Recent advances have increased the understanding of the pathogenesis of polymyositis and dermatomyositis. Clearly, the pathogenesis is complex, and adaptive (eg, autoimmune) and innate and nonimmune pathways play a role in the disease mechanisms, but the relative contribution may vary between patients and in different phases of the disease. Phenotyping patients using autoantibody profiling has resulted in information on molecular pathways that may be relevant in certain subsets of patients with polymyositis or dermatomyositis, but combining the autoantibody profiles with molecular signatures of innate and nonimmune mechanisms would enhance our ability to classify, diagnose, and treat these disorders more effectively.
Recent advances clearly have increased the understanding of the pathogenesis of polymyositis and dermatomyositis. Immune (adaptive and innate) and nonimmune pathways play a role in the disease pathogenesis. The magnitude and exact nature of the contribution of these pathways to disease initiation and progression are still unclear. Understanding the relative contribution of these pathways is important to design rational therapies for these disorders. Therefore, this review summarizes some of these concepts and recent advances in pathogenic mechanisms in polymyositis and dermatomyositis.
Adaptive immune mechanisms
Inflammatory myopathies are classified as autoimmune diseases because many patients show antibodies to specific autoantigens (eg, anti-Jo) and because of the presence of T cells in large infiltrates in muscle tissue. Another support for the involvement of adaptive immunity is the strong association with HLA-DR genotypes because the function of the HLA-DR molecules is to present antigens to T cells. In addition, the frequent association with other autoimmune and vascular diseases (eg, Hashimoto thyroiditis and scleroderma) coupled with favorable response to immunosuppressive and immunomodulatory therapies in some patients further supports that these myopathies are autoimmune diseases.
Humoral Immune Response
Up to 80% of patients with polymyositis and dermatomyositis have positive antinuclear antibodies and/or specific autoantibodies. Some of these autoantibodies are specific to myositis (eg, aminoacyl transfer RNA [tRNA] synthetases and anti-nuclear helicase [Mi-2]) and others not specific to but associated with myositis (eg, anti-snRNP, anti-Ro/SSA, anti-Ku, and anti-PMS1). Some of these antibodies are clearly associated with distinct clinical features of the disease; for example, anti-Mi-2 antibodies show very strong association with dermatomyositis with prominent features such as Gottron papules, heliotrope rash, the V sign, and shawl sign, whereas the most frequent myositis-specific autoantibodies, for example, the aminoacyl tRNA synthetases, are associated with another distinct clinical entity named antisynthetase syndrome with features including myositis, interstitial lung disease (ILD), nonerosive arthritis, Raynaud phenomenon, and skin rash on the hands, the so-called mechanic’s hands. To date, 8 different antisynthetase autoantibodies have been identified, of which anti-histidyl-tRNA synthetase (anti-Jo-1) autoantibodies are the most frequent, being present in 20% to 30% of patients with polymyositis or dermatomyositis. The others are anti-PL-7 directed against threonyl-tRNA synthetase, anti-PL12 directed against alanyl-tRNA synthetase, anti-KS directed against aspariginyl-tRNA synthetase, anti-OJ directed against isoleucyl-tRNA synthetase, anti-EJ directed against glycyl-tRNA synthetase, anti-Ha directed against tyrosyl-tRNA synthetase, and anti-ZO directed against anti-phenylalanyl-tRNA synthetase. The most common shared clinical feature of these autoantibodies is ILD, which may precede myositis or be present even without myositis. A more recently detected autoantibody, anti-P155/140, is strongly associated with dermatomyositis and cancer as well as with juvenile dermatomyositis. Taken together, the strong associations with distinct clinical phenotypes indicate that some of these autoantibodies are excellent clinical markers and are useful in classifying these heterogeneous disorders into homogeneous subgroups that may share pathogenic mechanisms.
The role of B cells in the pathogenesis of polymyositis and dermatomyositis is supported by the presence of B cells and plasma cell infiltrates in the muscle tissue and that immunoglobulin transcripts are among the most abundant of the immune transcripts in the muscle tissue of patients with myositis. More recently, analyses of the variable regions’ gene sequences revealed clear evidence of significant somatic mutation, isotype switching, receptor revision, codon insertion/deletion, and oligoclonal expansion, suggesting that affinity maturation had occurred within the B-cell and plasma cell populations in the muscle tissue. Antigens localized to the muscle might drive a B-cell antigen-specific response in myositis, and these antigens could be autoantigens or exogenous cross-reactive antigens derived from viruses or other infectious agents. The contribution of myositis-specific autoantibodies to the disease pathogenesis is still unclear because these antibodies are often directed to ubiquitous intracellular proteins that are not muscle specific. Although the myositis-specific autoantibodies are directed against ubiquitous intracellular proteins, there is a differential organ expression of these antigens whereby histidyl-tRNA synthetase has a higher expression in the epithelial cells of the bronchi than in other healthy organs. Moreover, a proteolytically sensitive conformation of the histidyl-tRNA synthetase has been demonstrated in the lung, which might suggest that autoimmunity to histidyl-tRNA synthetase is initiated and propagated in the lung. Furthermore, mice immunized with murine Jo-1 develop a striking combination of muscle and lung inflammation that replicates features of the human antisynthetase syndrome. Moreover, both histidyl-tRNA synthetase and the MI-2 antigens are clearly upregulated in muscle fibers undergoing regeneration in patients with myositis, suggesting that muscle fibers may become potential targets of the immune system after trauma. However, it is unlikely that these antibodies bind to their intracellular targets and contribute to significant tissue damage in myositis, but they could be involved in the disease pathogenesis in other ways by modulating the muscle microenvironment.
The histidyl-tRNA synthetase autoantibodies are the most studied for potential pathogenic relevance. Presence of anti-Jo-1 antibodies is strongly associated with HLA-DRB1*0301 genotype, and a few case reports suggest that these autoantibodies precede the onset of clinical myositis or ILD and that they vary in serum levels with disease activity. The antibody response to histidyl-tRNA synthetase undergoes class switching, spectrotype broadening, and affinity maturation, all of which are indicators of a T-cell–dependent antigen-driven process. Sera from patients with anti-Jo-1 antibodies have a type I interferon–inducing capacity, similar to anti-U1RNP and anti-SSA in systemic lupus erythematosus (SLE) and Sjögren syndrome and could thereby have a role in the pathogenesis by activating the type I interferon system, which could have several roles in autoimmune diseases as discussed later. In addition, sera from patients with anti-Jo-1 antibodies induced higher expression of intercellular adhesion molecule (ICAM)-1 on microvascular endothelial cells of lungs compared with other sera from patients with myositis. Thus, there is a possibility that these autoantibodies or yet unknown serum-associated factors might activate endothelial cells of microvessels and thereby facilitate homing of inflammatory cells into tissues. Presence of anti-Jo-1 antibodies is also associated with high serum levels of B-cell–activating factor, similar to what has been reported for anti-SSA in patients with SLE or Sjögren syndrome. Taken together, accumulating data to date might indicate an indirect pathogenic role of anti-Jo-1 autoantibodies.
Cell-Mediated Immune Response
There are mainly 2 different patterns of distribution, location, and type of lymphocyte subsets in the muscle tissue, suggesting 2 different pathways; one is dominated by CD4 + T lymphocytes, macrophages, B lymphocytes, plasma cells, and dendritic cells predominantly in perivascular and perimysial areas of tissue, which is mainly but not exclusively found in patients with dermatomyositis. The other is dominated by CD8 + T and CD4 + T lymphocytes, macrophages, and dendritic cells predominantly in an endomysial distribution, with inflammatory cells sometimes invading nonnecrotic muscle fibers. The latter is mainly found in patients with myositis without skin rash and polymyositis. The differences in location and inflammatory cell types suggest 2 different pathogenic pathways: one directed against blood vessels and the other directed against muscle fibers. However, these patterns are not mutually exclusive, because in some patients, these 2 patterns of inflammatory cell distribution are seen in the same specimen. The vascular involvement is in patients with dermatomyositis clearly manifested in the skin and can be clinically seen in the form of capillary nailfold changes. The capillary changes and damage are attributed to complement deposition. Capillaries in both polymyositis and dermatomyositis are often fewer per muscle area compared with those in healthy individuals, and they are often abnormally thickened and may show hyperplasia and necrosis. Likely, these morphologic changes could contribute to an ischemia that, in turn, could cause muscle fiber damage.
The endomysial inflammatory infiltrates contain a high percentage of activated CD8 + T lymphocytes, macrophages, and CD4 + T lymphocytes. CD8 + cytotoxic T lymphocytes recognize major histocompatibility complex (MHC) class I on muscle fibers and may mediate muscle fiber damage. This fact is partially supported by the evidence that (1) perforin-expressing cytotoxic T cells are seen to be oriented toward the target muscle fiber and by (2) clonal proliferation of CD8 T cells, both within the muscle, and that (3) T cell lines from patients show cytotoxicity against autologous myotubes. In some patients, T cells are present in muscle tissue even after treatment with high doses of glucocorticoids in combination with other immunosuppressive drugs. In this context, a subset of T lymphocytes, CD28 null T cells, are of interest. CD28 null T cells are apoptosis-resistant T lymphocytes that are terminally differentiated and lack CD28. They are often clonally expanded and have acquired new effect or functions and upregulated a set of activating receptors mostly associated with natural killer (NK) cells. Further characterization of the T lymphocytes in polymyositis and dermatomyositis has revealed that a large proportion of CD4 + and CD8 + T lymphocytes both in peripheral blood and in muscle tissue are of CD28 null T-cell phenotype. They were easily stimulated to produce proinflammatory cytokines, for example, interferon-γ and tumor necrosis factor (TNF). They contain perforin and granzyme, and therefore, both CD4 + and CD8 + CD28 null T cells have cytotoxic potentials, but whether they have a myocytotoxic capacity is still unknown. In polymyositis, there was an association between CD28 null T cells and disease activity, further supporting a role of these cells in disease mechanisms. The causative factor of CD4 + and CD8 + CD28 null T cells in myositis is not known. High frequencies of CD4 + CD28 null T cells have been found in peripheral blood in other autoimmune diseases, for example, rheumatoid arthritis, and in cardiovascular disease, whereas CD8 + CD28 null T cells are associated with repeated antigen stimulation and are increased in peripheral blood of individuals with chronic viral infections, such as Epstein-Barr virus, human cytomegalovirus (HCMV), and human immunodeficiency virus. In polymyositis and dermatomyositis, both CD4 + and CD8 + CD28 null T cells were strongly associated with immunity to HCMV, but a causal relationship with HCMV has not been confirmed. Moreover, the specificity of the CD28 null T cells in polymyositis and dermatomyositis is not known but should be subject of future studies.
Innate and nonimmune mechanisms
Cytokines
Effector molecules (cytokines and chemokines) that are produced by muscle fibers, inflammatory cells, and endothelial cells are thought to contribute to the pathogenesis of myositis. Proinflammatory cytokines such as interleukin (IL)-1α, IL-1β, TNF-α, type I interferons (interferon-α and -β), the DNA-binding nonhistone protein, high-mobility group box 1 (HMGB1), and chemokines such as the alpha-chemokines CXCL9 and CXCL10 and the beta-chemokines CCL2, CCL3, CCL4, CCL19, and CCL21 are present in the muscle tissue of patients with polymyositis and dermatomyositis. Other more recently reported cytokines that may also have a role in the pathogenesis of myositis are IL-15 and IL-18, although their role needs to be further explored. These molecules may not only amplify the immune response within the muscle microenvironment but also have a direct effect on muscle fiber function. The relative importance of the various cytokines and chemokines in patients with myositis is still uncertain, but these molecules offer possible targets for therapy in these conditions. One way forward to achieve increased understanding of the molecular pathways in myositis is to investigate the target organ, muscle, for molecular expression in different phases of disease and in longitudinal studies using different therapies and to relate the effects on molecular expression to the effects on clinical performance.
Interleukin-1
The most consistently found cytokines in various phases of disease, at diagnosis before and after treatment, in patients with persisting muscle weakness are IL-1α and HMGB1, both molecules that may be present not only in inflammatory cells but also in endothelial cells and muscle fibers even in the absence of inflammatory cell infiltrates. A possible direct effect of IL-1 on muscle fibers is supported by the presence of IL-1RI and IL-1RII in the muscle fiber membrane. Direct functional studies have not been published to demonstrate this effect, but treatment with IL-1 receptor antagonist, anakinra, has demonstrated efficacy in occasional patients, supporting a role of IL-1 in some patients with myositis. In the C-protein–induced myositis model, muscle inflammation depended on IL-1 but not on TNF, lending a further support for a role of IL-1 in myositis.
Enzymes in the prostaglandin pathway have been recorded in the muscle tissue: microsomal prostaglandin E synthase 1 (mPGES-1), cyclooxygenase (COX)-1, and COX-2. Conventional immunosuppressive treatment led to a significant downregulation of COX-2 in the muscle tissue of patients with myositis, but the expression of mPGES-1 and COX-1 remained unchanged, indicating a role of these enzymes in the chronicity of polymyositis and dermatomyositis. Their role in the pathogenesis of myositis is not known. IL-1β, which is markedly expressed in the muscle tissue of patients with myositis, may stimulate prostaglandin E 2 (PGE 2 ) production in skeletal muscles; therefore, PGE 2 expression may be a downstream effect of IL-1.
Type I Interferon System
The type I interferon system has gained much focus as having a role in propagating autoimmune diseases by its ability to break tolerance, primarily based on the clinical observation that treatment with type I interferon could induce autoimmune disease such as SLE. There are also a few case reports of myositis onset during interferon treatment. In polymyositis and dermatomyositis, a type I interferon gene signature has been observed in the muscle tissue and peripheral blood, and the interferon gene signature in peripheral blood was associated with disease activity. Moreover, an interferon-inducing capacity was strongly associated with anti-Jo-1 and/or anti-SSA autoantibodies, as mentioned earlier, and correlated with MHC class I expression in muscle fibers. In addition, the major producer of type I interferon, plasmacytoid dendritic cells, and the interferon-inducible protein, MXA, were present in the muscle tissue of preferentially not only patients with anti-Jo-1 and anti-SSA autoantibodies but also patients with dermatomyositis without detectable antibodies, indicating 2 different mechanisms that might be linked via the interferon pathway. Targeting type I interferon, which is now possible, seems to be an attractive treatment, at least in subgroups of patients with myositis with anti-Jo-1 or anti-SSA antibodies and some patients with dermatomyositis without these autoantibodies.
HMGB1 Protein
The alarmin HMGB1 is a ubiquitous nonhistone molecule present in all nucleated cells in which it is bound to DNA. It can be actively released from monocytes and macrophages and can be released from other cells undergoing necrosis. Extranuclear HMGB1 may have proinflammatory properties and may also have a function in muscle cell regeneration. Extracellular HMGB1 was detected in the muscle tissue of patients with polymyositis and dermatomyositis and was present even after treatment with high doses of glucocorticoids in which it was localized to the cytoplasm of muscle fibers and endothelial cells even in the absence of inflammatory infiltrates. A similar expression and co-localization of HMGB1- and MHC class I–positive muscle fibers were also evident in early cases without detectable inflammatory infiltrates. In these tissues, HMGB1-expressing fibers outnumbered fibers expressing MHC class I. Functional studies demonstrated that HMGB1 can induce a reversible upregulation of MHC class I in the muscle fibers. Moreover, HMGB1 exposure may cause an irreversible decrease in Ca 2+ release from the sarcoplasmic reticulum during fatigue induced by repeated tetanic contractions, which is a proxy for muscle performance, suggesting that HMGB1 has a direct negative effect on muscle fiber contractility. Furthermore, exposure to interferon-γ induced translocation of HMGB1 to the muscle fiber sarcoplasm. Therefore, HMGB1 may also be a possible endogenous molecule in muscle fibers that under inflammatory stress might be translocated from the fiber nuclei to the sarcoplasm and possibly affect MHC class I expression in muscle fibers. Hypoxia is another factor that can upregulate expression of HMGB; thus, HMGB1 could be an early inducer of skeletal muscle dysfunction in polymyositis and dermatomyositis independent of the presence of inflammatory cell infiltrates.
Hypoxia
Microvessel involvement (eg, expression of adhesion molecules and IL-1α) and loss are present in both dermatomyositis and polymyositis. Clinical symptoms and muscle fatigue commonly seen in these patients could be because of muscle tissue hypoxia. A local tissue hypoxia is further supported by upregulation of vascular endothelial growth factor seen in muscle tissue and in sera of patients with polymyositis and dermatomyositis. The hypoxia hypothesis is supported by the evidence that clinical improvement is observed after exercise, and magnetic resonance spectroscopic analysis showing reduced levels of energy substrates (eg, ATP and phosphocreatine) before and after a workload indicates that an acquired metabolic disturbance occurs in inflammatory myopathies, and this metabolic disturbance likely contributes to impaired performance of skeletal muscle in these patients.
MHC Class I Expression in Muscle Fibers
MHC class I molecules are known to play a critical role in initiating and perpetuating antigen-specific immune responses by presenting antigenic peptides to CD8 + T lymphocytes and by regulating the activities of NK cells. Recently, it is becoming increasingly clear that MHC class I molecules have broader nonimmune functions that are not associated with antigen presentation to lymphocytes; for example, MHC class I molecules are (1) important for the retraction of synaptic connections that normally occur during development, (2) crucial for maintenance of synapses during the synaptic removal process in neurons after lesion, and the absence of MHC class I expression may impede the ability of neurons to regenerate axons, and (3) important for receptor-mediated transmembrane signal transduction and cell-cell communications in multiple cell types.
In normal differentiated skeletal muscle cells, MHC class I is either absent or expressed at low levels; however, they can be promptly induced by proinflammatory cytokines, for example, interferon-γ or TNF-α. In contrast, skeletal muscle of patients with myositis shows increased expression of MHC class I not only in regenerating muscle fibers but also in nonnecrotic muscle cells. The biologic significance of these observations has been explored by generating a conditional transgenic mouse model overexpressing syngenic mouse MHC class I in the skeletal muscle. These mice show many features (eg, clinical, histologic, and immunologic) that resemble those of human myositis and provide a close model of the disease in humans. A series of observations in patients with human myositis and in the mouse model of myositis suggest that MHC class I molecules themselves may potentially mediate muscle fiber damage and dysfunction through innate and nonimmune mechanisms in the absence of lymphocytes: (1) MHC class I staining of muscle specimens from patients with myositis shows both a cell surface and an internal reticular pattern of reactivity, indicating that some of the MHC class I molecules may be retained in the endoplasmic reticulum (ER) of muscle fibers. (2) MHC class I overexpression persists in muscle fibers in the absence of an inflammatory infiltrate. (3) In vivo gene transfer of MHC class I expression plasmids attenuates muscle regeneration and differentiation, and the induction of MHC class I in the skeletal muscle of the transgenic mouse model of myositis results in muscle atrophy and an intrinsic decrease in force-generating capacity.
Collectively, these observations indicate that the muscle impairment and fiber damage seen in myositis may be mediated not only exclusively by CD8 + cytotoxic T lymphocyte attack but also through nonimmunologic mechanisms such as the ER stress response and hypoxia.
ER Stress
In patients with myositis, increased expression of MHC class I in myofibers initiates a series of cell autonomous changes that lead to myofiber damage. The authors and other researchers have shown that overexpression of MHC class I on muscle fibers of patients with myositis and the transgenic mouse model of myositis results in activation of the nuclear factor (NF)-κB and ER stress response pathways. The authors and other researchers have shown that NF-κB is activated and several downstream NF-κB target genes (eg, MHC class I, ICAM, and monocyte chemoattractant protein-1) are increased in human myositis specimens and in the mouse model, suggesting that this pathway may be directly involved in muscle fiber impairment and damage in myositis. Thus, MHC class I expression in the skeletal muscle links the classic immune (through CD8 T cells) and nonimmune (ER stress) mediated mechanisms of muscle impairment and damage. Furthermore, the role of ER stress in skeletal muscle function is explored recently using hexose-6-phosphate dehydrogenase (H6PD)–deficient mice. H6PD is an enzyme that generates nicotinamide adenine dinucleotide phosphate via the pentose phosphate pathway inside the ER, and H6PD-deficient mice have severe skeletal myopathy and exhibit fasting hypoglycemia, increased insulin sensitivity, and basal and insulin-stimulated glucose uptake in fast skeletal muscle fibers, indicating mild insensitivity to glucocorticoids. These studies also showed large intrafibrillar membranous vacuoles and abnormal triads in the affected muscle, indicating an altered redox state of sarcoplasmic reticulum that, in turn, leads to activation of unfolded protein response and myopathy.
Cell Death
Although classic apoptosis is not always detected in muscle specimens, signs of muscle fiber degeneration and necrosis are characteristic histopathologic features of polymyositis and dermatomyositis that may contribute to loss of muscle performance. Some, but not all, patients develop clinical muscle atrophy, which naturally may affect muscle strength and endurance. The mechanisms that cause muscle fiber degeneration or muscle atrophy have not been clarified, and both cytotoxic cell death and an effect of disuse or a negative effect of glucocorticoids have been suggested. An increasing knowledge of the mechanisms that cause muscle cell death is important to develop targeted therapies in myositis.
Some studies showed that classic apoptotic changes are absent in skeletal muscle fibers of patients with myositis because of the expression of antiapoptotic molecules (eg, FLICE inhibitory protein and inhibitor of apoptosis) in the skeletal muscle of these patients, indicating that other forms of cell death (eg, autophagy) may be responsible for muscle cell death in myositis. Recent literature supports that ER stress response and autophagy are interconnected, for example, endogenous ER degradation–enhancing α mannosidase-like protein 1 in nonstressed cells reaches the cytosol and is degraded by basal autophagy. Although caspase-3 activation is not clearly related to myositis, it has been well demonstrated that caspase-12 is activated in the murine transgenic mouse model of myositis, but its role in human skeletal muscle is still unclear. Moreover, accumulation of cell surface proteins such as MHC class I in the muscle fibers of patients with myositis may also lead to both ER stress and autophagy.
The role of autophagy in myositis is unexplored. One study recently compared clinical and histologic features of muscle specimen from patients with polymyositis with those of mitochondrial disease, steroid-responsive polymyositis, and inclusion body myositis. It was observed that selective weakness in the quadriceps or finger flexors was common in PM-Mito and IBM and progressed slower in PM-Mito than in IBM and that autophagy markers LC3 and α-B-crystallin were found in PM-Mito and IBM but not in polymyositis specimens. Recent studies suggest that autophagy signaling (Beclin 1 and LC3-II) is upregulated in response to denervation and may preferentially target mitochondria for degradation in the skeletal muscle and consequently loss of skeletal muscle mass. Deficiency of a component of V-ATPase proton pump complex (VMA21) leads to mammalian target of rapamycin (mTOR)-dependent autophagy, vacuolation, and atrophy of skeletal muscle in cell X-linked myopathy with excessive autophagy (XMEA). These findings provide clues to the perifascicular atrophic phenotype seen in muscle specimens from patients with dermatomyositis.
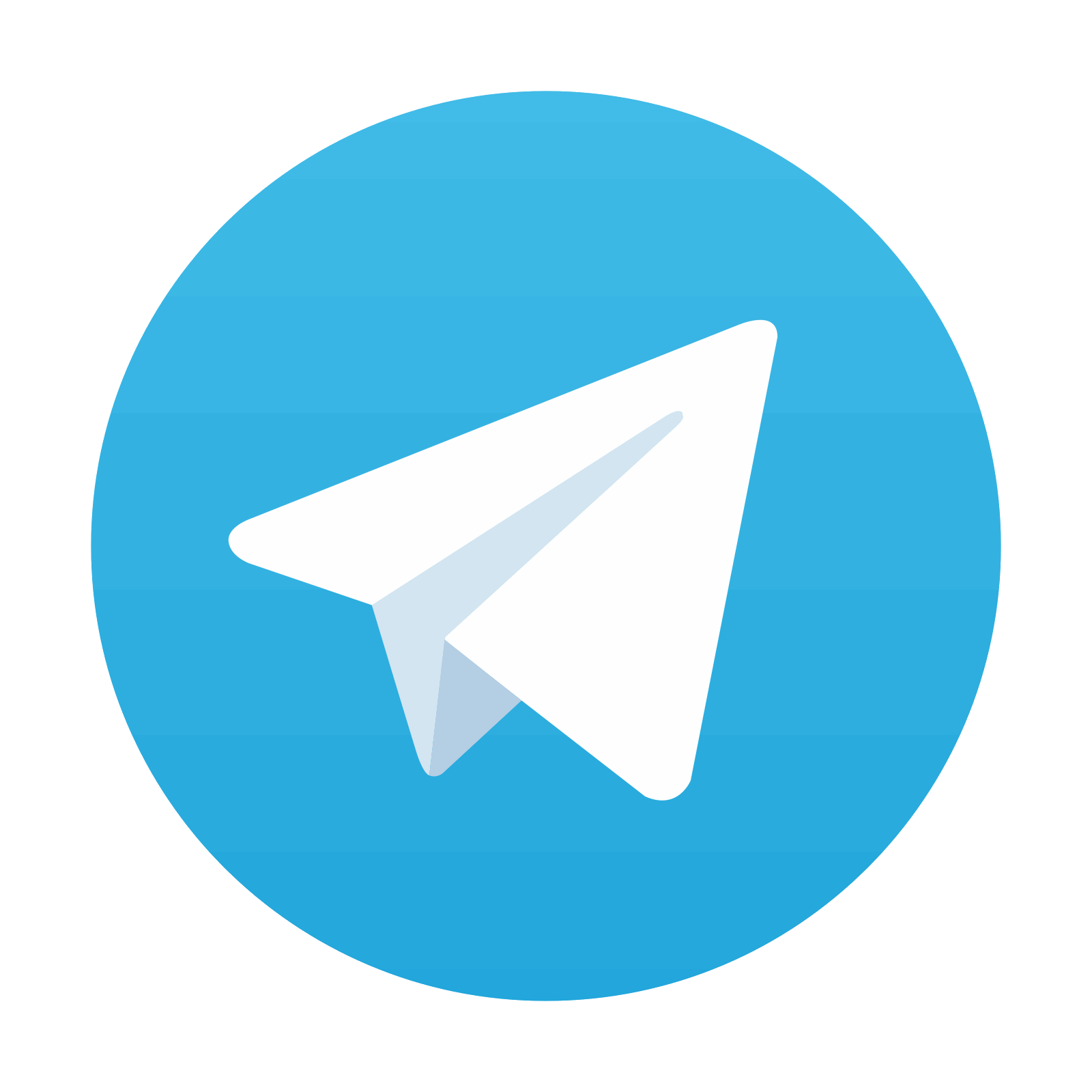
Stay updated, free articles. Join our Telegram channel

Full access? Get Clinical Tree
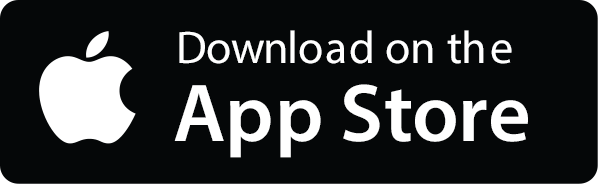
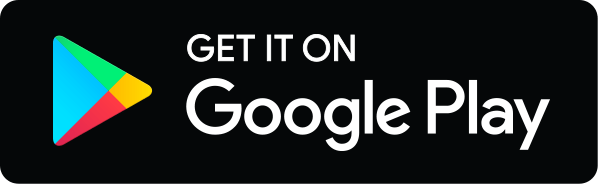