The rheumatologist is frequently called on to evaluate patients with complaints of myalgia, muscle cramps, and fatigue. The evaluation of these patients presents a diagnostic challenge given the nonspecific and intermittent nature of their complaints, often leading to inappropriate diagnostic testing. When these symptoms are associated with physical exertion, a metabolic myopathy should be suspected Although inflammatory myopathies may present with similar features, such a pattern should prompt a thorough evaluation for an underlying metabolic myopathy. This review discusses the most common causes of metabolic myopathies and reviews the current diagnostic options available to the clinician.
The metabolic myopathies are a heterogeneous group of disorders that share the common feature of inadequate production of cellular energy in the muscle ( Table 1 ). These myopathies are often categorized as either hereditary (primary) disorders or acquired (secondary) disorders. A further clinical distinction can be made between those disorders associated with primarily dynamic features characterized by transient, exercise-induced fatigue, muscle cramping, and rhabdomyolysis and those disorders associated with primarily static features such as fixed weakness.
Disorder | Presentation | Provocation | Screening Laboratory Testing | Confirmatory Testing | Treatment | Differential Diagnosis | Inheritance | OMIM Number |
---|---|---|---|---|---|---|---|---|
Dynamic Myopathies | ||||||||
McArdle disease (GSD V) | Acute rhabdomyolysis, second wind phenomenon | Vigorous exercise | Abnormal ischemic forearm test, ± elevated CK, elevated muscle glycogen content | Myophosphorylase gene mutation; phosphorylase activity | Oral sucrose before exercise | PK deficiency | Autosomal recessive | #232600 |
Carnitine palmitoyl transferase II (CPT II) deficiency | Delayed rhabdomyolysis | Fasting, prolonged exercise | Abnormal acylcarnitine profile when stressed, normal CK | CPT II gene mutation, CPT activity in fibroblasts or muscle | Carbohydrate ± MCT oil before exercise, avoidance of fasting | Disorders of β-oxidation of long-chain fatty acids | Autosomal recessive | #255110 |
Phosphofructokinase (PFK) deficiency (GSD VII/Tarui) | Exercise intolerance, muscle pain | Stressful illness, exercise | ± Elevated CK, ± elevated muscle glycogen content | PFK gene mutation, PFK activity in muscle | High protein diet, aerobic conditioning | Mitochondrial disorders | Autosomal recessive | #232800 |
Phosphorylase b kinase (PK) deficiency (GSD IXd) 16 | Acute rhabdomyolysis | Vigorous exercise | ± Elevated CK, elevated muscle glycogen content | PK gene mutation, PK activity in muscle | High protein diet | McArdle disease | Autosomal recessive or X-linked recessive | #300559 |
Static Myopathies | ||||||||
Mitochondrial disorders (mitochondrial oxidative phosphorylation defects) | Exercise intolerance | Stressful illness,exercise | Abnormal urine organic acids, normal CK | Electron transport chain (ETC) testing in muscle, coenzyme Q 10 in muscle | Coenzyme Q 10 /creatine monohydrate/α-lipoic acid supplementation | PFK deficiency | Autosomal recessive or maternal inheritance (mitochondrial DNA mutations) | MERRF #545000; Kearns-Sayre syndrome #530000; many others |
Acid maltase deficiency (GSD II)/Pompe disease | Proximal weakness | Not applicable | Elevated urinary glucose tetrasaccharide, baseline elevated CK, elevated muscle glycogen content | Acid α-glucosidase (GAA) gene mutations, GAA activity in blood, fibroblasts, muscle | Enzyme replacement with recombinant human GAA | Limb girdle muscular dystrophies | Autosomal recessive | #232300 |
A detailed review of muscle energy metabolism is beyond the scope of this review, but a brief consideration of the pertinent metabolic pathways is useful to understand better this group of disorders. Under normal circumstances, energy for skeletal muscle function in the form adenosine triphosphate (ATP) is derived from muscle glycogen, blood glucose, and free fatty acids. Each of these primary energy sources is metabolized through specific biochemical pathways into the final common product, ATP. The majority of fuel for muscle is provided by carbohydrates in the form of glycogen and by lipids in the form of free fatty acids. Through the process of anaerobic glycolysis, glycogen is metabolized to pyruvate inside the muscle cells ( Fig. 1 ). Pyruvate is then decarboxylated into acetyl-coenzyme A (acetyl-CoA) inside the mitochondria. Similarly, β-oxidation of free fatty acids (fatty acid oxidation; FAO) inside mitochondria provides another source of acetyl-CoA ( Fig. 2 ). Acetyl-CoA then enters the Krebs cycle, generating reduced electron carriers that deliver electrons to the mitochondrial electron transport chain, thus driving the production of energy in the form of ATP (see Fig. 1 ). Defects in any one of the steps involved in this complex metabolic pathway can lead to an insufficient supply of ATP and an inability to sustain normal muscle function in times of increased metabolic demand.


The complex nature of the various metabolic pathways involved is reflected in the broad phenotypic presentation of many of these disorders (see Table 1 ). Classic symptoms of the metabolic myopathies, including early exertional fatigue, myalgias, muscle cramps or contractures, and myoglobinuria, may emerge during times of high energy demand due to exercise, illness, or fasting. Because the predominant energy substrate varies as a function of the intensity and duration of exercise, a detailed clinical history can often provide important diagnostic clues. Glycogen breakdown provides substrates for cellular energy production during rapid, vigorous exercise, whereas FAO acts as the main player during prolonged exercise and/or fasting. At rest, free fatty acids provide the major energy source for skeletal muscle via aerobic metabolism. By contrast, brief, high-intensity (isometric) exercise such as weight-lifting relies heavily on anaerobic glycogenolysis for a rapid, short-lived energy supply. The primary source of energy for muscle for light-to-moderate intensity exercise of short duration is predominantly glucose and free fatty acids. As exercise duration increases glycogen stores are depleted, and the demand for free fatty acid–derived energy increases. This process explains the characteristic pattern of muscle fatigue, stiffness, cramping, and myalgias that occur shortly after brief, maximal exertion in the myogenic forms of glycogen storage diseases (GSDs). By contrast, symptoms associated with disorders of lipid metabolism typically appear after prolonged, moderate-intensity exercise, such as jogging or swimming. Thus, patients with McArdle disease (GSD type V) become symptomatic during intense exercise, whereas in carnitine palmitoyltransferase II (CPT II) deficiency, an FAO disorder, patients present following sustained exercise and other metabolic stressors (see Table 1 ).
The variable and often transient nature of these symptoms can present a challenging diagnostic dilemma. Depending on the underlying metabolic defect, some patients may present in infancy or early childhood, whereas others may not present until well into adulthood. Furthermore, some disorders may be strictly limited to muscle pathology whereas others, particularly those involving mitochondrial dysfunction, may demonstrate multisystem involvement. To make a proper diagnosis, it also becomes necessary to recognize other inherited and acquired conditions mimicking metabolic myopathies.
Several acquired and inherited conditions may mimic metabolic myopathies, and must be properly diagnosed both to avoid unnecessary and potentially harmful therapies and to allow appropriate treatment and counseling ( Box 1 ). For example, statins, a common group of drugs used for treatment of hypercholesterolemia, has emerged as a cause of iatrogenic myalgia. The precise mechanism of muscle injury in statin-induced myopathy is unknown, but there appears to be a dose-related effect, with higher doses leading to myopathy and rhabdomyolysis. A recent study has shown an increased risk for statin-induced myopathy in patients harboring a common single-nucleotide polymorphism in the SLCO1B1 gene on chromosome 12. This gene is involved in the hepatic uptake of statin drugs. The incidence of homozygosity or heterozygosity for mutations associated with rare metabolic myopathies was increased in a large group of patients evaluated for statin-induced myopathy. Muscle coenzyme Q 10 deficiency was highly prevalent in this group, and their response to supplementation remains to be established; however, this biochemical abnormality could shed light on the pathogenesis of statin-induced myopathy.
- 1.
Inflammatory myopathy (dermatomyositis, polymyositis, inclusion body myositis)
- 2.
Necrotizing, noninflammatory myopathies (paraneoplastic, anti–signal recognition particle)
- 3.
Infection (viral)
- 4.
Toxin/drug-induced (ethanol, statins, cyclosporine, colchicine)
- 5.
Endocrinopathies (hypo-/hyperthyroidism, hypo-/hyperparathyroidism, diabetes mellitus)
- 6.
Polymyalgia rheumatica
- 7.
Fibromyalgia
- 8.
Benign cramp-fasciculation syndrome
- 9.
Restless leg syndrome
- 10.
Muscular dystrophies (dystrophinopathies, limb girdle muscular dystrophy)
- 11.
Myotonic disorders (myotonic dystrophy type 2, myotonia congenita)
- 12.
Motor neuron disease (amyotrophic lateral sclerosis, spinal muscular atrophy)
Other myopathies that may mimic metabolic myopathies include limb-girdle muscular dystrophies or, more commonly, chronic inflammatory myopathies such as polymyositis. Systemic manifestations such as a rash, malaise, interstitial fibrosis, carditis, and gastrointestinal dysfunction may suggest the presence of an acquired inflammatory myopathy, but the distinction can be difficult. For a more detailed discussion, the reader is referred to an excellent review of the subject.
Many tools are available to the clinician to aid in the diagnostic evaluation of metabolic myopathies. As discussed later with each group of disorders, one of the most cost-effective and least invasive tools remains the clinical history and physical examination that can provide invaluable information, allowing the clinician to narrow or broaden further diagnostic testing as appropriate and avoid unnecessary invasive testing. A thorough history and examination will often help differentiate a dynamic myopathy characterized by transient, severe symptoms from a static myopathy characterized by fixed weakness (see Table 1 ). As the primary metabolic myopathies are hereditary, a thorough, targeted family history may provide valuable information. Once a thorough history and physical examination have been performed, routine laboratory tests including serum electrolytes, glucose, liver transaminases, creatine kinase (CK), lactate, ammonia, and urinalysis may be useful. In some cases, electromyography (EMG) and routine muscle biopsy may provide valuable information (see Table 1; Table 2 ), but they are often normal. The forearm ischemic exercise test has been reviewed elsewhere and may be helpful as well, particularly if GSD type V is suspected. Further diagnostic tests such as urine organic acids, plasma amino acids, and plasma acylcarnitine profile may provide more specific results, allowing the clinician to approach more costly confirmatory testing, including enzyme and/or DNA analysis on leukocytes, fibroblasts, and/or liver, in a rational, potentially cost-effective manner (see Table 2 ).
Disorder | Blood, Urine (u) | Cultured Fibroblasts | Muscle Histology | Muscle Enzymology a |
---|---|---|---|---|
GSDs | ||||
GSD II | GAA, Hex 4 (u) | GAA | PAS | GAA, glycogen content |
GSD V | Mutation screen, gene sequencing | N.A. | PAS | Myophosphorylase, glycogen content |
GSD VII | Gene sequencing | N.A. | PAS (occasionally revealing) | PFK, glycogen content (occasionally elevated) |
GSD IX | Gene sequencing (4 genes), phosphorylase kinase (XL only) | N.A. | PAS | PK, glycogen content |
Other GSD | Gene sequencing, if available | N.A. | PAS (occasionally revealing) | Specific enzyme assays, glycogen content (occasionally elevated) |
FATMO Disorders | ||||
CPT II deficiency | Plasma acylcarnitine profile (when symptomatic), mutation screen (limited sensitivity), gene sequencing | CPT II | Lipid myopathy (?) | CPT II |
LCHAD/Tri-functional protein, VLCAD, or MAD deficiency | Plasma acylcarnitine profile, mutation screen (limited sensitivity), gene sequencing, urine organic acids (MAD deficiency) | Enzyme assay, in vitro probe (limited sensitivity) | Lipid myopathy (?) | Specific enzyme assays (not usually needed, given less invasive testing) |
Mitochondrial Disorders | ||||
Respiratory chain defects | Gene sequencing (targeted, limited sensitivity), organic acids (u), plasma lactate (limited sensitivity) | ETC (limited sensitivity) | Ragged red fibers or cytochrome c oxidase deficiency (limited sensitivity) | Respiratory chain/ETC (freshly isolated mitochondria preferred, limited availability) |
Coenzyme Q 10 deficiency | Gene sequencing (limited sensitivity) | ETC (limited sensitivity) | Coenzyme Q 10 , respiratory chain/ETC (freshly isolated mitochondria preferred, limited availability) |
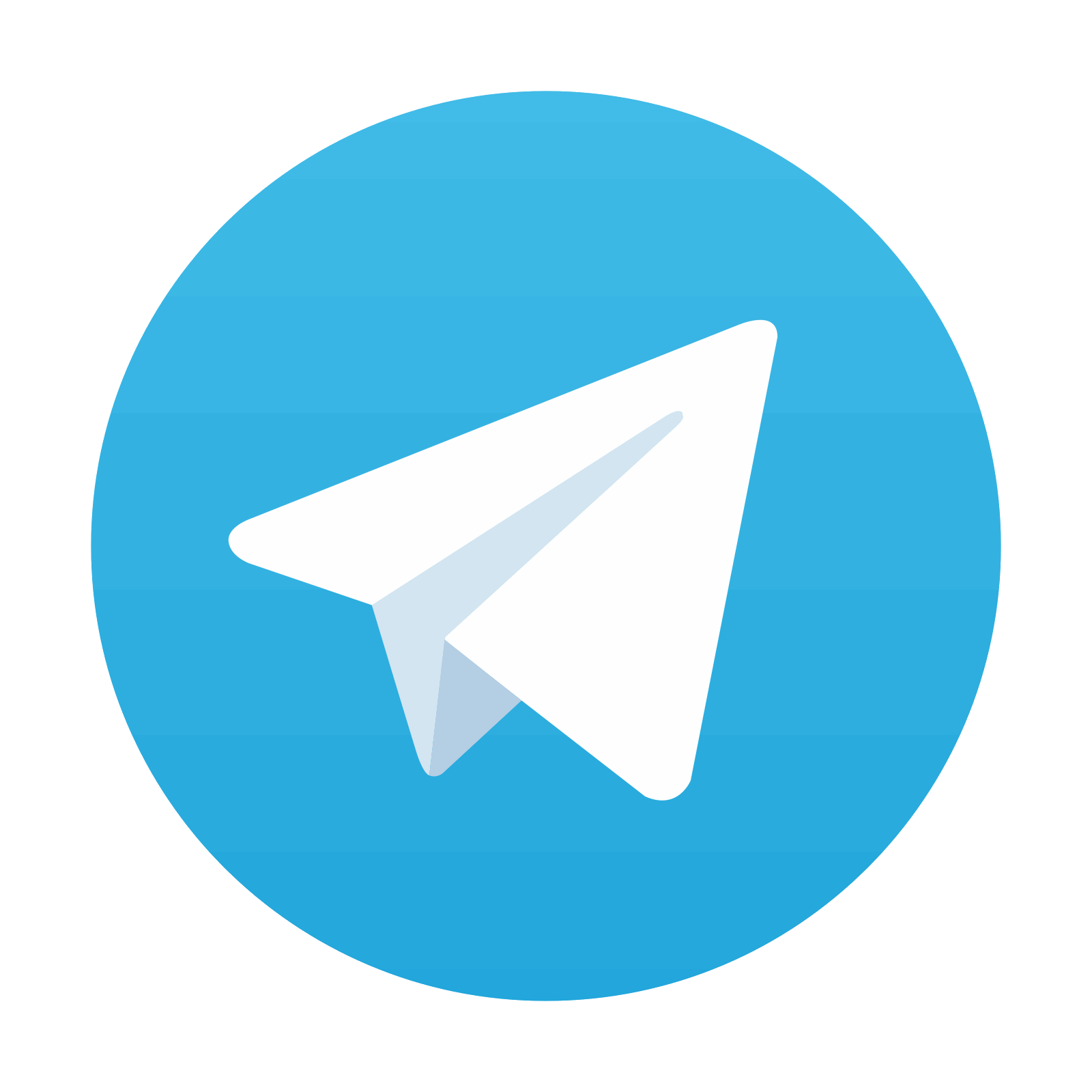
Stay updated, free articles. Join our Telegram channel

Full access? Get Clinical Tree
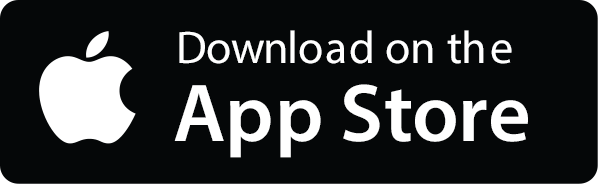
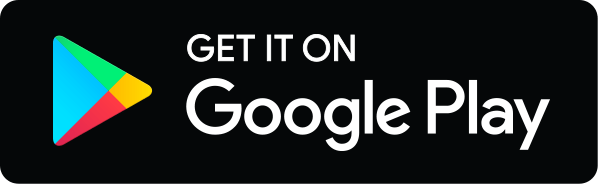
