Understanding progressive rehabilitation, assessment and observation
On initial observation of an injury it is essential to perform an injury screening (refer to Chapter 2 for a detailed overview of screening procedures). SINS (severity, irritability, nature and stage) and SOAP notes (subjective, objective, assessment and plan) are two acronyms that provide an effective method of gathering information relating to possible trauma (Sleszynski et al. 1999). Table 12.1 describes the notation and how these acronyms are used to organisation injury information and assessment.
Table 12.1 Overview of SINS and SOAP notation, adapted from (Borcherding and Morreale 2007)
Components of rehabilitation including flexibility, the restoration of muscular strength and endurance and enhancing proprioceptive control are concepts that must be managed effectively to ensure optimal injury management (Beam 2002), within the context of physical activity and return to sport. Systematic, functional and progressive rehabilitation involves the process of carefully considering the key components of musculoskeletal trauma. This involves adequate management of the injury in order to prevent further soft tissue trauma and enable a progressive treatment protocol to be implemented effectively. This is of particular importance, as previous history of injury can lead directly to an increased risk of trauma following the implementation of a rehabilitation programme (O’Sullivan et al. 2009).
Goal planning is key to the successful implementation of a progressive rehabilitation programme. For example, if an athlete has excessive valgus movement of the knee during a single leg functional activity, it is the role of the rehabilitator to provide effective stabilisation and strengthening exercises for the gluteal muscles in order to provide the lumbo-pelvic hip complex with the stability it requires to maximise total power output throughout the entire kinetic chain (Liebenson 2006; Boudreau et al. 2009). However, during the rehabilitative process it is imperative to ensure strengthening is not focused solely on a specific muscle, this is because during closed kinetic chain exercises, which simulate functional movement, it is inaccurate to make judgement stating a specific muscle is responsible for poor neuromuscular control. Previous research linked to isokinetic muscle testing, which isolates a target muscle, has stated hip musculature is responsible for optimal functional single leg activity; however, fatigue does not occur in a single muscle group when performing closed kinetic chain exercises, which simulate movement patterns more likely to be carried out during everyday actives and athletic performance (Reimer and Wikstrom 2009). Similarly, deficits in muscular strength have been shown to increase the risk of injury. Hollman et al. (2006) suggest that reduced isometric strength of the hip abductors in relation to the hip adductors is associated with malalignment of the lower extremity such as foot pronation and knee valgus. This may result in inadequate neuromuscular control during functional movement increasing the risk of anterior cruciate ligament injury (ACL) (Hewett et al. 2006). Therefore, when prescribing exercises during the rehabilitation of an athlete, it is important to take into account not only strengthening of the effected region of the body, but also to continue strengthening the entire extremity, which can prevent muscle imbalances and potential injury concerns.
It is the synthesis of knowledge coupled with clinical practice that best defines how and when to either progress or regress exercise patterns and routines.
It would be useful to read the chapters on Clinical Reasoning (Chapter 16) and An Introduction to Periodisation (Chapter 9) along with this chapter to better aid understanding of exercise design and decision-making processes.
Consideration and application of the concept of continual progression is useful as it provides the clinician with the ability to justify why specific exercises have been prescribed and how best to regress or progress the exercises depending on the athlete’s development through the rehabilitation process. During the final stages of a rehabilitation programme it is important that the athlete performs exercises within their functional range, this involves performing exercises that initiate abnormal motor control and allow minimal mechanical sensitivity (Liebenson 2006). By ensuring exercises are performed within a functional range, this limits the possibility of re-injury (Stracciolini et al. 2007). In addition to introducing the athlete to functional exercise it is important to ensure that movements simulate the patterns of movement the athlete will eventually perform in their sport, this needs to include the relevant muscle actions (concentric, eccentric, isometric), velocity of movement, force generation, power output and rate of force development.
Muscle actions that relate to effective movement patterns in the athlete’s sport must be implemented during final stages of rehabilitation. For example, the hamstring muscle complex is a commonly injured region of the body in relation to sports injury (Brockett et al. 2004; Hoskins and Pollard 2005; Askling et al. 2006; Verrall et al. 2006). It is important, however, not to generalise when assessing and initiating injury management for this muscle complex (see the example in Chapter 4, Pathophysiology of Skeletal Muscle Injuries). The demands of various sports often lead to trauma developing in different regions of the hamstring complex. For instance, in sprinters it has been shown that injury consistently occurred in the long head of the biceps femoris; in contrast dancers suffered common trauma at the proximal tendon of the semimembranosus. Return to pre-injury level was shown to be shorter for high-speed trauma as experienced by the sprinters, in contrast to trauma resulting from overstretching from which the dance group demonstrated (Askling et al. 2008). This evidence provides information that shows rehabilitation from hamstring trauma particularly, should consider both the type of sport in which the athlete participates and the common movement patterns in preparation for implementation of a sports-specific functional rehabilitation programme designed to prevent re-injury. This protocol should apply directly to any injury, to ensure successful management and long-term protection against chronic and ongoing injury concerns.
Inflammation and pain management
Liebenson (2006) found that the application of an early stage rehabilitation programme with restoring full pain-free functional range of motion as a main objective, is beneficial and can result in a rapid attainment of pre-set functional tasks. Table 12.2 below details how for example cryotherapy can be used in the acute management of soft tissue injuries.
Table 12.2 Summary and suggestions from primary literature for the use of cryotherapy, compression and elevation in the early stages of acute management of a soft tissue injury
MacAuley (2001) and Bleakly et al. (2006) suggest that intermittent application of ice may enhance the therapeutic effect of ice in pain relief after acute soft tissue injury. They prescribe the following protocol: “10 minutes ice/water submersion (approx 0°) on 10 minutes off (room temperature) 10 minutes on ice/water submersion (approx 0°)”. The submersion may also help with increased pressure and in turn mimic compression effects. The sport rehabilitator is advised to adhere to prescribed guidelines for the use of cryotherapy within progressive injury rehabilitation. These could include the responsible use of cryotherapy, to prevent ice burns that may be caused by direct application or prolonged exposure to cryotherapy. The use of a wet cotton towel similar to that of a dish cloth may act as a suitable barrier between ice and skin (Bleakly et al. 2007). Early intervention of the use of cryotherapy has also been suggested to help speed recovery and return to sport (Hubbard and Denegar 2004), therefore highlighting the importance of early cryotherapy use within the management of an injury.
According to Hubbard and Denegar (2004), this field of applied study and practice would benefit from evidence-based research into the use and barriers of cryotherapy application. This has further been supported by Collins (2008), who claims that the evidence behind cryotherapy is insufficient to the outcomes to improve the outcomes of clinical management of soft tissue injuries.
Range of motion and flexibility
Range of motion (ROM) and flexibility are central to rehabilitation and used not only as markers of assessment, but more importantly as techniques for rehabilitation practices (Small et al. 2008). ROM testing is an essential component of athlete evaluation and provides the practitioner with the acquired information including active and passive ROM, contractile ability of the musculature and occasionally severity of injury (Stracciolini et al. 2007). This can then be used to develop a specific stretching programme with the goal to enable the athlete restore full ROM to allow progression through the appropriate rehabilitative exercises and eventually to return to full sports participation (Beam 2002).
It is important to ensure that prior to progressing rehabilitative exercises and return sport the athlete has sufficient ROM at the joint enabling full capacity of movement to perform the exercises and to perform the sport-specific movements. The athlete must have pain-free active ROM in the frontal, saggital and transverse planes when performing basic movements and have the ability to contract the affected muscle in synergy with the antagonist muscles (Liebenson 2006). Only after an athlete can carry out these movements can a sport specific, functional rehabilitation programme be implemented (Small et al. 2008).
During an initial musculoskeletal evaluation the clinician may have to take the athlete through active and passive range of motion. Active range of motion is when the athlete moves the affected joint through its range without any external influence. This is done as a measure of pain through a functional range and also provides the sports rehabilitator with the information about whether to perform a passive range of motion assessment at that joint (Jarvinen et al. 2007). Passive range of motion occurs when the clinician takes the affected limb through its range in order to provide information regarding the integrity of joint or ‘end feel’. This usually provides important information regarding the client’s condition and informs the clinician about future action, assessment and intervention. In considering normal joint range of motion, resultant injury risk factors and how to relate the importance of full functional range of motion to the athlete’s movement patterns in their sport, the clinician can appreciate the relationship between the latter stages of the rehabilitation process and the return of an athlete to a competitive environment (Pizzari et al. 2008). The athlete should not return to full activity until full pain-free functional range has been established (Stracciolini et al. 2007). As flexibility is an expression of range of motion, knowing when and how to incorporate flexibility training into range of motion rehabilitation is important. The clinician must also be able to determine the effectiveness of flexibility training in relation to final stage rehabilitation and the athlete’s sport. It is more beneficial to fully and accurately assess movement dynamics in relation to the common muscular movement patterns and then decide whether or not increased range of motion is beneficial to improving the performance of that athlete (Jarvinen et al. 2007).
Flexibility training
The aim of a flexibility programme should be to achieve and maintain an optimum ROM at each joint that is specific to the athletes sport.
Three modalities that are universally employed during a flexibility programme to obtain an increase in joint ROM are static stretching, proprioceptive neuromuscular facilitation (PNF) (Roberts and Wilson 1999; Babault et al. 2009) and self myofascial release (SMR) (Curran et al. 2009)
Static stretching
Static stretching involves taking the limb to a position that produces increases in muscular tension and allows elongation of a muscle with the aim of enhancing tissue extensibility. The proposed neurophysiological process by which this occurs is termed autogenic inhibition (Olivo and Magee 2006). This is performed in a slow and controlled manner (consequently the possibility of exciting the muscle spindles and inducing a stretch reflex is minimal) and held for ≥30 seconds (Bacurau et al. 2009; Yuktasir and Kaya 2009) and repeated 3–4 times (Costa et al. 2009; Yuktasir and Kaya 2009). As a result of the prolonged period under increased muscular tension the golgi tendon organs (GTOs) may be stimulated and allow for autogenic inhibition to occur, thereby resulting in a decrease in tension and therefore lengthening of the agonist which enables a prolonged and increased stretch of the muscles (Olivo and Magee 2006).
Figure 12.2 Rehabilitation progressions including sport specific progression. (Adapted from: Beam (2002) Rehabilitation including sport specific functional progression for the competitive athlete. Journal of Bodywork and Movement Therapies 6 (4), 205–219).
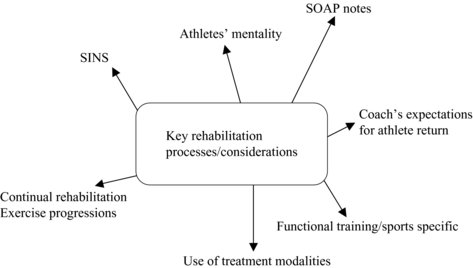
Figure 12.3 showa an example of a static stretch for the gastrocnemius. To initiate the stretch follow these guidelines:
1. facing a wall, place hands out in front of the body
2. place one foot in front of the other ensuring heels stay in contact with the ground
3. maintaining an upright posture, push forwards from the hips keeping the head upright and facing forwards until a stretch is felt in the gastrocnemius of the back leg.
Passive stretching (at the end of available range) should be avoided for the first 72 hours as a minimal period and possibly for the first 7–10 days following injury if more severe (Neidlinger-Wilke et al. 2002). The reasons for this is that healing tissue is weak and intolerant to tensile loading and is likely to be damaged by uncontrolled stretching. Prior to the 10th day post injury it would be more appropriate to take the muscle through its full available pain free range without any attempt to force the muscle beyond this point.
Once it is appropriate to begin stretching the muscle, that is, elongating the tissue beyond its available range then careful passive stretching can be performed. Each stretch should be held at the end of available range within the limits of pain. Time, frequency, duration and intensity of stretch remain debateable in the literature. Some research suggests passive stretching should be held for a minimum of 15 seconds with 6–8 sets per day (Roberts and Wilson 1999), however, Bandy et al. (1994, 1997) reviewed stretches at 15 seconds, 30 seconds and 60 seconds. They determined that 30 seconds was the optimum duration (ideally repeated 3 times and performed 3 times per day), also stating that longer periods after 30 seconds was ineffective in promoting additional stretch.
As with strengthening exercises stretching exercises need to be progressed in order that the tissue adapts to the different types of load once the athlete is comfortable (pain free) with passive stretching (Bandy et al. 1994, 1997).
Although implementing a stretching protocol may elicit improvements in performance as a result of increased ROM at a joint (Herda et al. 2008), research has suggested the use of static stretching may have a detrimental effect on performance, immediately post stretching, in relation to sprinting, strength endurance and jumping, all power based activities (Bacurau et al. 2009; O’Sullivan et al. 2009; Hough et al. 2009). PNF has also been linked to a decrease in power production immediately post stretching (Mareket al. 2005). Therefore it is essential that ROM is restored as early in the rehabilitation process as possible to ensure that this does not have a detrimental effect on subsequent strength or power performance.
Proprioceptive neuromuscular facilitation
Proprioceptive neuromuscular facilitation is a form of muscle energy technique (MET) performed passively with a partner involving a voluntary isometric contraction followed by a static stretch phase (Smith and Fryer 2008). There are two main PNF techniques; contract-relax and contract relax-antagonist contract techniques (Olivo and Magee 2006).
The contract relax method requires a limb to be move in to a stretched position and then the agonist undergoes an isometric contraction for 7–10 seconds. As the limb does not move, but the muscle contracts and therefore shortens, the change in length is accommodated by a lengthening of the tendons. This lengthening of the tendon stimulated the GTO and thereby inhibits the agonist. Once the isometric contraction ends the ROM can be increased and the procedure repeated.
The contract relax-antagonist contract technique has been suggested as the most effective in increasing muscle length due to the concept of reciprocol inhibition, which produces increased suppression of the motor pool (Etnyre and Abraham 1986). Reciprocal inhibition is the relaxation that occurs in the opposing muscle that is experiencing increased muscular tension (Olivo and Magee 2006). This is performed with a voluntary isometric contraction of the desired muscle to be stretched lasting approximately 7–10 seconds at a range of 25–40% of maximal voluntary contraction (MVC) followed by an antagonist assisted static stretch phase (Olivo and Magee 2006; Smith and Fryer 2008). The incorporation of a passive stretch supported by the antagonist is believed to produce greater joint flexibility than static stretching (Sady et al. 1982; Guissard 1988; Magnusson et al., 1996; Handel et al. 1997) and produce the longest duration of maintained flexibility than any other form of stretching (Spernoga 2001).
Figure 12.4 illustrates how to perform hamstring PNF, along with the guidelines for correct implementation.
1. The leg is taken to the initial tissue barrier, with the opposing leg straight along the floor. Ensure your hand position is not over the joint.
2. Hold for initial period, stretching the raised leg.
3. Induce an isometric contraction from the athlete in the hamstring musculature.
4. Rest the hamstring muscles and induce an isometric contraction of the antagonist muscles (quadriceps).
5. Rest and repeat the process.
6. Follow the guidelines as stated above for advised muscle contraction force and length of time contractions should be held.
Self myofascial release (SMR)
Self myofascial release (SMR) is a soft tissue technique centred on enhancing ROM through the breakdown of myofascial restrictions in the body’s fascial system (Curran et al. 2009). Fascia is a three-dimensional web of connective tissue that envelopes the body’s soft tissue from head to toe providing stability and flexibility (Barnes 1997; Myers 1997). SMR is performed by slowly applying a force with the use of a foam roller to tender spots of the muscle (Curran et al. 2009), which are indicative of fascially restricted areas termed “trigger points” (Travell and Simons 1992; Simons et al. 1998). The force is maintained for a time period ranging from 20 to 90 seconds or until a significant reduction in discomfort is attained. This encourages the transformation of a ‘knotted’ hypertoned bundle of fibrous tissue to a more pliable tissue that is parellel in formation to the fascia (Travell and Simons 1992; Simons et al., 1998). The subsequent increased ROM achieved using SMR has been attributed to the neurophysiological effects on the fascia (Johansson 1962; Schleip 2003) and histological changes in the fascias cellular content (Cantu and Grodin 2001; Sefton 2004; Barnes 2005).
When attempting to enhance ROM at a joint with flexibility training it is important to not only address the muscles that are displaying reduced extensibility but also the synergistic muscles that are underactive or weak and therefore reducing active ROM. In this case, strengthening at the ends of the ROM is required.
Proprioception/neuromuscular control
As suggested by Myer et al. (2005) neuromuscular control training is important to the improvements of athletic performance and biomechanical stability, which in turn reduces the risk of injury.
Proprioception is a term used frequently during rehabilitation and can be defined as a specialised variation of the sensory system of touch that encompasses the sensation of joint movement (kinesthesia) and joint position (joint position sense) (Lephart et al. 1997). These signals are transmitted to the spinal cord via afferent (sensory) pathways (Prentice 2004). The term refers specifically to conscious and subconscious appreciation of a joint potion in space. The efferent (motor) response to sensory information is termed neuromuscular control (Jonsson et al. 1989). Two motor control mechanisms are involved with interpreting afferent information and coordinating efferent responses (Dunn et al. 1986; Prentice 2004), feed-forwards and feedback.
Feed-forward neuromuscular control involves planning movements based on sensory information from past experience (La Coix 1981; Dunn et al. 1986). Feedback process continuously regulates muscle activity through reflex pathways. Feed-forward mechanisms are for preparatory muscle activity; feedback processes are associated with reactive muscle activity. The level of muscle activation, whether it is preparatory or reactive, greatly modifies its stiffness properties (Prentice 2004). From a mechanical perspective, muscle stiffness is the ratio in the change in force to change in length (Dietz et al. 1981; Bach et al. 1983; Dyhre-Poulsen et al. 1991). Muscles that are stiffer resist stretching more effectively and provide more effective dynamic restraint to joint displacement (Bach et al. 1983; Mc Nair et al. 1992); for example, in an ACL deficient knee the increase in hamstring muscle activation increases hamstring stiffness and therefore the functional ability of the knee to reduce anterior translation (Bach et al. 1983; Mc Nair et al. 1992; Myer et al. 2005). The dynamic restraint system is mediated by specialised nerve endings called mechanoreceptors (Grigg 1994), which function by transducing mechanical deformation of tissue into modulated neural signals (Grigg 1994).
Increase tissue deformation is coded by increase afferent discharge rate or increase in mechanoreceptors activated (Grigg 1994; Prentice 2004). The signals provide sensory information concerning internal and external forces action on a joint. Mechanoreceptors (Pacinian corpuscles, Meissner corpuscles and free nerve endings) can be classified as either quick adapting (QA) or slow adapting (SA). Quick adapting receptors cease discharging shortly after the onset of a stimulus, whereas SA continue to discharge as long as the stimulus is present (Clark and Burgess 1975; Schultz et al. 1984; Katonis et al. 1991; Grigg 1994).
In a healthy joint these QA mechanoreceptors are believed to provide conscious and subconscious kinaesthetic sensation in response to a joint movement or acceleration, whilst SA mechanoreceptors provide continuous feedback (Freeman and Wyke 1966; Clark and Burgess 1975) and therefore proprioceptive information relative to the joint position (Freeman and Wyke 1966; Clark and Burgess 1975; Schultz et al., 1984; Katonis et al., 1991; Grigg 1994).
The pre-activation theory suggests that prior sensory feedback (experience) concerning the task is utilised to pre-programme muscle activity patterns. Rehabilitation programmes should be designed to include a proprioceptive component that addresses the following three levels of motor control: spinal reflexes, cognitive programming, and brainstem activity. Such a programme is highly recommended to promote dynamic joint and functional stability (Lephart et al. 1997). There are different proprioceptive states that are important to consider in the design of a progressive rehabilitation programme.
Static proprioceptive development involves exercises with the maintenance of a stable base, while allowing for only minimal movement. During this stage of neuromuscular development the athlete should focus on control of posture and be able to perform a number of modifications of static proprioceptive training before progressing on to a dynamic, more functional proprioceptive training programme (Liebenson 2006).
Dynamic proprioceptive training should only be introduced when the athlete has demonstrated a sufficient level of balance and coordination during the static proprioceptive exercise phase of the neuromuscular control program (Beam 2002). Since dynamic proprioceptive exercises involve greater levels of instability and require a higher demand for accuracy, strength and speed of motion (Myer et al. 2006), balance and coordination are important to ensure the athlete can progress safely without hindering their development. A simple objective test to determine the athlete’s progression from static to dynamic exercises can include the Romberg test which assesses the ability to balance. The athlete stands with their feet together and eyes closed (Thuan-Lee and Kapoula 2007). A more advanced test is the stork stand, which involves the athlete standing on one leg usually the effected limb and maintaining the position for a period of at least 30 seconds without touching the floor with the opposing leg or supporting themselves with their other limbs (Melorose et al. 2007). This can then be progressed by being performed with eyes shut. If the athlete finds these tests demanding and cannot perform them effectively there is a deficit in balance that needs to be addressed before progressing onto dynamic exercises.
Table 12.3 shows an example of four different balance tests that could be integrated into all stages of a functional rehabilitation programme to enhance proprioceptive responses and neural drive to the working muscles and aid in the athlete’s development. This example can be implemented as soon as the athlete is able to weight bear on both legs. Once this capability is obtained, the exercises including taking the individual from a standing position to a countermovement jump can be progressed or regressed depending on the ability of the athlete to perform each exercise without excessive biomechanical dysfunction.
Table 12.3 Balance tests
Continual adaptation to sports specific, functional balance training is fundamental to enhancing an athlete’s proprioceptive awareness. As a result of proprioceptive training, receptors in soft tissue structures and joint complexes are trained to perform coherent actions, able to initiate dynamic, functional movements (Komi 2003).
Dynamic proprioceptive exercises are performed to enhance the ability of the muscles around the affected joint to control joint motion and stabilise the body during movements in multi-planar directions (Myer et al. 2006; Stracciolini et al., 2007; Pasanen et al 2008; Subasi et al. 2008). When an athlete is performing dynamic neuromuscular control exercises to a high level, it is the practitioner who must continue to progress the exercises, in terms of either intensity or difficulty as required, in order to prevent a plateau development.
For further reading and applied examples regarding specific neuromuscular rehabilitation for selected joints refer to the chapters on the knee (Chapter 21) and ankle (Chapter 22), in the latter section of this book.
By continuing to enhance the athletes neuromuscular development the clinician is allowing for adaptive changes to occur within the neuromuscular system. This can lead to increases in the ability of an athlete to perform plyometric based exercises, increased levels of balance and improve leg co-ordination in sprint drills (Cameron et al. 2007). Therefore, appropriate progressions during neuromuscular control training will prepare the athlete to progress their rehabilitation on to effective plyometric training.
To implement an effective neuromuscular control programme the clinician should have an expansive knowledge of the anatomical structures and entire kinetic chain. Knowledge of the specific demands and common mechanisms of injury associated with the athlete’s sport will also aid in reducing the risk of injury and preventing the re-occurrence of a previous trauma.
The clinician should be able to effectively adapt a neuromuscular programme without the risk of the onset and formation of muscle imbalances, which in turn may lead to musculoskeletal dysfunction and subsequent injury.
For example, decreased neuromuscular control of the trunk can increase the risk of knee injury, specifically anterior cruciate ligament (ACL) injury (Zazulak et al. 2007). The reason for this being that athletes with reductions in neuromuscular control are more susceptible to increased valgus moments of the knee during the impact phase of jump and landing activities, increasing the risk of ACL injury (Hewett et al. 2005).
The altered neuromuscular activation of the hamstrings and quadriceps muscles are believed to play an important role in this risk of ACL injury. Since the hamstrings insert onto the posterior aspect of the tibia and fibular head, they provide a posterior draw force on the knee that resist anterior tibial forces (Bryant et al. 2008). However, if there are deficits in muscle activation of the hamstrings there is a limited ability to protect the knee ligaments (Hewett et al. 2006). Consequently, over-dominant quadriceps activation as a result of under active hamstrings is believed to produce anterior displacement of the tibia, increasing the risk of ACL injury (Griffin et al. 2006).
This is a commonly neglected stage of knee rehabilitation that is of particular importance since ACL injury is one of the most common causes of reduced sport participation and is associated with recurrent injury and an increased risk of osteoarthritis of the knee (Walden et al. 2006).
Another example is the shoulder joint, which allows the greatest ROM of all the joints (Wassinger et al. 2007), but as a consequence compromises its stability. As a result, in order to maintain optimal dynamic stability, effective proprioceptive input is necessary to ensure neuromuscular deficits do not impair the athletes’ functional ability (Wassinger et al. 2007).
This is achieved by enhancing sensory motor control and ability of the muscles to respond to a neural stimulus (Roig-Pull and Ranson 2007).
In addition to shoulder instability, lateral ankle sprains are a common injury within the sporting arena, which has lead to research being conducted to understand the most effective interventions in relation to prevention and treatment by means of balance and co-ordination training (McKeon and Hertel 2008). The cause of functional instability of the ankle is likely due to altered neuromuscular feedback, which changes the neuromuscular response within the appropriate musculature (Coughlan and Caulfield 2007).
Therefore, great emphasis should be placed on incorporating neuromuscular control/proprioceptive exercises in the athletes’ rehabilitation programme in order to restore and improve balance, coordination and agility thus increasing functional ability of the effected limb and the entire kinetic chain (Subasi et al. 2008). Non-injured athletes can also perform neuromuscular control training during their weekly routine training sessions as part of a injury prevention training programme (Paterno et al. 2004; Pasanen et al. 2008)
Basic concepts of application
As with all stages of injury rehabilitation and the planning of exercise conditioning, the development of a neuromuscular control programme too is also dependant on many factors including gender, injury status, type of sport and level of competition. Since the aim of a proprioceptive training programme is to promote balance, coordination and agility accordingly (Subasi et al. 2008), the athlete must begin first with static exercises before progressing to dynamic exercises. The exercises must develop from simple to complex with emphasis placed on precision, accuracy and control. The clinician can alter a range of variables in order to progress proprioceptive exercises. This can involve changing the rate of speed, amount of simultaneous activities performed at one time, limiting the amount of sight during training and adapting exercises that are more functional with sport specific movements (Risberg et al. 2001)
This is important as adapting the proprioceptive training directly to the neuromuscular response of the athlete throughout the rehabilitation process will simulate similar motor patterns in accordance to the athletes sport. Consequently it has been shown that this may not only enhance performance potential in a competitive sporting environment, but further play a significant role in injury prevention (Hewett et al. 2005; Chappell and Limpisvasti 2008; McKeon and Hertel 2008; Twomey et al. 2008; Zazulak et al. 2007).
It may also be more beneficial to the athlete to perform proprioceptive exercises during the beginning of a rehabilitation session or before a training routine, particularly if the proprioceptive exercises are new to the athlete or are part of more advanced progressions. The reason for this is that it has been shown that exercise induces fatigue on the mechanoreceptors that are situated in the musculature surrounding the joint (McLean et al. 2007). Since mechanoreceptors are responsible for their input of proprioceptive feedback, proprioception is affected along with neuromuscular control and therefore negatively impacts the athletes’ ability to perform the proprioception exercises (Myers et al. 1999).
Practical implementation of unstable surface training
The importance of unstable surface training (UST) has been shown to be beneficial in enhancing proprioceptive input, enabling neuromuscular adaptations to commence during the early stage rehabilitation process (Cressey et al. 2007). This form of training has been commonly implemented worldwide with a wide range of products. However, the key consideration is whether this form of training should continue throughout the athlete’s rehabilitation. In the latter stages when optimisation of force production and power output are required an unstable surface will result in a decrease in force and power output, as illustrated from the results of Cressey et al. 2007).
The majority of sporting movements in the upper extremity occur in an open chain manner; therefore it may be more beneficial to incorporate unstable surface based exercises under these circumstances, rather than in the lower extremity. Most athletic movements in the lower limb occur in a closed chain fashion, in which unstable surface training may prove detrimental to performance in relation to reduced power output. The potential decrease in power and strength capacity is due to elevated activation of the antagonist muscle in relation to the prime move or agonist, which although can assist in maintaining joint stability can compromise complete activation of the functional agonist. Reciprocal inhibition is a physiological response to UST leading to the resulting decrease in force production.
Table 12.4 shows some practical examples of UST and its comparison to stable surface training. Each of the tests demonstrated show movements that are commonly used within the sporting environment; these include sprinting, jumping and quick changes of direction.
Table 12.4 Examples of UST compared with stable surface training (adapted from Cressey et al. 2007)
Table 12.4 demonstrates that the implementation of a UST programme can negatively impact the performance of subjects in relation to power and speed-based activities.
Test summary
Jumping assessments
The stable group showed significantly greater results than the unstable group in relation to the bounce drop jumps (BDJ) and counter-movement jumps (CMJ). The UST group did not shown significant improvements in performance following the intervention at post testing. This is in contrast to the stable training group which showed significant improvements.
Sprinting assessments
The stable training group demonstrated improvement in results, in comparison with the unstable group. Pre-test results were similar for both groups in both sprint activities; however the stable group showed greater advances in performance in contrast to the unstable training group. See Table 12.4 for the exact figures.
Agility assessment
There were insignificant differences between the two groups prior to the training interventions. Both the stable training group and UST group demonstrated advances in performance in relation to the pre-test, following the training intervention. See Table 12.4 for the data collated from the T-Test drill.
Summary
Continual adaptation to sports specific, functional balance training is fundamental to enhancing an athlete’s proprioceptive awareness. As a result of proprioceptive training, receptors in soft tissue structures and joint complexes are trained to perform coherent actions, able to initiate dynamic, functional movements (Komi 2003). Futhermore by creating a more advanced understanding of postural control requirements amongst athletes participating in different sports, the practitioner may be able to prescribe the most effective exercises in relation to the balance and co-ordination demands of the specific sport, enabling more optimal movement for the athlete (Bressel et al. 2007).
The question of when best to implement a dynamic proprioceptive programme remains controversial. Research has yet to fully support arguments surrounding pre versus post training sessions. The important factor to consider here is the influence of continuous eccentric muscle contractions on performance caused from over exertion (Lavender and Nosaka 2007). Intermittent high-intensity eccentric training causes micro-trauma within muscle fibres (Clark et al. 2005). The breakdown of fibres within the skeletal muscle causes slight alterations in the ability of proprioceptive responses to interact effectively with the central nervous system to initiate effective movement patterns in multi-directional planes of motion.
Strength endurance and maximal strength
To initiate a systematic rehabilitation approach through the strength phase it is important to understand the reasons behind why a progressive approach is demanded and how the benefits of strength training can relate to improved performance for an athlete on their return to sport.
It is imperative to ensure athletes progress through the rehabilitation process establishing an adequate level of muscular endurance and then strength (Jarvinen et al. 2007) as required by their individual sport. Isometric strength training needs to be considered in the initial rehabilitation phase, and is often implemented 3–7 days post injury (Jarvinen et al. 2007), usually with a focus on preventing muscle atrophy and/or a loss of strength. Frequency, duration and intensity are limited by the patients’ pain. Some therapists advocate three sets of 10 repetitions using 5–10 second holds to begin with at intensity within pain tolerance (Pull and Ranson 2007). These then are undertaken at multiple angles, beginning in mid range then progressing to inner range (shortened position) then outer range (lengthened position).
The sports rehabilitator should continue to monitor the progress of the athlete taking into consideration exercise frequency, intensity and duration. Early phase strengthening exercises, involve an isometric contraction of the agonist muscle with no movement at the joint. The contraction should be performed at different joint angles to initiate muscle fibre activation in different planes of motion. The stabilising force surrounding the joint is the antagonist muscle (Middleton and Smith 2007). Pain management and the acknowledgement of pain free training should be considered simultaneously with the implementation of strength training protocols. This is evident within the isometric strength development, where the primary goal is to create an adaptive response in the muscle, without stressing the joint to excess (Liebenson 2006). Once an athlete is able to perform isometric muscle contractions at various joints without the onset of pain, it may then be advisable to progress the athlete onto dynamic exercises (Newberry and Bishop 2006). Sports rehabilitators must ensure that the athlete has full bilateral range of motion, no swelling or pain prior to implementation of progressive, dynamic, functional based activity (Beam 2002).
During isotonic exercise the athlete must provide a force powerful enough to initiate concentric and eccentric muscle actions, whilst coping with a constant external load. There are different isotonic exercises that could be embedded into the rehabilitation process. These can include use of dumbbells, machine weights, and resistance bands (Beam 2002).
Muscle injuries are associated with preferential atrophy of type 1 muscle fibres with disuse (Stockmar et al. 2006), and high loads and rates of force development are most likely to over stress the healing tissue. Therefore, initially an endurance based programme should be used (3 sets of ≥15 repetitions at 40–60% of one repetition maximum) this should be progressed to strength (4–6 sets of 3-6 repetitions at 85–95% of one repetition maximum and then power training (3–5 sets of 3–5 repetitions at 75–85% of one repetition maximum) (Kraemer et al. 2002).
A key consideration during the progression of isotonic training is the velocity at which the movement is performed (Wrbaskic and Dowling 2007). Initially the athlete should perform slow and controlled movements in order to allow for increased neural response to the working muscles and the continued development of neuromuscular control. Once the athlete is able to control the exercise effectively the continual progression to sports specific, functional activity coupled with changes in velocity, increased load and use of different planes of motion, should be considered (Liebenson 2006).
Through training, the body’s systems and tissues adapt in direct response to the stresses imposed on the body during each training session. The adaptation responses can be explained through the general adaptation syndrome (GAS) first described by Hans Seyle in (1985) (Figure 12.5).
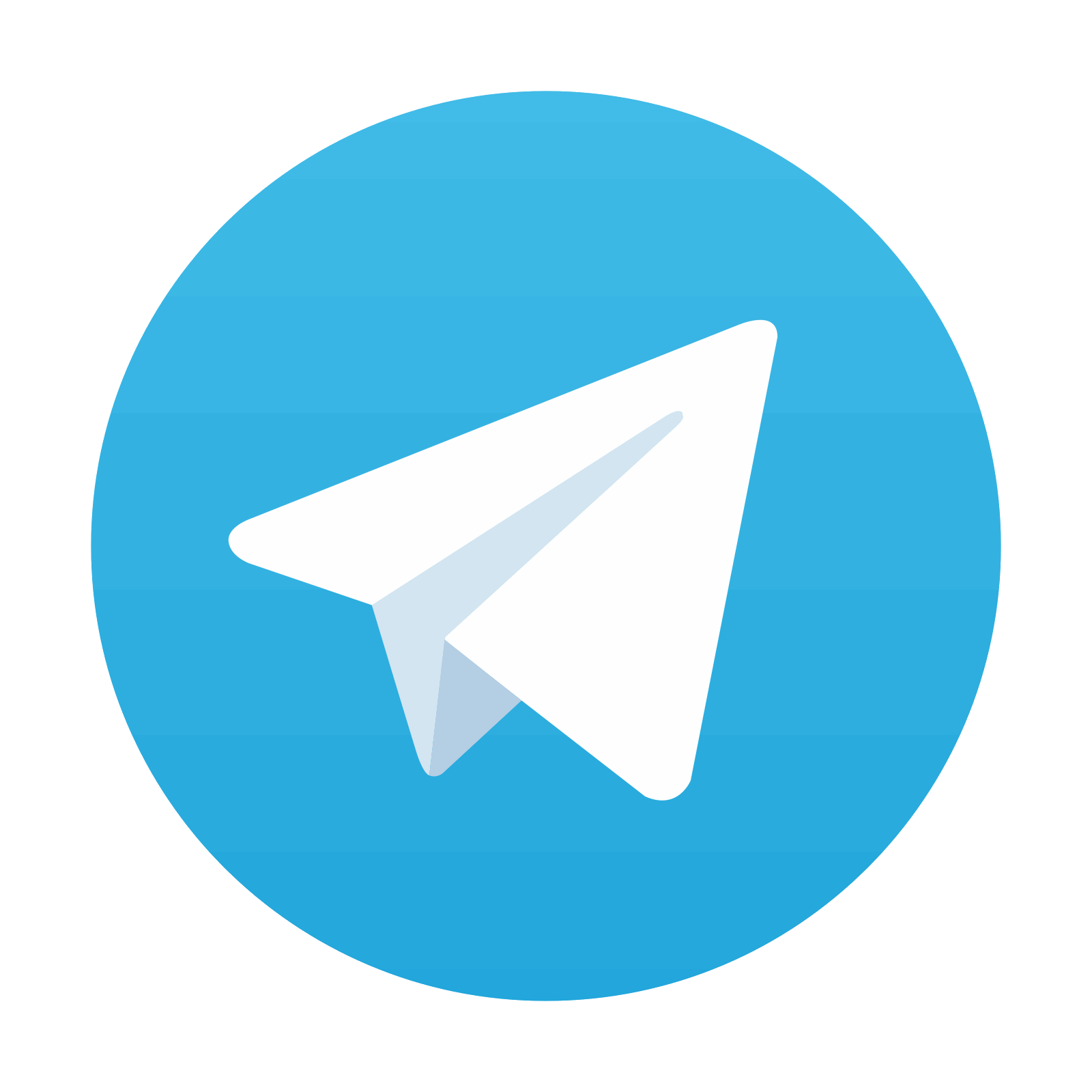
Stay updated, free articles. Join our Telegram channel

Full access? Get Clinical Tree
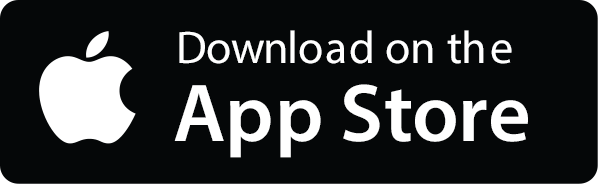
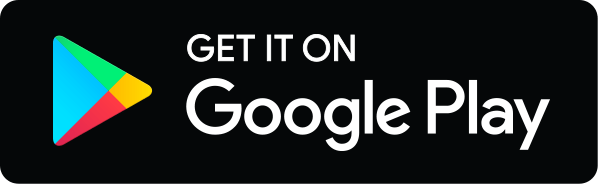