The innermost connective tissue layer is the endoneurium, which is composed of longitudinally aligned collagen fibres and therefore, plays an important role in protecting the axon from tensile forces (Butler 1991). Encompassing the endoneurial components, axon and Schwann cells is the perineurium, the second layer of connective tissue, whose primary responsibility is to act as a primary barrier to external forces (Lundborg 1988). Collectively, all the contents surrounded by the perineurium form what is known as a nerve fascicle (Topp and Boyd 2006). Collections of nerve fascicles then form a nerve and these are surrounded by the outermost layer of connective tissue, the epineurium (Campbell 2008). The epineurium is regarded as the most resistant connective layer to tensile forces (Sunderland 1978) as it surrounds, protects and cushions the nerve fascicles (Butler 1991). Collectively all three connective tissue layers not only protect the axon, but are also structurally developed to cope with the tensile stresses and compressive forces nerves typically have to endure (Butler 1991). In everyday movements and postures, nerves are subjected to various mechanical stresses (Topp and Boyd 2006), which can elongate, compress or increase strain within the nerve (Shacklock 1995).
The ability of the nervous system to withstand and adapt to the mechanical stresses placed on it, is essential to prevent injury (Shacklock 1995). Alterations in the physical stress to which a biological tissue is subjected, causes predictable responses within the tissue according to Mueller and Maluf’s (2002) Physical Stress Theory. Injury to tissues is caused by excessive physical stress via any of the following mechanisms:
1. High magnitude stress applied to the tissue for a brief duration; spinal cord injury is a typical outcome from this mechanism of injury.
2. Low magnitude stress applied for long duration or repetitively; an example of nerve injury from this particular mechanism of injury is Carpal Tunnel Syndrome at the wrist.
3. Moderate stress applied to a tissue many times. Cubital Tunnel Syndrome at the elbow, for example in a javelin thrower, whereby repetitive high load forces are exerted through the elbow and consequently the ulnar nerve, is an example (Mueller and Maluf 2002).
Biological tissues, such as nerves, have an ideal physical stress range that they can tolerate to maintain homeostasis. This is called the “maintenance stress range” (Mueller and Maluf 2002). Stress levels lower than the maintenance range decreases a tissue’s tolerance to physical stress; for example during immobilisation of a limb, muscle atrophy is a typical bi-product of being in a cast for a prolonged period of time. Contrastingly, when physical stresses are exceedingly higher than the homeostatic stress range, the associated tissues are unable to tolerate these and any subsequent stresses and ultimately become injured (Mueller and Maluf 2002).
The mechanical stresses a nerve is subjected to are not the only factor which must be taken into account with nerve injury as the nature of the surrounding structures a nerve traverses or passes through must also be considered (Butler 1991). The nervous system is surrounded by and comes into contact with many different anatomical structures which may be firm and unyielding, such as the radial nerve in the spiral groove of the humerus, or soft, such as the tibial nerve in the posterior thigh musculature (Butler 1991). Additionally, anatomically narrow passages through which the nerve must pass may also predispose individual nerves to entrapment neuropathies, such as the ulnar nerve in the cubital fossa of the elbow (Bencardino and Rosenberg 2006). The extent of an injury to a nerve is dependant on the mechanism of injury, as traumatic injuries, such as a gun-shot wound (i.e. high magnitude stress), will significantly damage a nerve’s integrity, whilst a low magnitude stress, such as prolonged intermittent compression over a long duration of time, will have less of an impact on the nerve. Nerve injuries were initially classified by Seddon (1943) based on the severity of the injury and the potential for reversibility of the condition (Bencardino and Rosenberg 2006).
Classification of nerve injury
Neurapraxia, axonotmesis and neurotmesis are the three categories of nerve injuries classified by Seddon (1943) each describing different degrees of injury to the nerve’s anatomical structures (Table 8.1). Neurapraxia is the most benign injury and typically results from compression and/or traction (Perlmutter and Apruzzese 1998; Bencardino and Rosenberg 2006). Pathologically, the nerve is intact but is temporarily unable to transmit signals (Campbell 2008) due to injury to the myelin about the nodes of Ranvier (Bencardino and Rosenberg 2006). Consequently, the clinical presentation is that of motor loss, with little sensory involvement and little disturbance of sympathetic innervation (Perlmutter and Apruzzese 1998). With remylenation of the nerve, motor and sensory dysfunction will gradually be restored, usually within 12 weeks (Novak and Mackinnon 2005; Bencardino and Rosenberg 2006). The prognosis for neuropraxia is excellent (Perlmutter and Apruzzese 1998) and complete recovery is expected (Novak and Mackinnon 2005).
Table 8.1 Classification and characteristics of nerve injury
When there is loss of continuity of the axon and myelin sheath, but the majority of the connective tissue structure is maintained, the injury is classified as axonotmesis; the second classification (Seddon 1943). The endoneurium and Schwann sheath are preserved, but injury to the axon is evident, accompanied with secondary Wallerian degeneration (Bencardino and Rosenberg 2006). A clinical finding of significant loss of motor, sensory and autonomic function distal to the injury site is evident (Perlmutter and Apruzzese 1998). Neural regeneration will occur, but at a rate of approximately 1mm per day and over-all the prognosis for recovery is good (Novak and Mackinnon 2005). Stinger Syndrome is also an example of axontmesis, particularly should the injury recur within a short period of time.
The most severe peripheral nerve injury is referred to as neurontmesis and involves complete disruption of the nerve trunk and connective tissue (axons, endoneurial tube, Schwann sheath and epineurium) (Campbell 2008). The prognosis for recovery is poor as there is no potential for nerve regeneration (Bencardino and Rosenberg 2006) and therefore surgical intervention is often required in an attempt to re-establish the continuity of the peripheral nerve (Perlmutter and Apruzzese 1998). Avulsion of the nerve, via stab wounds or gunshot, is a typical example of neurontmesis; it is a rare occurrence in sport.
The majority of nerve injuries in sport will typically involve neurapraxia or axontmesis and therefore prognosis for recovery is generally good.
Assessment of nerve injury
Neural and non-neural tissues should be assessed by the clinician in all patients presenting with pain (Nee and Butler 2006) by means of a comprehensive subjective and physical examination. All the physical examination findings should complement and support the information obtained from the patient via subjective examination (Hall and Elvey 1999). Nee and Butler (2006) proposed numerous clinical features that may be evident in the subjective and physical assessment of patients presenting with peripheral neuropathic pain, such as complaints of tingling, burning and parasthesia in addition to antalgic postures and movement impairments being present. Motor or sensory losses, or both, may also be evident in the assessment of patients presenting with nerve pain. However, when nerve injury is suspected, the assessment of the integrity of the nerve is vital.
Evaluation of the function of the muscles innervated by the injured nerve should be assessed via manual resistance testing to determine the motor function of the nerve (Figure 8.2 and Table 8.2). Likewise, the areas of innervation should be assessed via touch to assess the sensory function of the injured nerve (Dahlin 2008) (Figure 8.3). Knowledge of the myotomes and dermatomes of the upper and lower extremities is important to conduct a thorough assessment of the peripheral nervous system. Nee and Butler (2006) also recommend incorporating neurodynamic tests into the physical assessment to determine the mechanosensitivity of the nervous system.
Table 8.2 Myotomes of the upper and lower limb
Myotomes of the Upper limb | |
Nerve root/nerve | |
C1 | Cervical flexion |
C2 | Cervical flexion |
C3 | Cervical lateral flexion |
C4 | Shoulder elevation |
C5 | Shoulder abduction |
C6 | Elbow flexion |
C7 | Elbow extension |
C8 | Thumb extension |
T1 | Finger abduction |
Myotomes of the Lower limb | |
Nerve root/nerve | |
L1 | Hip flexion |
L2 | Hip adduction |
L3 | Knee extension |
L4 | Ankle dorsi-flexion |
L5 | Great toe extension |
S1 | Ankle plantar flexion |
S2 | Knee flexion |
Neurodynamic testing
Nerves slide and stretch during limb movements to allow for changes in nerve bed length (Babbage et al. 2007) and whilst healthy nerves can tolerate strain and compression, injured or inflamed nerves become sensitive to mechanical stimuli and can inflict pain on movement (Bove et al. 2005).
Neurodynamic tests were developed to evaluate peripheral nerve sensitivity to movement and to infer underlying pathomechanics (Topp and Boyd 2006). To determine if neural tissues contribute to the patient’s symptoms, it is important to move the neural structure in the area in question, without moving the musculoskeletal tissues in the same region; a task attempted by structural differentiation during neurodynamic tests (Shacklock 1995). The concept behind structural differentiation is to move a joint remote to the area where the patient experiences symptoms; should this decrease or increase symptoms, neural involvement into patient symptoms should be suspected. For example, in a patient presenting with posterior thigh pain, moving the head from cervical flexion to extension during the slump test should have no effect on patient symptoms if the pain is of a non-neural origin. However, should the clinician suspect the sciatic nerve is a contributing factor to patient symptoms, moving the cervical spine from flexion to extension should decrease the pain and/or permit a further range of movement at the knee joint.
Neurodynamic tests for the upper and lower extremities have been developed; each one intent on identifying whether a specific nerve is contributing to the symptomatic patient. The termination of the movement during a neurodynamic test is dependant on whether the clinician wants to stop at the point where resistance is experienced by the clinician (Shacklock 1995; Nee and Butler 2006) or to the point where the patient reports onset of pain or sub-maximal pain (Coppieters et al. 2002). Three tests for the upper limb are described below, each focused on examining the integrity of the three primary nerves in the upper extremity; the median, radial and ulnar nerves. For the lower limb, the slump test and straight leg raise tests will be illustrated. In-depth and more detailed clinical neurodynamic tests have been presented by Shacklock (2005).
Upper limb neurodynamic test with median nerve bias
In patients presenting with suspected median nerve pathology whereby symptoms may be localised to the median nerve, such as carpal tunnel syndrome or pronator tunnel syndrome, incorporating a neurodynamic test into the physical examination is essential to determine if the nerve contributes to patient symptoms. To conduct this test, the patient lies supine on the plinth with arms relaxed by the side of the body. Keeping the head in a neutral position, the clinician places a hand over the superior aspect of the shoulder with the aim of depressing the shoulder as the test commences. The clinician then holds the patient’s ipsilateral hand and places the glenohumeral joint in 90° abduction and 90° lateral rotation, the forearm in full supination and the wrist in extension. The clinician then supports the patient’s arm on their thigh with the aim of preventing adduction of the shoulder during the test. Once in this position, the clinician slowly extends the elbow to either onset of resistance or pain (Figure 8.4). To structurally differentiate, the clinician can either ask the patient to execute contralateral side flexion of the head for distal symptoms or the wrist can be moved from extension into flexion for proximal symptoms (Shacklock 2005).
Upper limb neurodynamic test with radial nerve bias
In patients presenting with radial neuropathy such as radial tunnel syndrome, the clinician requires a physical test which can be conducted to determine the sensitivity of the nerve to stretch. To do this, a clinical test was developed to focus specifically on the radial nerve and is conducted as follows: the patient lies supine with arms resting by the side of the body but in a diagonal direction across the plinth. This allows the clinician to apply shoulder depression using the anterior aspect of their hip whilst conducting the test. The patient’s starting position involves placing the elbow at 90° and the wrist and fingers in neutral. The clinician then applies shoulder depression before moving the elbow into extension and the wrist and fingers into flexion whilst internally rotating the glenohumeral joint. Finally, glenohumeral abduction is applied and again the clinician ceases the movement at the point of resistance or pain (Figure 8.5). To structurally differentiate, shoulder depression can be removed for distal symptoms, whilst moving the wrist from flexion to neutral may identify proximal lesions (Shacklock 2005).
Upper limb neurodynamic test with ulnar nerve bias
When symptoms are evident in the anatomical pathway of the ulnar nerve or the lower trunk of the brachial nerve (Shacklock 2005), neurodynamic testing specific to this nerve is important. The testing sequence for an upper limb neurodynamic test with ulnar nerve bias requires the patient to lie supine on the plinth, with the head in a neutral position and the arms relaxed by the side of the body. The clinician prepares for depression of the shoulder as for the median nerve test. The patient’s shoulder joint is abducted slightly whilst the elbow is maintained in extension and the forearm slightly pronated. The wrist and hand of the patient remains in a neutral position, following which, the clinician then commences the test by depressing the shoulder before applying extension to the wrist and fingers and pronation to the forearm. The elbow is then brought into a flexed position; following which, the shoulder is gradually moved into an abducted position (Figure 8.6). The clinician again uses a leg to support the moving limb; thereby making the movement more fluid. Again once resistance or pain is experienced by the clinician or patient respectively, shoulder abduction ceases and the structural differentiation manoeuvre is applied; either contralateral cervical side flexion for distal symptoms or radial deviation for proximal symptoms (Shacklock 2005).
Lower limb neurodynamic test: The slump test
The slump test is used to evaluate the dynamics of the central and peripheral nervous systems from the head, along the spinal cord and sciatic nerve tract and its extensions in the foot (Shacklock 2005). The slump test should be a component of the clinical examination in patients who present with spinal symptoms (Butler 1991) and/or spinal, pelvic and lower limb conditions whereby pain is experienced in the neural distribution of the sciatic nerve and its extensions (Butler 1991; Shacklock 2005). To conduct the slump test, the patient is requested to side on the plinth with the back of the knees to the edge of the plinth and to “sag” the upper body, or bring their shoulder towards their hips, thereby resulting in thoracic flexion. The clinician then applies overpressure at C7 spinous process with the medial aspect of the forearm (Figure 8.7). The patient is instructed to bring their chin to chest, following which the clinician applies dorsi-flexion to the ankle before slowly extending the knee to the point of resistance or pain. To structurally differentiate, the release of cervical flexion and ankle dorsi-flexion are used for distal and proximal lesions respectively.
Lower limb neurodynamic test: the straight leg raise
The straight leg raise (SLR) is a similar neurodynamic test to the slump test, intent in evaluating the integrity of the lumbosacral trunk and plexus, sciatic nerve and its expansion in the leg and foot (Shacklock 2005). The format for the SLR is as follows: the patient lies supine on the plinth with arms resting by the side of the body. The clinician uses one hand to support the posterior calf, just proximal to the ankle joint; whilst the other hand is placed on the anterior aspect of the knee joint to ensure knee extension is maintained throughout. Maintaining this position, the clinician then begins to lift the leg off the bed, thereby conducting hip flexion, moving to the point of resistance or patient pain. Following this the clinician then applies dorsi-flexion to the ankle by way of structurally differentiating (Figure 8.8). An increase in symptoms at this point indicates a neural involvement in the patient’s condition. To ascertain whether the peroneal division of the sciatic nerve is sensitive to stretch, whilst conducting the SLR, the clinician can plantar-flex and invert the ankle whilst moving the ankle into dorsi-flexion and eversion emphasises the tibial nerve (Shacklock 2005).
Neurodynamic tests as treatment tools
A positive neurodynamic test is constituted by a reproduction or increase in symptoms during the test, which is subsequently decreased with the removal of the structural differentiating manoeuvre (Maitland 1985); difference in responses between limbs (Nee and Butler 2006); differences in available range of motion (Coppieters et al. 2002; Nee and Butler 2006); or where there is structural differentiation supporting a neurogenic source (Butler 2000). Whilst all the aforementioned tests have been described from a diagnostic point of view, each of these can be modified and incorporated into treatment plans, should their inclusion as a treatment modality be warranted. Modified versions of the neurodynamic tests can be used in clinical practice in the treatment plan for peripheral nerve injuries and can be specific to each patient. The purpose of utilising neurodynamic tests as treatment tools is to minimise scarring and stretching of the nerve, and maintain or restore normal nerve excursion and function (Wehbé and Schlegel 2004).
Two neural treatment techniques will be considered in this chapter; sliding and tensioning techniques, the principle of which can be applied to any neurodynamic test and considered for use in the treatment plan for neuropathy patients. A “sliding” technique was defined by Coppieters et al. (2009), as a combination of movements that elongate the nerve bed at one joint, whilst simultaneously reducing nerve bed length at an adjacent joint, to counterbalance the elongation. For example, during the neurodynamic test with median nerve bias, instead of having the patient placed in the final testing position, with contralateral side flexion of the cervical spine, the patient would move the elbow into extension whilst simultaneously moving the cervical spine into ipsilateral side flexion (Figure 8.9). The opposing technique is the “tensioning” technique, which the same authors define as the elongation of the nerve bed at two adjacent joints (Coppieters et al. 2009) and again, referring back to the median nerve test, would involve the patient actively extending the elbow whilst simultaneously side flexing the cervical spine into the contralateral direction (Figure 8.10).
The sliding technique causes more longitudinal excursion of a nerve than the tensioning technique (Coppieters et al. 2009), and therefore, from a clinical viewpoint, may be more suitable for highly irritable or acute neural conditions as it still encourages movement of the inflamed nerve, whilst limiting the strain placed on the nerve. A tensioning technique, however, is proposed to induce higher tension within the nerve and therefore less excursion (Coppieters et al. 2009) than a sliding technique and can therefore be considered more aggressive and possibly suitable for less irritable conditions. The clinician should therefore consider the implementation of sliding or tensioning techniques into a treatment plan for the patient presenting with neural symptoms, with the former to be used in acute, irritable conditions and the latter technique for use in the end stage of rehabilitation.
Ultimately, neurodynamic tests can be modified and used in the treatment plan for the neuropathic patient and can also be incorporated into a home exercise programme (Kostopoulos 2004). Typical patient responses to neurodynamic tests as both a diagnostic and treatment tool, of which the patient should be aware, range from reporting feelings of “stretching”, tissue tension, light numbness or a slight increase of pain during the technique; all which should reduce or dissipate on cessation of the test or treatment (Kostopoulos 2004).
Treatment plans for nerve injury
Immediately post-injury, inflammation occurs, thereby rendering injured tissues less capable of tolerating stress compared to their pre-morbid level (Mueller and Maluf 2002). Nerve fibres become sensitive to stretch and low intensity pressure following injury (Dilley et al. 2005). Therefore the importance of protecting the tissues during this acute inflammation stage from subsequent stress cannot be underestimated (Mueller and Maluf 2002). Conservative treatment is the initial recommended strategy in numerous neuropathies not requiring urgent medical attention. Within this category of treatment, several authors suggest incorporating non-steroidal anti-inflammatory drugs (NSAIDs), ice, rest, elimination of the aggravating activity, physical and manual therapy into the conservative treatment plan (McKean 2009; Shapiro and Preston 2009); the primary aim of which is to decrease inflammation and restore nerve function. Brief treatment options will be provided in this section, following the discussion of each specific injury.
Peripheral nerve injury
Neurological conditions are common in athletes (Dimberg and Burns 2005) often dependant on the nature and intensity of the sporting activity (Toth 2009). A clinician may be confronted with signs and symptoms of neurological injuries affecting various aspects of the nervous system, such as spinal nerve roots, peripheral nerve injuries or plexopathies. Possessing the ability to recognise and diagnose injuries specific to sporting activities and then subsequently to treat the injury, is vital to a clinician in sport (Toth 2009) to ensure a rapid return to play for the athlete.
The upper extremity is particularly vulnerable to nerve injury (Dahlin 2008) due to its high mobility (Aldridge et al. 2001) and it is therefore unsurprising that the brachial plexus is the most commonly injured plexus in the body (Wilbourn 2007). The brachial plexus is formed by the ventral rami of the spinal nerves C5 to T1, which enter the posterior cervical triangle between the scalene anterior and medius muscles (Pratt 2005). Superior, middle and inferior nerve trunks of the brachial plexus are then formed by the C5–C6, C7 and C8–T1 nerve roots respectively (Reid and Trent 2002; Pratt, 2005). These trunks divide into anterior and posterior portions behind the clavicle whereby these divisions then unite to form lateral, medial and posterior cords which enter the axilla (Reid and Trent 2002). The end result of the brachial plexus is the formation of peripheral nerves which supply the upper limb (Pratt 2005) (Figure 8.11). The primary peripheral nerves which are subsequently discussed in this chapter are the axillary nerve, long thoracic nerve, suprascapular nerve, ulnar nerve, median nerve and radial nerve; the anatomical locations of which can be viewed in Figure 8.12.
Brachial plexus neuropathy
Stinger Syndrome
One of the most common brachial plexus neuropathy is Stinger Syndrome (Hershman 1990), which is more common in young adults who participate in sport (Unlu et al. 2007) and particularly in contact sports (Wilbourn 2007). The superior trunk of the brachial plexus (C5 and C6) is thought to be the structure injured in this syndrome (Aldridge et al. 2001; Dimberg and Burns 2005; Wilbourn 2007). Tensile overload is one mechanism of injury for the brachial plexus (Hershman 1990), whereby the head and shoulder of the symptomatic side have been forced in opposite directions to each other (Weinstein 1998; Dimberg and Burns 2005) thereby causing stretch and traction of the nerve. Alternatively, another mechanism of injury in Stinger Syndrome is where a compressive force forces the head and neck into the posterolateral corner, causing nerve root compression and thereby injuring the nerve (Weinstein 1998). Finally, injury to the brachial plexus can occur as the result of a direct blow to the plexus region (Weinstein 1998; Reid and Trent 2002).
Symptoms reported for Stinger Syndrome generally involve a single upper extremity (Dimberg and Burns 2005; Wilbourn 2007) with sharp, burning pain and numbness accompanied by short-lived weakness down the arm (Weinstein 1998; Aldridge et al. 2001). Patients who report bilateral or lower extremity symptoms should be treated with caution as spinal cord injury is a potential injury (Dimberg and Burns 2005). An important finding with Stinger Syndrome is that symptoms are unilateral (Wilbourn 2007) and short-lived. Neuropraxia and axontmesis are typically associated with this type of injury.
If the symptoms resolve within minutes and there is no evidence of reduced strength or disrupted movement of the neck, the athlete can be considered for return to play in the same competition (Dimberg and Burns 2005). However, the player should be continuously monitored during the remainder of the competition and re-assessed over the subsequent weeks. Cervical spine rehabilitation should be undertaken in the Stinger Syndrome patient, aiming to protect the injured structures and control pain and inflammation in the initial stages, following which flexibility and strength imbalances and deficits should be addressed (Weinstein 1998). Postural abnormalities should also be considered over the course of treating patients with cervical spine and nerve injuries. A treatment strategy for Stinger Syndrome can include NSAIDs, modification of activity, neurodynamic sliding techniques in the acute stage, manual therapy of the cervical spine, range of movement activities for the shoulder and strengthening of the shoulder and neck muscles.
Thoracic outlet syndrome
The thoracic outlet region in the upper extremity contains three structures; the subclavian artery, subclavian vein and the brachial plexus, all of which pass under the clavicle and subclavius muscle, before then travelling beneath the pectoralis minor muscle close to its insertion point at the coracoid process, whereafter, the structures enter the axilla space (Atasoy 2004). These neurovascular structures pass through the interscalene triangle; comprising of the anterior and middle scalene muscles and the first rib, whilst en-route to the axilla (Brantigan and Roos 2004) and are considered vulnerable to compression within this area. Restriction of space for the neurovascular structures to pass through within the thoracic outlet can cause compression of the neural and/or vascular structures, subsequently resulting in symptoms (Crosby and Wehbé 2004). Compression of one or more of the structures can lead to the diagnosis of thoracic outlet syndrome (TOS). The clinical presentation of TOS can be variable, presenting as either a neurological or a vascular condition, or both. However, with Brantigan and Roos (2004) reporting 98% of cases to be neurological in origin and Crosby and Wehbé (2004) agreeing that the majority of patients have neurogenic symptoms, for the purpose of this section, TOS will be discussed in relation to the brachial plexus only.
TOS can develop insidiously, or as a consequence of a previous traumatic injury, such as whiplash or a similar such trauma, which ultimately causes chronic muscle spasm within the neck or shoulder (Brantigan and Roos 2004). Whilst the initial injury may be considered to be relatively minor, the resultant effect of the injury on the brachial plexus may be delayed and the condition may take considerable time to develop. The patient may complain of arm elevation exacerbating symptoms, such as shoulder press or serving in tennis, whilst exercise can induce a similar response, but only following cessation of the exercise, rather than during it (Brantigan and Roos 2004). Neck stiffness may also be evident in patients with suspected TOS, and therefore the physical examination should include a comprehensive assessment of the neck, shoulder and the upper extremity. Typically evident in patients with neurogenic TOS, is the reproduction of symptoms when the clinician places mild to moderate pressure over the brachial plexus in the supraclavicular fossa of the symptomatic side (Brantigan and Roos 2004). Brantigan and Roos (2004) recommend incorporating the EAST (elevated arm stress test) into the clinical examination to diagnose TOS as few individuals with this condition are actually able to complete the test.
In the majority of cases, conservative treatment will alleviate symptoms and address the cause of TOS unless there is significant neural loss or vascular compression (Crosby and Wehbé 2004). Incorporation of NSAIDs, postural control including during sleep, manual therapy such as thoracic and first rib mobilisation and muscle and nerve gliding exercises into the treatment plan should be undertaken for patients with TOS (Crosby and Wehbé 2004). Exercise therapy such as neck side flexion, neck rotation, chin tucks, shoulder shrugs and shoulder circles to name but a few, can also be beneficial for this condition (Crosby and Wehbé 2004). Crosby and Wehbé (2004) present a comprehensive overview of treatment strategies for TOS.
Axillary nerve
The axillary nerve is the terminal branch of the posterior cord of the brachial plexus, receiving its contributions from C5 and C6 nerve roots (Bencardino and Rosenberg 2006). Axillary nerve injury is a well-known complication of anterior glenohumeral dislocation (Perlmutter and Apruzzese 1998; Goslin and Krivickas 1999) evident in 9–18% of this injury (Perlmutter and Apruzzese 1998). The close proximity of the anatomical course of the axillary nerve to the shoulder joint increases its susceptibility to injury, secondary to shoulder dislocation. The nerve travels inferior to the humeral head before passing through the quadrangular space, where it then wraps horizontally around the posterior aspect of the surgical neck of the humerus (Pratt 2005). It then enters the deltoid muscle which it innervates, along with teres minor (Goslin and Krivickas 1999).
Glenohumeral dislocation typically occurs in an inferior-anterior direction (Owens and Itamura 2000) whereby on dislocation, the humeral head moves into the infra-articular fossa (Pratt 2005). As a consequence of the forced, abrupt movement of the humeral head, a traction force is placed on the axillary nerve (Perlmutter and Apruzzese 1998) resulting in stretching of the nerve around the humeral head and tension propagating to the infraclavicular brachial plexus (Pratt 2005); the overall outcome resulting in nerve injury (Pratt 2005). Blunt trauma to the anterior/lateral aspect of the shoulder, typically seen in tackling during rugby, whereby a compressive force is applied to the axillary nerve is another common mechanism of injury for this structure (Perlmutter and Apruzzese 1998).
Injury to the axillary nerve with shoulder dislocations may go unnoticed initially as the bony injury may dominate the clinical picture (Perlmutter and Apruzzese 1998) and consequently for an athlete participating in contact sports, a thorough neurological examination with shoulder dislocation should be undertaken (Perlmutter and Apruzzese, 1998). A well-defined patch of sensory loss over the lateral shoulder, in addition to weakness in shoulder abduction (deltoid) and external rotation (teres minor) are typical clinical reporting for axillary neuropathy (Perlmutter and Apruzzese 1998; Goslin and Krivickas 1999). Neurological complications following shoulder dislocation, of the brachial plexus and axillary nerve are enhanced in patients >50 years or if the shoulder remains dislocated for greater than 12 hours (Pratt 2005).
Restoring full range of motion at the shoulder is a priority in the conservative treatment for the axillary nerve (Perlmutter and Apruzzese 1998; Goslin and Krivickas 1999. NSAIDs and analgesics will assist with pain and inflammation control during the acute stage. Strengthening exercises for the rotator cuff and periscapular muscles should also be undertaken (Aldridge et al. 2001) in addition to nerve gliding exercises.
Long thoracic nerve
The long thoracic nerve arises from the ventral rami of C5–C7 spinal nerves (Goslin and Krivickas 1999) and innervates exclusively the serratus anterior muscle (Goslin and Krivickas 1999; Aldridge et al. 2001; Pratt 2005). The relative immobility of the long thoracic nerve is a primary factor in the occurrence of injury to this structure, as it is anchored at regular, short intervals throughout it’s anatomical course (Pratt 2005), particularly at the scalene medius and serratus anterior muscles (Goslin and Krivickas 1999). Consequently, traction or stretch of the long thoracic nerve, such as with extreme excursion of the shoulder girdle, is a common mechanism of injury for this structure (Goslin and Krivickas 1999; Pratt 2005). Palsy of this nerve has been associated with repetitive motion or muscular hypertrophy in sport (Toth et al. 2005). One case reported an elite marksman presenting with long thoracic neuropathy, due, according to the author, to the positional stress imposed during repetitive shooting postures whilst holding the gun (Woodhead 1985). Maintaining static postures that keep the shoulder girdle elevated for long periods is an alternate way in which injury to the long thoracic nerve occurs (Goslin and Krivickas 1999; Pratt 2005). This is evident in patients with Saturday night palsy (Pratt 2005), which originates from patients falling asleep on a chair with the shoulder draped over the back of a chair, thereby subjecting the long thoracic nerve to prolonged stretching. Due to the relative superficiality of the nerve, it is also susceptible to external compression (Pratt 2005).
Scapula winging is the most prevalent clinical finding associated with injury to the long thoracic nerve (Aldridge et al. 2001; Goslin and Krivickas 1999) as a result of weakness of the serratus anterior muscle (Owens and Itamura 2002). This clinical finding is best demonstrated by having the patient perform a push-up against a wall (Owens and Itamura 2002). An ache or burning pain in the posterior shoulder with associated weakness in arm elevation and abduction is an early finding in patients with long thoracic nerve pathology (Owens and Itamura 2002), in addition to them having difficulty performing overhead activities (Aldridge et al. 2001), possibly due to serratus anterior being unable to efficiently laterally rotate the scapula during shoulder elevation.
Treatment strategies for long thoracic neuropathy involve rest and avoidance or modification of the aggravating activity (Goslin and Krivickas 1999), NSAIDs and physical therapy. Exercises aiming to fix the scapula against the thorax are advised to prevent further overstretching of the nerve or scapula winging (Goslin and Krivickas 1999). Maintaining shoulder range of motion (Aldridge et al. 2001) and increasing the strength of trapezius and the rhomboids (Goslin and Krivickas 1999) should be additional aims for the treatment plan.
Suprascapular nerve
The suprascapular nerve originates from the superior nerve trunk (C5–C6 nerve roots) at Erbs’ point (Aldridge et al. 2001; Pratt 2005; Bencardino and Rosenberg 2006) and is responsible for innervating supraspinatous and infraspinatous muscles, which abduct and laterally rotate the shoulder respectively (Goslin and Krivickas 1999; Pratt 2005). The suprascapular nerve is vulnerable to entrapment (Goslin and Krivickas 1999) as it passes through the suprascapular foramen before curving around the spinoglenoid notch; both anatomical points of nerve entrapment (Pratt 2005).
Injury to this structure can occur as a result of acute stretching, a blow to the superior aspect of the shoulder (Owens and Itamura 2001) whereby the shoulder is forcibly depressed (Goslin and Krivickas 1999), or can be of insidious onset (Aldridge et al. 2001). Repetitive scapular motion, such as that which occurs during overhead activities in tennis or badminton can stretch and compress the suprascapular nerve and induce entrapment neuropathy (Bencadino and Rosenberg 2006). Patients with suprascapular neuropathy may complain of pain at the superior border of the scapula (Goslin and Krivickas 1999), which may co-exist with impingement signs and symptoms (Owens and Itamura 2002) or weakness (Goslin and Krivickas 1999). Weakness and pain during shoulder abduction and lateral rotation may be evident, due to dennervation of the supraspinatous and infraspinatous muscles respectively (Goslin and Krivickas 1999; Aldridge et al. 2001). Point tenderness over the area of nerve compression is evident in patients with entrapment of the nerve whilst horizontal adduction of the shoulder may elicit patient symptoms as the tension on the nerve is increased with this manoeuvre (Aldridge et al. 2001).
The management for injury to the suprascapular nerve should involve modifying or ceasing the aggravating activity (Goslin and Krivickas 1999; Aldridge et al. 2001), whilst incorporating NSAIDs and scapular stabilisation exercises into the rehabilitation programme (Aldridge et al. 2001). Restoring full range of motion at the shoulder, increasing strength of the scapular stabilisers and rotator cuff muscles (Goslin and Krivickas 1999) are additional aims for a treatment programme for this neuropathy.
Ulnar nerve
The ulnar nerve is the end point of the medial cord of the brachial plexus, composed of fibres from C8 and T1 nerve roots (Rokito et al. 1996; Aldridge et al. 2001; Bencardino and Rosenberg 2006) and is extremely mobile at the elbow joint. It is therefore susceptible to direct trauma, compression and traction; to name but a few causes of injury to this structure (Izzi et al. 2001).
Cubital tunnel syndrome
Cubital tunnel syndrome is the second most common neuropathy in the upper extremity (Bencardino and Rosenberg 2006) and the commonest entrapment neuropathy at the elbow (Salama and Stanley 2008), being responsible for entrapment of the ulnar nerve. The potential for ulnar nerve compression at the cubital tunnel is unsurprising considering its anatomical arrangement (Pratt 2005). The arcuate ligament and medial collateral ligament of the elbow form the roof and floor aspect of the tunnel respectively (Pratt 2005; Bencardino and Rosenberg 2006). During elbow flexion the points of attachments for the structures of the cubital tunnel are pulled further apart, resulting in tightening of both the floor and roof of the tunnel. In addition, during forearm flexion, the trochlea of the humerus rotates under the medial collateral ligament and elevates the floor of the tunnel, culminating in decreased space for the ulnar nerve to glide and move (Pratt 2005). Therefore, it is unsurprising that this syndrome is prevalent in throwing athletes (Izzi et al. 2001), many of whom undertake repetitive elbow joint movements, with forced extension, such as seen in pitching a baseball. During repetitive, high-velocity throwing, athletes, particularly in those who demonstrate excessive laxity at the elbow (Badia and Stennett; Rokito et al. 1996), increased traction is placed on the nerve during the movement, all of which culminates in an increased susceptibility to ulnar neuropathy (Rokito et al. 1996).
Clinically, patients with cubital tunnel syndrome may present with diminished sensation in the ulnar aspect of the fourth finger and all of the fifth finger (Izzi et al. 2001; Salama and Stanley 2008) and elbow pain radiating to the hand with sensory symptoms, particularly at night, when the elbow is maintained in flexion for long periods of time during sleep (Bencardino and Rosenberg 2006). Weakness in the finger abductors, and thumb adductor or struggling to maintain a powerful grip may be additional clinical complaints (Izzi et al. 2001; Shapiro and Preston 2009). In the overhead athlete, pain along the medial joint line may be the first clinical sign of ulnar neuropathy or complaints of a popping or snapping sensation at the elbow during flexion and extension of the forearm, due to subluxation of the ulnar nerve, may also be reported (Aldridge et al. 2001).
In the early stages of nerve compression, provocative testing may be the only positive finding (Novak and Mackinnon 2005). A positive Tinel’s sign should be performed at the entrapment site and along the course of the nerve whereby reproduction of patient symptoms in the relevant neural distribution constitutes a positive test. Tapping the ulnar nerve behind the medial epicondyle is a Tinel’s test for the ulnar nerve at the elbow (Salama and Stanley 2008). Positional and provocation tests should also be undertaken in potential entrapment neuropathy, whereby each test is held for one minute, and are deemed positive if there is alteration in sensations in the correct neural distribution (Table 8.3) (Novak and Mackinnon 2005). It is important in cubital tunnel syndrome to differentially diagnose the possibility of thoracic outlet syndrome, lower cervical neuropathy or compressive ulnar neuropathy at the wrist (Bencardino and Rosenberg 2006). Medial epicondylitis, medial ligament laxity and ulnar neuritis all require differential diagnosis by the clinician (Rokito et al. 1996).
Table 8.3 Positive tests for nerve compression in the upper extremity
Nerve | Site of entrapment | Provocation test |
Radial nerve | Distal forearm | Forearm pronation with wrist ulnar deviation. Pressure over tendinous junction of extensor carpi radialis and brachioradialis |
Ulnar nerve | Cubital tunnel | Elbow flexion and pressure on ulnar nerve at the cubital tunnel region |
Median Nerve | Proximal forearm | Forearm supination with pressure in the region of pronator teres |
Carpal tunnel | Wrist flexion and/or extension with pressure proximal to carpal tunnel | |
Adapted from Novak and Mackinnon (2005). |
Conservative treatment of cubital tunnel syndrome can include avoidance of the aggravating activity (Badia and Stennett 2006), NSAIDs, altering throwing technique, manual therapy, nerve gliding (Kostopoulos 2004) and exercise therapy such as a progressive strengthening exercise programme (Badia and Stennett 2006). A night splint may be indicated in early cases, particularly if the patient sleeps prone, with the elbow tucked under a pillow whereby local pressure can be placed on the ulnar nerve. In this position, traction can also be placed on the nerve, if the elbow remains flexed and shoulder abducted during the sleeping posture (Izzi et al. 2001; Salama and Stanley 2008). Night splints should only be utilised if the patient complains of symptoms whilst sleeping.
Radial nerve
The radial nerve is the larger branch of the posterior cord of the brachial plexus (Bencardino and Rosenberg 2006), with part of its anatomical course running obliquely around the posterior shaft of the humerus in the spiral groove (Pratt 2005), making it therefore very vulnerable to injury following fracture of the humerus. Radial nerve injury can occur secondary to humeral shaft fracture, as a result of the inappropriate use of axillary crutches or due to entrapment at the elbow (Bencardino and Rosenberg 2006). The radial nerve also has the potential to become entrapped in callus formation during the healing process following humeral fracture (Pratt 2005).
Radial tunnel syndrome
Compression of the radial nerve at the elbow is referred to as radial tunnel syndrome and more commonly affects the posterior interosseus nerve (Bencardino and Rosenberg 2006). It is commonly seen in racquet sport athletes or swimmers where repetitive pronation and supination occurs (Izzi et al. 2001; Bencardino and Rosenberg 2006). The posterior interosseus nerve is vulnerable to compression at various sites within the radial tunnel (Bencardino and Rosenberg 2006), of which the arcade of Frohse is one of the hazardous anatomical structures that can compress the nerve at the elbow (Pratt 2005). The arcade of Frohse is a fibrous arch formed by the proximal portion of the superficial head of the supinator muscle (Pratt 2005; Bencardino and Rosenberg 2006) and under which the radial nerve passes (Bencardino and Rosenberg 2006).
Clinically, a patient with compression of the radial nerve at the elbow will present with poorly localised pain to the antero-lateral aspect of the elbow, which can be provoked by manoeuvers that stretch or compress the nerve (Izzi et al. 2001). Tenderness over the radial nerve along the radial tunnel, pain on resisted supination and a positive Tinels’ sign over the radial forearm are factors that may be present on physical examination (Bencardino and Rosenberg 2006). Differential diagnosis for lateral epicondylitis of the elbow is vital as radial tunnel syndrome can masquerade as or co-exist with this condition (Bencardino and Rosenberg 2006; Salama and Stanley 2008). Management of radial tunnel syndrome should incorporate pain-relieving modalities such as NSAIDs (Izzi et al. 2001; Salama and Stanley 2008), nerve gliding exercises (Badia and Stennett 2006), activity modification and splinting (Izzi et al. 2001; Salama and Stanley 2008).
Median nerve
The median nerve is formed by contributions from the medial and lateral cords of the brachial plexus (Pratt 2005; Bencardino and Rosenberg 2006) containing motor and sensory fibres from the C5–T1 nerve roots (Bencardino and Rosenberg 2006). Whilst carpal tunnel syndrome is the most common nerve entrapment of the upper extremity (Bencardio and Rosenberg 2006), it is more common in the general population as opposed to athletes, as the latter typically suffer from nerve compression at the elbow (Izzi et al. 2001).
Pronator teres syndrome
Pronator teres syndrome is compression of the median nerve (Izzi et al. 2001; Kostopoulos 2004) and is the most common cause of median nerve entrapment at the elbow (Bencardino and Rosenberg 2006). It is more prevalent in athletes as opposed to the general population (Badia and Stennett 2006) with hypertrophy of the pronator teres cited as one of the potential inflictors of compression on the nerve (Izzi et al. 2001). External compression on the forearm muscles is another source of medial neuropathy discussed by Badia and Stennett (2006) whereby one of the authors reported medial nerve compression in an acrobat who wrapped a curtain of material around the forearm as part of an acrobatic routine during numerous performances, thereby repetitively compressing the median nerve and ultimately inflicting injury.
The clinical features of pronator teres syndrome are often vague (Kostopolous 2004). However, patients with this syndrome may complain of volar arm pain, with or without hand pain, which may be exacerbated by repeated forearm pronation and wrist flexion (Izzi et al. 2001). Numbness in the median nerve distribution with repetitive pronation and supination, but not elbow flexion and extension, is another indicator of this neuropathy (Bencardino and Rosenberg 2006). Weakness in resisted forearm pronation, wrist flexion and radial deviation are additional clinical presentations with this condition, in additional to thenar atrophy and an inability to oppose or flex the thumb (Bencardino and Rosenberg 2006). Tenderness on palpation can be evident over the pronator muscle (Izzi et al. 2001).
Patients with pronator teres syndrome are advised to abstain from the aggravating activity or modify their choice of equipment or technique (Badia and Stennett; Salama and Stanley 2008). NSAIDs, rest (Salama and Stanley 2008), nerve gliding exercises and stretching of the pronator teres muscle are some of the treatment strategies available to clinicians for this neuropathy. Splinting is another option presented by Salama and Stanley (2008) for treatment of this condition.
Carpal tunnel syndrome
Carpal tunnel syndrome (CTS) is the most common entrapment neuropathy in the upper body (Shapiro and Preston 2009), involving compression of the median nerve as it traverses through the wrist at the carpal tunnel (Rempel and Diao 2004) and presents relatively frequent in athletes (Aldridge et al. 2001). The carpal tunnel is composed of the stiff carpal bones of the wrist, which make up the floor and walls of the tunnel and the flexor retinaculum, which acts as the roof of the tunnel (Kostopoulos 2004). Nine tendons, the medial nerve, synovium and radial and ulnar bursae occupy the carpal tunnel (Rempel and Diao 2004). It is therefore quite apparent how this particular area may be subjected to entrapment neuropathy due to the limited space within the tunnel, and the high volume of structures occupying this space.
CTS is considered to develop as the result of repetitive wrist use and commonly presents in gripping athletes, such as archery, racquet and throwing athletes (Izzi et al. 2001). The syndrome, according to Kostopoulos (2004) is defined by the signs and symptoms of the median nerve at the wrist, as sensory loss and parasthesia are commonly present in the distribution of the median nerve with this condition (Shapiro and Preston 2009). A thorough investigation of the wrist and cervical spine should be undertaken to eliminate the potential for double crush syndrome (Kostopoulos 2004). Clinically, patients complain of pain in the wrist and hand with parasthesia evident in the lateral three and a half fingers (Izzi et al. 2001) in addition to reporting nocturnal pain (Aldridge et al. 2001; Izzi et al. 2001). In chronic conditions, symptoms may be reported above the carpal region, as far distal as the cervical spine (Kostopoulos 2004). Abductor pollicis weakness is the most common motor weakness associated with CTS (Izzi et al. 2001) and a diminished grip strength may be evident when compared to the asymptomatic side (Aldridge et al. 2001). A positive Tinel’s sign or Phalens test is indicative of CTS (Aldridge et al. 2001; Shapiro and Preston 2009) and thenar atrophy may be observed in advanced cases of CTS (Aldridge et al. 2001). Symptoms can be exacerbated during sleep if the wrist is maintained in a flexed or extended position (Shapiro and Preston 2009).
Conservative treatment of CTS can include NSAIDs, active rest, modification of the aggravating activity, tendon and nerve gliding (Aldridge et al. 2001; Izzi et al. 2001; Kostopoulos 2004) and exercise therapy. Splinting of the wrist is another treatment option for clinicians for CTS management.
Lower limb nerve injuries
Comprising of the lumbar and sacral plexuses, the lumbosacral plexus originates from L1, L2, L3 and, in part, L4 anterior primary rami (APR) (Wilbourn 2007). The remaining APR of L4 fuses with L5 APR to form the lumbosacral trunk, which subsequently contributes to the formation of the sacral plexus; an entity responsible for providing sensation to the majority of the lower limb (Wilbourn 2007). The lower branch of L2, all of L3 and the upper branch of L4 terminate by dividing into anterior and posterior divisions; the former forming the obturator nerve and the latter forming the femoral nerve. The superior and inferior gluteal nerves are formed by the posterior divisions of L4, L5 and S1, and the posterior divisions of L5, S1 and S2, respectively (Figure 8.13). The sacral plexus provides sensation to the gluteal region and, with the exception of the anterolateral thigh and a lengthy strip of medial leg, the lower limb. It innervates the pelvic floor muscles, gluteals and tensor fascia latae, hamstrings and all the muscles of the leg and foot (Wilbourn 2007). Lumbosacral plexopathies are infrequent, compared to brachial plexus injury, possibly due in part to the protective anatomical arrangement of the pelvis and surrounding musculature, in addition to the nerves being associated with less mobile structures (Wilbourn 2007). The anatomical arrangement of the sciatic, tibial and peroneal nerves can be viewed from Figure 8.14 and are subsequently discussed in relation to injury and treatment, particularly in sporting populations.
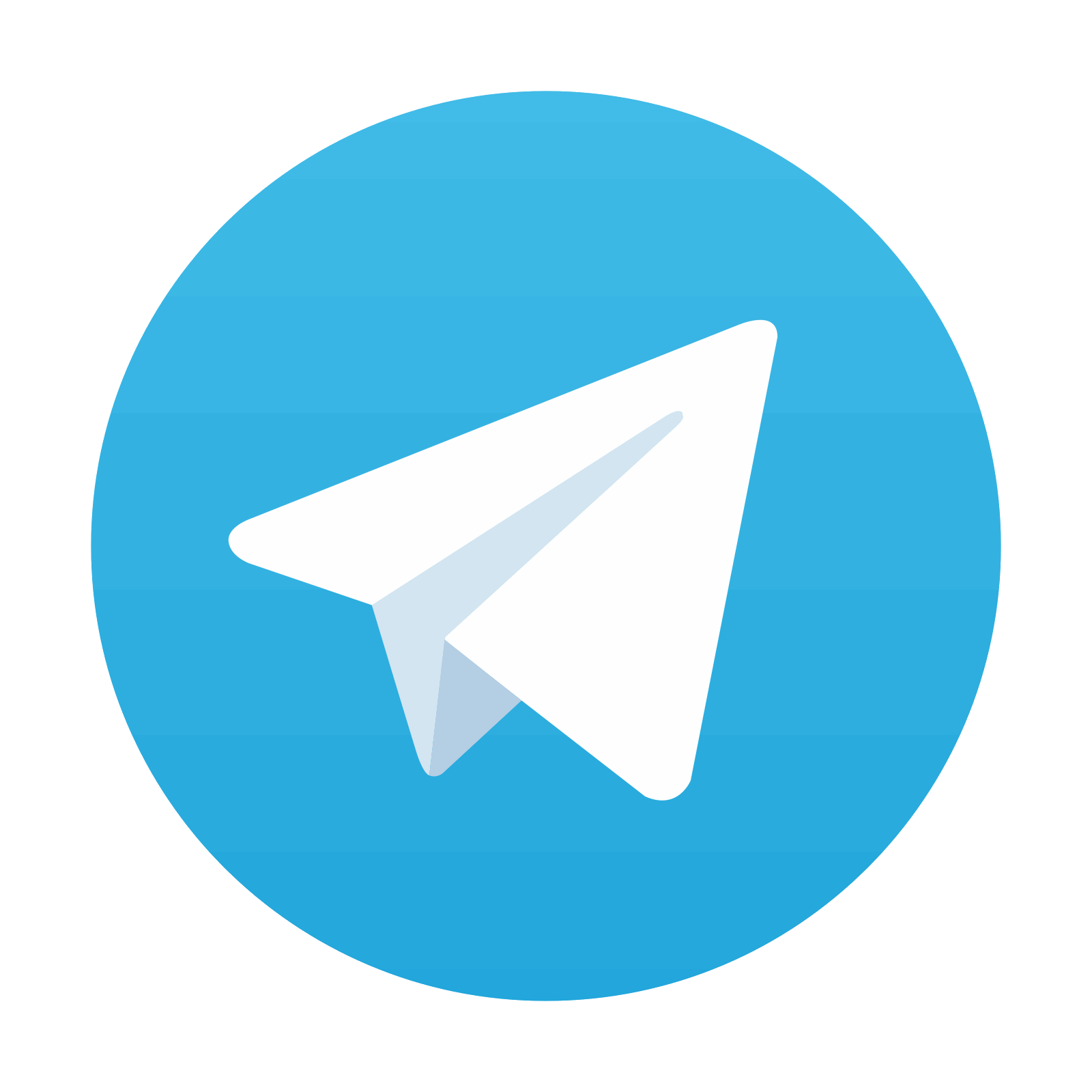
Stay updated, free articles. Join our Telegram channel

Full access? Get Clinical Tree
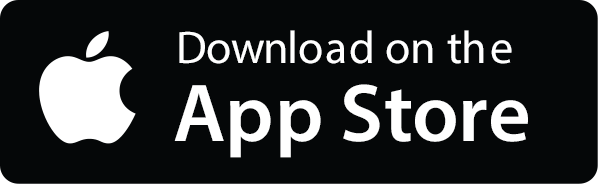
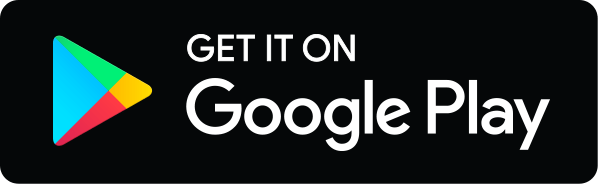