Chapter 23 Osteochondral Autograft Plug Transfer
The treatment of symptomatic focal articular cartilage defects continues to present a clinical dilemma for many orthopedists. Surgical approaches to this problem can be categorized into those that attempt to resurface focal defects through tissue repair or regeneration and those that reconstitute articular cartilage surfaces through transplantation of intact osteochondral grafts. The former group includes repair strategies such as marrow stimulation and cell-based therapies such as implantation of chondrocytes expanded ex vivo. The results of these resurfacing procedures have been associated with tissue repair predominated by fibrocartilage, which has been shown to have inferior wear characteristics compared with hyaline tissue.61 Osteochondral autograft plug transfer (OAT) involves the direct transplantation of osteochondral segments from less loaded regions of cartilage to areas with symptomatic focal defects. Despite concerns of donor site morbidity and limited availability, this method has been demonstrated to reliably restore native hyaline cartilage architecture and the underlying subchondral bone.
Early work on autologous hyaline cartilage transplantation to the femoral or tibial condyles involved direct transfer of patellar osteochondral grafts. The procedure was first described using a pedunculated patellar graft by d’Aubigné in 1945; in more recent times, Campanacci et al reported on a case series of 19 patients who underwent similar transfer of a free patellar graft.13 Indications included primarily giant cell tumors and involved resection of almost the entire osteochondral segment of the patella and screw fixation to the tibial or femoral condyle. Attempts to restore native articular cartilage contour were made through careful orientation of the patellar graft, but with obvious donor–recipient mismatches. Patients were noted to have overall success, with the main complication being loss of range of motion.
Later reports described the transfer of autogenous osteochondral fragments for treatment of osteochondritis dissecans of the knee. Yamashita et al in 1985 described two patients who underwent graft harvest from the superomedial femoral trochlea in a region which “in extension was in contact with neither patella nor meniscus.”90 Donor sites were filled with iliac crest bone graft, and all segments were fixed using orthogonal mini-cancellous screws. Second-look arthrotomy for screw removal revealed macroscopically intact hyaline cartilage with mild irregularities at the interface between graft and native tissue. Slight surface contour irregularities were noted at both donor and graft sites, but with negligible clinical sequelae. Outerbridge et al in 1995 described the transfer of an osteochondral graft from the lateral facet of the patella to repair a large osteochondral defect in the ipsilateral femoral condyle in 10 patients.68 A manual press-fit technique was used for graft fixation. Preoperative and postoperative function was assessed using the Cincinnati Knee Score; an average improvement from 43 points (range, 24 to 64) to 93 points (range, 79 to 100) was reported. All patients were satisfied with the procedure, and 70% were able to resume full, unrestricted activity. Success was offset by increased patellofemoral pain and progression of degeneration of the medial facet of the patella. Second-look arthroscopy revealed solid graft fixation and intact surface hyaline cartilage.
Indications
Numerous treatment algorithms have been proposed for the management of articular cartilage lesions.15,77,82 Smaller lesions (<10 mm) are typically managed with simple débridement, citing the limited increase in biomechanical loading and rim stresses around the edges of the defect.25 Primary treatment options typically include marrow stimulation techniques such as microfracture, which has been demonstrated to provide good symptomatic relief and restoration of function in defects with intact edges and good containment.62,84 However, more recent studies have raised concerns about the durability of fibrous/fibrocartilaginous repair tissue.61 Larger lesions (surface area >200 mm2) and those with defects extending into subchondral bone represent a more complex scenario.
OAT is indicated for the management of focal articular cartilage lesions of the medial or lateral femoral condyles, the trochlear groove, and the patella. Reports of retrograde methods to address tibial plateau lesions have been discussed but are not clinically common. Indications as far as size considerations are concerned have traditionally been described as ranging from 10- to 25-mm-diameter lesions to 150- to 250-mm2-area lesions.14,55 Overall, the optimal lesion size indicated for treatment includes symptomatic lesions that measure from 50 to 250 mm2. Smaller osteochondritis dissecan (OCD) lesions and osteochondral fractures represent a good indication for OAT in that restoration of both the surface hyaline and the underlying subchondral bone can be successfully addressed. A variation on the typical OAT procedure has been described for the treatment of unstable OCD lesions. Rather than conventional hardware fixation, single or multiple osteochondral plugs may be used as biologic pegs to secure OCD lesions following débridement and backfill of underlying fibrous tissue.60,63 Age is a consideration; patients younger than 50 years of age with an absence of degenerative pathology are indicated for OAT, depending on the quality and availability of donor tissue. Other patient factors include compliance with postoperative weight-bearing restrictions.
Technical Considerations
In 1982, Osterman and colleagues reported on a series of animal model experiments in rats, in which different strategies were taken toward reconstituting medial femoral condyle defects.51 Fixation of a loose osteochondral fragment with a bone peg or with tissue adhesive was studied along with comparison of fresh-frozen allografts versus autogenous grafts harvested from the lateral femoral condyle. The authors concluded that precise reconstruction of the articular surface was essential because failures were seen in cases where restoration of joint surface congruity was inadequate. Subsequent studies have expanded on our understanding of the factors influencing outcomes from OAT. These include topography, contact pressures, fill pattern, depth of insertion, graft harvest, and graft insertion. Although advances in instrumentation and surgical techniques are essential and important, challenges remain, and several technique-related resurfacing factors must be addressed.
Topography
In 1991, Ateshian et al described an analytic stereophotogrammetry technique through which the articular surface topography and the cartilage thickness of the knee joint were mapped (Fig. 23-1).3 In a later study, Bartz et al described a method to be used for computerized matching of the topography of donor and recipient sites for OAT, allowing analysis of which commonly used donor site is best matched to a specific defect.7 They concluded that cartilage defects in the weight-bearing regions of the medial and lateral femoral condyles are best treated with grafts taken from the most inferior parts of the superomedial and superolateral borders of the trochlea (Fig. 23-2). Similarly, lesions in the saddle-shaped trochlea may be addressed with grafts from the intercondylar notch.1
It has been proposed that matching of cartilage thickness between donor and recipient sites is important, and avoiding underlying subchondral step-off may result in a better loading response.87 Although no biomechanical or animal studies have proven this to be the case, the structural composition of cartilage and the means by which contact pressures are distributed support this theory. Hydrostatic pressures within cartilage and the rate of increase with joint loading can be expected to vary with different cartilage thicknesses. Studies have shown that cartilage thickness is correlated with local joint load, with central condylar thickness up to 3.65 mm compared with sulcus terminalis thickness of as little as 0.22 mm.85 Typical graft sites measure 1.2 to 1.6 mm in cartilage thickness compared with condylar thicknesses in the 1.6- to 2.0-mm range.
The concept of split line orientation of surface cartilage may also merit consideration during osteochondral autografting. In 1898, Hultkrantz demonstrated a distinct orientation to collagen fibers along the articular cartilage surface, postulating that this was related to directional variation in stiffness and strength. Benninghoff in 1925 further described the microarchitecture of articular cartilage to include collagen fibers oriented perpendicular to the joint surface in the deep layer, with parallel orientation superficially. Below et al mapped the split line pattern of articular cartilage at the distal femur, demonstrating a characteristic pattern of collagen fiber orientation (Fig. 23-3).8 Although this may have no significant clinical effect, it is a theoretical concern that deserves consideration.
Contact Pressures
Contact pressures of the patellofemoral articulation have been examined by several investigators.1,21,83 Simonian et al83 reported that commonly used donor sites along the periphery of the femur at the patellofemoral joint and within the intercondylar notch were not completely free of joint loading forces. Ahmad et al1 quantified a patellofemoral contact map exhibiting the differences in contact pressures at various sites along the distal femur, showing lowest contact forces at the superomedial border of the femur, as well as along the most inferior part of the intercondylar notch (Fig. 23-4). Garretson et al21 measured contact pressures under two different loads through the entire range of knee flexion. Contact pressures were noted to be lower along the medial condyle; however, no significant differences were noted along the far medial or far lateral edges of the distal femur. The authors concluded that harvesting of grafts from the medial femoral flare may have less impact on patellofemoral biomechanics, but is limited by the fact that the medial condyle is narrower and more sloped than the lateral condyle. They recommend that grafts 5 mm in diameter or smaller should be harvested from the medial femoral flare, and that larger grafts be harvested from the lateral femoral flare. The consensus from these studies is that although all articular surfaces bear some load, the edges of both medial and lateral flares bear the least force during patellofemoral articulation and may represent optimal surface match contours for condyle lesions. Depending on the size of the recipient site and the number and size of donor plugs needed, individualized strategies for harvesting may be devised, with the goal of minimizing alterations in patellofemoral biomechanics.
Fill Pattern
Depending on the size and pattern of the lesion, different technical strategies for coverage may be used. Smaller lesions can be covered with a single donor graft, depending upon condyle size and topography. Larger lesions may require multiple grafts. Biomechanical studies of osteochondral defects in cadaveric knees have demonstrated increased rim stress concentration in defects measuring 10 mm or greater in diameter.25 Smaller defects did not demonstrate significant load redistribution. The implication for OAT is that complete fill is not required for adequate reconstitution of lesions. Hangody et al illustrated how multiple osteochondral plugs may be arranged to fill a large defect (Fig. 23-5).32 Given the limited nature of donor plugs, as well as the biomechanical considerations outlined previously, defects are typically tiled with multiple plugs of different sizes with fibrous fill in the intervening gaps. It has been proposed that the gaps between these osteochondral plugs may be filled with hyaline-like tissue, but no evidence indicates that this additional measure results in appreciably improved outcomes.34
Burks et al in 2006 demonstrated that incomplete fill of a larger lesion better preserved the contour of a femoral condyle than of untreated or bone-grafted defects.12 In an ovine model, a 6-mm plug was placed in the center of a 10-mm defect. While surrounded by fibrous tissue, this method better preserved the articular surface and contour compared with unfilled defects. Hyaline cartilage was noted in the central plug, and composite cartilage scores on histologic evaluation were significantly higher in incompletely filled defects than in unfilled defects.
Depth of Insertion
Early concerns over subsidence of osteochondral plugs led to recommendations that osteochondral autograft plugs be placed slightly proud. In 2001, Pearce et al performed a study on sheep comparing grafts placed flush with the joint surface and those placed 2 mm proud.73 Contrary to the authors’ hypothesis, grafts placed proud showed poor bony incorporation evidenced by surrounding fibroplasia and chondroplasia consistent with hypertrophic nonunion attributed to micromotion or shear of the grafts. Grafts placed flush with surrounding cartilage revealed good alignment and continuity, with interstices filled by well-attached repair tissue. In a subsequent series of biomechanical studies, Koh et al studied contact pressures in elevated and countersunk osteochondral plugs placed in porcine knees, as well as grafts inserted at an angle with surface asymmetry.44,45 Contact pressures were markedly elevated (roughly 50% increase in peak pressure) in grafts placed as little as 0.5 or 1 mm above the surface. Countersunk grafts exhibited approximately 10% increases in peak pressures over those placed flush, although still less than defects left unfilled (20% increase). Similarly, angled grafts placed with any part of the graft protruding above the surrounding cartilage resulted in significant increases in peak contact pressures, whereas those placed partially countersunk were similar to plugs placed orthogonally and flush. These results were corroborated in a case series by Nakagawa et al.65 Clinical outcomes and second-look arthroscopy were compared in five patients following OAT; two had proud grafts and three had countersunk grafts. Patients with proud grafts complained of catching sensation about 4 months postoperatively, as well as occasional knee sensations. Second-look arthroscopy showed fibrillation and fissuring around the grafts. Patients with countersunk grafts reported no clinical symptoms and exhibited fibrocartilaginous tissue overlying the grafts with a smooth joint surface. These data clearly demonstrate that although the ideal osteochondral plug placement is flush with surrounding cartilage, there is far greater tolerance for plugs placed slightly countersunk as opposed to elevated. Any prominence of the transferred tissue results in significant elevation of contact pressures and may lead to toggling, clefting, and perimeter breakdown of the graft with degradation of the transplanted cartilage.
Graft Insertion
Fixation of osteochondral autograft plugs is achieved through a press-fit technique. This method allows all-arthroscopic delivery of grafts, as well as arrangement of several grafts within a single defect bed. Several investigators have studied the effects of different parameters on graft stability. Kock et al compared push-in forces for different lengths of plugs, as well as the effects of matching donor to recipient plug depth.41 They found that longer plugs (>12 mm) were more stable when unmatched to recipient site depth, owing to higher frictional forces along the edge of the graft. In contrast, donor plugs that matched recipient site depth (e.g., those that contacted the base of the prepared defect) were more stable when shorter lengths were used. Kordas et al found that grafts with larger diameters withstood greater push-in forces, but that multiple grafts regardless of arrangement (row vs. circle) were less stable than individual plugs.47 Similarly, overlap of multiple plugs with breach of interplug native bone (socket wall) was found to decrease stability.27 It is hypothesized that this decreased stability may be related to less adjacent native articular cartilage and bone available to buttress the graft. Dilatation of the recipient bed has been described as a means of obtaining an optimal press-fit. Shorter dilatation lengths allow for more of a tapered fit between donor plug and recipient site. Similarly, slight oversizing of donor plugs relative to recipient sites increased stability by compensating for extraction blade width. In a rabbit model, Makino et al demonstrated increased stability and better preservation of cartilage thickness when grafts oversized by 1 mm relative to the recipient site were used.54
One disadvantage of the press-fit technique is the force required for graft impaction. Several studies have documented a decrease in chondrocyte viability following impaction, although the clinical relevance of this has yet to be determined.6,10,26,89 Lower forces used to gently seat plugs into well-matched recipient sites may be benign, whereas the markedly increased forces required to compress a graft that would otherwise be left proud may adversely affect chondrocyte viability.71
Graft Harvest
Harvesting of osteochondral autografts has been a focus of several studies, with emphasis on cell viability. Evans et al reported superiority of manual punches over power trephines, with inadvertent skidding, ragged cartilage edges, and separation of cartilage from subchondral bone seen in the latter.19 Chondrocyte viability was also decreased when power trephines were used for graft harvest. Duchow et al, in a study of pull-out strengths with porcine osteochondral autografts harvested using a manual punch, noted that plugs extracted by levering of the plug were less stable than those extracted by turning of the punch.18 They also noted that failure loads were significantly less frequent in 10-mm-long plugs compared with those 15 mm or 20 mm long. Moreover, repeated insertion and extraction led to decreased stability. The cutting profile of manual punches also had an effect on chondrocyte survival, with subtle compression of the articular cartilage resulting in a marginal zone of death.35,36
A critical concept in graft harvest is perpendicularity.16 Although this may be achieved through an open or an arthroscopic approach, it is critical that grafts that are orthogonal to the articular surface are harvested.39 This allows for improved articular congruity, in addition to more precise restoration of the condylar bevel and radius of curvature, which is of particular importance in defects requiring multiple plugs.
Donor site morbidity remains a technical concern when OAT is considered. Early reports used iliac crest cortical bone grafts to fill lesions following graft harvest. Similar fill using the bone remaining from recipient site preparation with a trephine cutting technique has been documented.64 In a canine model, Feczkó et al in 2003 compared several biodegradable materials for donor site filling, including hydroxyapatite, carbon fiber, polyglyconate-B, compressed collagen, and polycaprolactone.20 They found on second-look arthroscopy and histologic evaluation that compressed collagen yielded the best fibrocartilage coverage of harvest site defects. Several composite bone graft substitute polymer plugs are now commercially available and have been used to fill donor site defects (TruFit, Smith & Nephew, Andover, Mass; OsseoFit, Kensey Nash Corp., Exton, Pa).80
Pearls and Pitfalls
Many of the complications associated with OAT can be directly attributable to technical details. Hemarthrosis has been reported, and filling of the donor site, as well as placement of a postoperative drain, may reduce this problem.29 Donor site collapse has been reported and may be avoided by maintaining a 1- to 2-mm spread between harvest sites and from the edge of the condyle, as well as by taking into account the natural convergence of the bases of individual grafts caused by the curvature of the condylar surface. Graft failure may occur for a number of reasons. Impaction forces may be minimized by careful matching of recipient site and donor plug depth. Although this has already been incorporated into several commercial systems, optimization of the difference between plug diameter and recipient bed diameter to allow good press-fit without excessive force is important. Careful removal of donor plugs limits collateral damage to surrounding cartilage. Likewise, meticulous handling of the donor plug from the osseous side minimizes microtrauma to the graft cartilage surface, which can affect chondrocyte viability; OCD lesions and other bony defects extending into subchondral bone may require longer grafts. In cases where multiple grafts are used, minimizing the step-off between grafts and relative to the surrounding cartilage will decrease surface fibrillation and edge-loading of grafts.
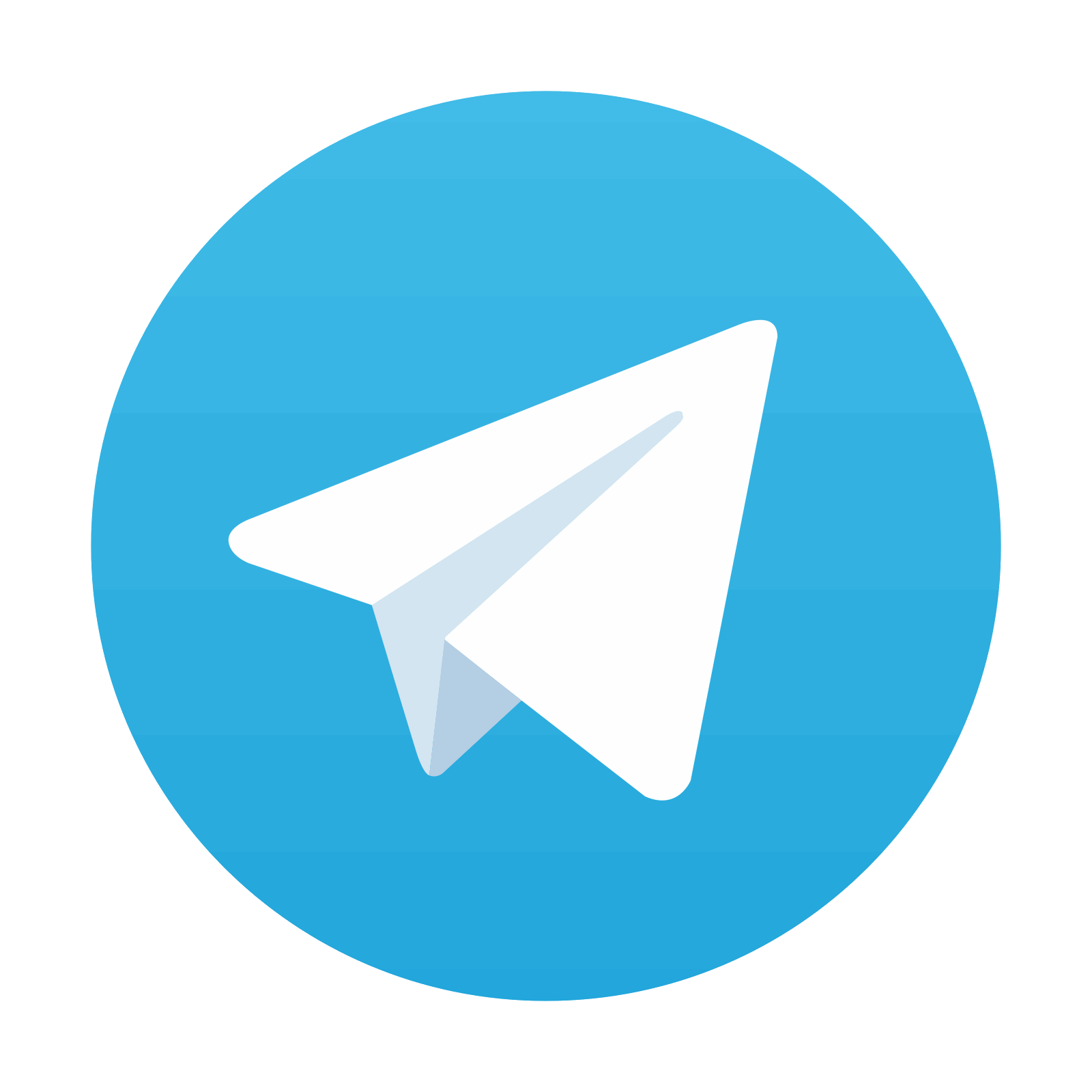
Stay updated, free articles. Join our Telegram channel

Full access? Get Clinical Tree
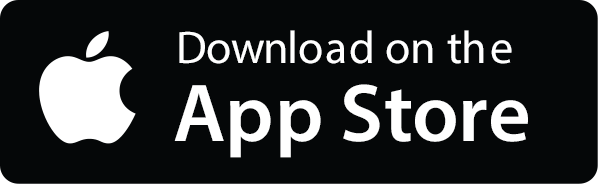
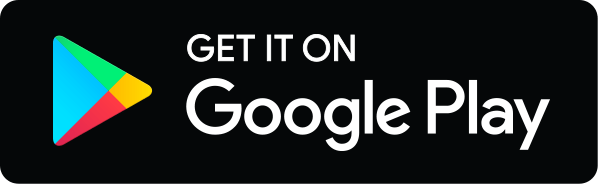