Chapter 50 Robotic devices for rehabilitation of patients with spinal cord injury
The incidence of spinal cord injury (SCI) in industrial countries is approximately 40 new cases per year per million population. In 2004 there were 1,800 new cases in Germany15 and 11,000 in the United States.32 The majority of SCIs are caused by trauma, but an increasing number are caused by nontraumatic injuries. Injuries of the spinal cord lead to variable losses in limb movement and sensation. Loss of function, such as walking, mobility, or hand movement for activities of daily living, creates dramatic changes in the lives of patients. In the past, almost every patient with SCI died within the first 5 years after sustaining the injury. However, increased understanding of the complications related to SCI and improved treatments during rehabilitation have changed the life expectancy of patients.32 Medical complications, if managed well, provide us the opportunity to maximize remaining motor function during rehabilitation using appropriate training methods or technical assistive devices.
Only 30% of patients have complete SCI.32 In patients with incomplete SCI, some sensory and/or weak motor functions are preserved. Eighty percent of patients improve to a higher functional level within the first year after injury. The greatest extent of neurological recovery occurs within the first 6 months after injury and reaches a plateau 12 to 15 months after injury.46
According to this time line, three phases of primary rehabilitation with different therapeutic goals can be defined. The first phase, the very acute stage, begins at the date of injury and lasts for the first few weeks. This phase, which directly follows the lesion, is characterized by immobilization of patients due to cardiopulmonary problems and the consequences of spine stabilization surgery, which is performed in almost all patients in Europe and the United States. The very acute stage is followed by the subacute stage, which is the period from 2 to 6 months after the lesion. During this phase, clinicians and therapists aim at functional improvement of patients using gains in muscle strength resulting from spontaneous neurological recovery. The last stage during primary rehabilitation is the chronic stage. This phase consists of the period from 6 months after the injury and beyond when typically only minor neurological recovery occurs. Within this phase, preserved and permanent functional limitations of patients must be carefully analyzed in order to derive valid recommendations for surgical interventions, such as muscle–tendon transfers, or implementation of technical aids.
Robotics in therapeutic applications
Lower limb rehabilitation
Novel therapeutic methods for rehabilitation of the lower extremities in patients with SCI are based on recent neurophysiological findings. These findings suggest that the spinal cord is more than just a connection between the motor areas of the brain and the muscles of the lower extremities. Experiments performed mainly in cats by Grillner and Wallen16 and in humans by Barbeau and Rossignol2 show that the spinal cord contains functional networks of nerve cells. If adequately stimulated from afferent input sources, this neural network, called the central pattern generator, is capable of producing complex muscle activation sequences in terms of stepping movements. In contrast to spinalized cats, humans with complete SCI do not show locomotion-like intrinsic activity. Even if no autonomous spinal activity is present in patients with complete SCI, some rhythmic activity of spinal origin can be induced. Involuntary stepping movements in patients with incomplete SCI have been reported.6 Dietz et al.9 showed that the spinal cord below the site of lesion in completely paralyzed patients is able to adequately process information from peripheral sensors (forces or joint angles) and to transform this information into a functional muscle activation pattern.
Early mobilization of patients with SCI
If these principles of “spinal intelligence” are incorporated appropriately into the rehabilitation scheme, they can be successfully applied to reduce the initial problems of patients with SCI. During the very acute stage after injury, spinal reflexes are absent in most patients (spinal shock). Venous pooling occurs in the lower extremities, and the circulatory system is unstable. Together these symptoms are the cause for the extended period of immobilization. Standard therapy during this period consists of static verticalization of patients using a tilt table and application of passive movements of the legs by physiotherapists for about 10 minutes once per day. However, bedrest studies have shown that prolonged immobilization leads to quick loss of muscle mass, negative hemodynamic effects, and severe bone demineralization.41
In order to provide the clinical basis for an effective mobilization therapy at this very early stage of rehabilitation, two centers dedicated to the rehabilitation of SCI patients (Orthopedic University Hospital, Heidelberg, Germany and Balgrist University Hospital, Zurich, Switzerland) developed a novel robotic tilt table called the Erigo. This device moves the patient’s legs in a manner comparable to the physiological gait pattern. In contrast to conventional devices that provide continuous passive motion, the Erigo achieves not only appropriate physiological kinematics in terms of adequate hip extension but also kinetics (loading/unloading of the foot sole) that are close to a normal gait. The importance of these factors as triggers for the central pattern generator has been shown in experiments in spinalized cats11 and in humans.10 A main design criterion of the device was the possibility of increasing the stepping frequency to a value comparable to that of normal walking.
Erigo device
The Erigo is based on a traditional tilt table (Fig. 50-1). The upper body part can be continuously tilted from the supine position up to 80 degrees. When the patient is lying on the tilt table, the patient’s upper body is secured through a harness, with chest and shoulder fixed. Each thigh is fastened by a cuff to a linear drive mechanism that is controlled by a microcomputer. In order to generate hip extension according to the physiological principles, the upper body part of the device also can be tilted downward up to 20 degrees in relation to the leg part. The foot is fixed by a strap onto a proprietary footplate. For physiological loading of the foot, a special spring damper mechanism is integrated into the footplate. Load is applied to the foot sole during the stance phase (hip and knee extension) by clamping of a spring beneath the plate. When the hip and the knee are flexed, the spring is released from the plate, and no load is generated.8 The overall load on the foot increases with the degree of tilting, as a greater percentage of the patient’s body weight counteracts with the spring-damped footplate.
Clinical results of the Erigo
To prove the hypothesis that this device leads to stabilization of the cardiovascular system, we initiated a clinical pilot study where we included six acutely injured patients with clinically complete injury of the cervical spinal cord (ASIA impairment scale A, lower extremity motor score 0) 4 weeks after injury and analyzed relative changes in blood pressure compared to baseline measurements recorded with patients in the initial resting position.8 Baseline measurements were recorded in the horizontal position because patients could not tolerate 10 minutes of upright standing position without signs of presyncope, especially at the onset of study participation. With the automated movement therapy, blood pressure increased up to 5% even though patients were tilted to the 60-degree upright position after the baseline interval (Fig. 50-2). After stopping the movement in this position, mean arterial pressure decreased significantly (−30%), thus showing the direct effect of passive movement on the circulatory system in these patients.8 From these results, this robotic stepping device seems to help reduce the negative side effects that occur during the early immobilization phase and shorten the time to functional training in a wheelchair. The Erigo device is commercially available (Hocoma, Volketswil, Switzerland).
Plasticity of neural structures
Experiments with spinalized cats with complete transection of the spinal cord in the thoracic region have shown the occurrence of training-induced reorganization of neural networks in the spinal cord. Lovely et al.26 and Edgerton et al.12 demonstrated that a spinalized cat that undergoes locomotor training walks much better than a cat without training. However, when locomotor training was stopped, the cat’s walking function deteriorated. This finding leads to the conclusion that the structure of the neural network responsible for movement generation is highly flexible and that it can adapt to changes caused by injuries to the central nervous system. The described phenomenon is called training-induced neural plasticity. The plasticity is based on feedback of afferent signals coming from force and joint position sensors and increased afferent input to the spinal cord when a dedicated functional task, such as walking, is performed. The increased stimulation results in reorganization of axonal connections and leads to improvement of function.
Treadmill training with partial body weight support
The traditional method for mobilization of patients with restrictions in gait function during the subacute phase of rehabilitation is the use of parallel bars, where patients strut themselves on the bars with their arms and thereby are able to walk a few steps. This kind of mobilization is considered ineffective with respect to the principles of training-induced plasticity. Repetition of a dedicated movement leads to accelerated skill acquisition of a motor function.13 This is the main reason why locomotor training on the treadmill has been established as a standard therapy for rehabilitation of neuromuscular gait disorders over the last 10 years.18,47 Whereas patients can walk only a dozen steps in parallel bars, they can walk up to 20 times the distance on a treadmill. During treadmill training, patients are first secured in a harness and then attached to a counterweight, which ensures constant body weight support during all gait phases (Fig. 50-3). Initial support up to 60% of the body weight can be used; higher percentages appear to be less effective because of insufficient loading of the foot soles. The initial slow walking speed, which is chosen by the patient, is increased during the training course up to a normal walking speed.
Robot-assisted locomotion therapy
To overcome these limitations of manually assisted treadmill training, Colombo et al.7 (ParaCare, University Hospital Balgrist, Zurich, Switzerland) developed the Lokomat motor-driven gait orthosis, which is commercially available in combination with a treadmill. The Lokomat consists of a steel frame, which can be adjusted to the patient’s body properties, and a motorized bilateral hip and knee joint (Fig. 50-4). The Lokomat is fixed to the patient’s thigh and shank by three cuffs of different sizes made of hook-and-loop material (Fig. 50-5). Joint movements are generated by linear spindles at walking speeds comparable to a normal gait (up to 3.5 km/h). With implementation of a recently released adaptive impedance control algorithm, the Lokomat can precisely produce the amount of force necessary to support the patient’s preserved motor functions for achievement of a physiological gait pattern.35
A multicenter study of 20 chronic, incomplete SCI patients (injury for more than 2 years, stable neurological status) performed at four SCI centers (Schweizerisches Paraplegikerzentrum, Basel, Switzerland; Department of Physical Medicine and Rehabilitation, University of Illinois, Chicago, Illinois; Orthopaedic University Hospital, Heidelberg, Germany; and Balgrist University Hospital, Zurich, Switzerland) showed positive effects of a 4-week Lokomat training program (45-minute training session, 3–5 times per week). Patients who were able to walk with walking aids at the beginning of the training were able to walk almost two times faster and two times further after training. However, only two of the 20 patients were able to achieve significantly reduced dependency on their walking aids (walkers, crutches).48
Another robotic device, a commercially available gait trainer developed by Hesse group (Berlin, Germany), has shown beneficial effects in patients with stroke and in a few patients with subacute incomplete SCI.19 However, our experience with the device revealed handling problems when training SCI patients with severe limitations of body and lower limb posture control. The basic design principle of the gait trainer, which generates walking movements only by motor-driven positioning of the foot, does not provide sufficient stabilization of the trunk and the legs and seems to be less effective in terms of physiological afferent stimulation of the spinal pattern generator.
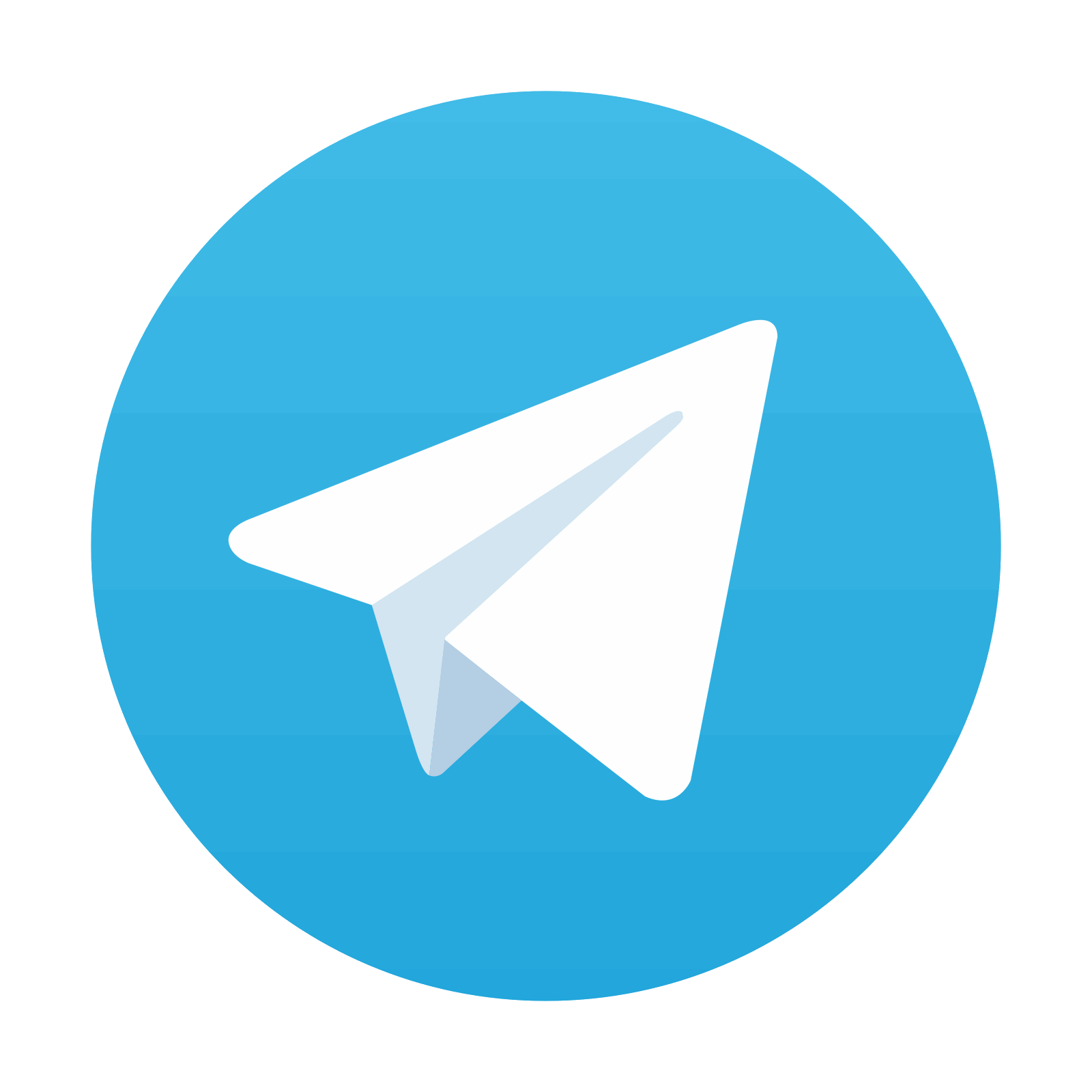
Stay updated, free articles. Join our Telegram channel

Full access? Get Clinical Tree
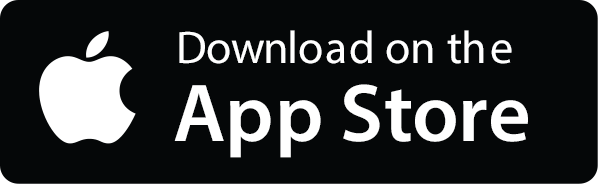
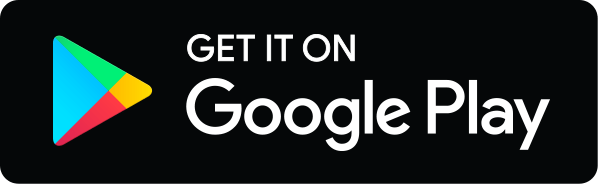