be normal by light microscopy revealed that there were significant injuries of Schwann cells with vacuolization and disintegration of Schmidt-Lanterman incisures (Fig. 8–2).65 Schmidt-Lanterman incisures are essential for the normal exchange of ions between the axon and the surrounding tissues. An injury to this structure would be likely to interfere with the normal impulse conduction properties of the axons, although these models’ changes may not fully explain the neurophysiologic dysfunction observed.
The pathophysiologic potential of the nucleus pulposus was emphasized further in an experiment using a dog model in which it was seen that a surgical incision of the anulus fibrosus, with minimal leakage of nucleus pulposus, was enough to induce significant changes in structure and function of the adjacent nerve root.66 It has also been seen that epidural application of the autologous nucleus pulposus within 2 hours induces an intraneural edema67,68 that leads to a reduction of the intraneural blood flow.68 Histologic changes of the nerve roots are present after 3 hours,69 and a subsequent reduction of the nerve conduction velocity starts 3 to 24 hours after application.6,69 The nucleus pulposus may also interfere with the nutrition to the intraspinal nerve tissue. After application to the dorsal root ganglion, it was found that the intraneural blood flow was dramatically decreased and that there was a simultaneous increase of the tissue fluid pressure.68
Methylprednisolone reduces the pathophysiologic events of the nucleus pulposus–induced nerve root injury if given within 24 hours. To establish if the presence of autologous nucleus pulposus could initiate a leukotactic response from the surrounding tissues, a study was initiated that assessed the potential inflammatogenic properties of the nucleus pulposus.70 Autologous nucleus pulposus and autologous retroperitoneal fat were placed in separate perforated titanium chambers and placed subcutaneously, together with a sham chamber, in the pig. The number of leukocytes was assessed 7 days later for the chambers. The nucleus pulposus–containing chambers had a number of leukocytes that exceeded the two others by 150%. In another experiment, autologous nucleus pulposus and muscle were placed in Gore-Tex tubes subcutaneously in rabbits.71 After 2 weeks, there was an accumulation of macrophages and T-helper and T-suppresser cells in the tube with nucleus pulposus that persisted the full observation time of 4 weeks.
Kawakami and colleagues72 showed that neuropathic pain in an experimental setting seems to be mediated by infiltrating leukocytes, a finding consistent with the previous observations of neuroimmunologic inflammatory changes and pain.73 In rats made leukopenic by using nitrogen mustard, the pain response was absent after application of nucleus pulposus, whereas normal rats with nucleus pulposus application displayed a pathologic response to stimulation. The same group also showed that inhibition of cyclooxygenase-2 might reduce nucleus pulposus–induced pain behavior.74 Taken together, these data further support the impression that autologous nucleus pulposus may elicit inflammatory reactions when outside the intervertebral disc space and that such reactions may not be restricted to resorption of the herniated tissue but also may be intimately involved in the pathophysiology of sciatica.
Nucleus Pulposus and Sciatic Pain
Pain is much more difficult to assess than nerve conduction in controlled experimental studies. The available literature indicates that pain may be induced by mechanical factors and nucleus pulposus–mediated factors. The role of the nucleus pulposus in this context is interesting in view of patients with obvious symptoms of disc herniation but with no visible herniation at radiologic examination or surgery.75,76 The potential of nucleus pulposus material to induce pain has also been indicated in clinical studies that showed that noncontained herniations (the nucleus pulposus was in contact with the epidural space) were much more painful and had a more pronounced straight-leg raising test result than contained herniations.77–79
Studies on rats using pain behavior assessment indicated that the nucleus pulposus is involved in pain production. Pain behavior in this context refers to response thresholds to thermal and mechanical stimulation. Kawakami and colleagues52,53 showed that a three-level laminectomy and application of homologous nucleus pulposus or anulus fibrosus taken from three intervertebral discs in another rat, applied at three nerve roots, produces pain behavior. Other studies54 suggest a dose-response relationship between pain behavior and the amount of nucleus pulposus material in the epidural space. The combination of nucleus pulposus herniation and mechanical injury produces pain.54 This observation is consistent with the neuropathologic understanding of pain and the consequences of combined mechanical and inflammatory injury to nerve fibers that are superimposed to increase the number of fibers injured and the corresponding increase in proinflammatory cytokines.63,64 The same pathophysiologic response was observed in a study assessing walking patterns, in which it was seen that only the combination of displacement and disc incision produced detectable changes.56 Also, a pain behavior study assessing changes in spontaneous behavior showed that only the combined action of displacement and disc incision produced changes, whereas displacement or disc incision per se did not produce changes.80
These experimental studies on pain behavior suggest that the presence of nucleus pulposus has sensitized the nerve tissue. Minor compression of peripheral nerves is not painful, and touching of a normal nerve root during local anesthesia is not painful.81 Touching of a nerve root exposed to a disc herniation often reproduces the sciatic pain, however.81 Although the combination of a mechanical component and the presence of nucleus pulposus seems to be a prerequisite to produce changes in the in vivo situation, more recent neurophysiologic studies have shown that the mere application of nucleus pulposus may induce increased neuronal pain transmission.82 This finding reflects that pain behavior assessment is a gross instrument to detect pain and that nucleus pulposus may induce pain in the absence of a mechanical component as well.
The spinal dura mater is known to contain nerve endings, and stimulation of the dura has been suggested as a mechanism for sciatic pain.9,81,83,84 Irritation or stimulation of the dura as one important factor for sciatica is an interesting theory that could explain many clinical features. One may assume that the dura is segmentally innervated, the sensory nerves travel in a caudal-lateral direction, and the dura is drained to the corresponding nerve root by the nerve of Luschka.85–88 Stimulation of the dura at a point where the dorsolateral herniations appear (I in Fig. 8–3) should be recorded by the corresponding nerve root.89 At this location, the irritation may spread medially to the contralateral segment, however, producing bilateral symptoms, or laterally, producing symptoms from levels above. Similarly, a lateral disc herniation (II in Fig. 8–3) could produce symptoms in the lower level.
< div class='tao-gold-member'>
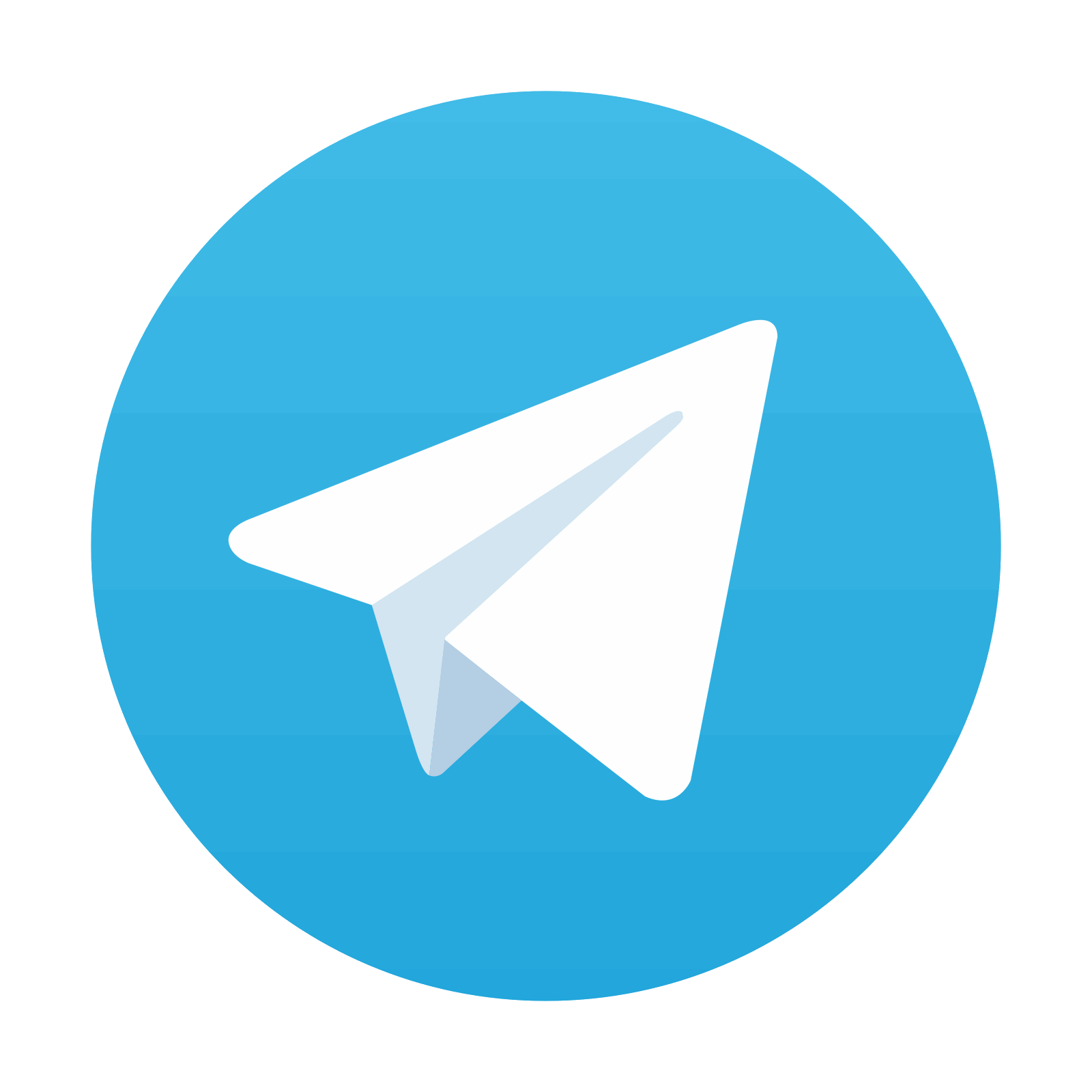
Stay updated, free articles. Join our Telegram channel

Full access? Get Clinical Tree
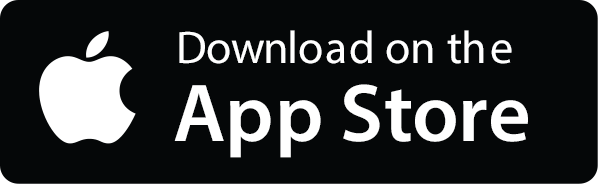
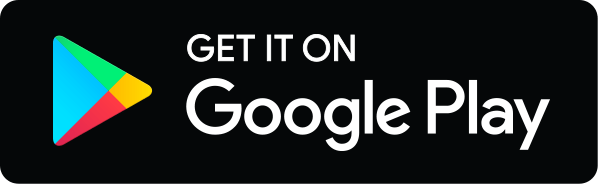