Orthoses for Spinal Dysfunction
Jeff Coppage and S. Elizabeth Ames
Learning Objectives
On completion of this chapter, the reader will be able to:
1. Identify the nomenclature for spinal orthoses.
2. Describe the basic structural and functional anatomy of the spine and its alignment.
3. Discuss the three-column concept of spine stability as it pertains to spine trauma.
4. Identify the types of injury and pathology for which spinal orthoses are used.
5. List the different types and functional use of spinal orthoses.
7. Evaluate different areas of the spine as being amenable to bracing.
8. Discuss indications and contraindications for using spinal orthoses.
9. Describe basic pathophysiology of scoliosis and its implications.
10. Identify common braces used in the treatment of scoliosis.
11. Discuss the prescription process for a spinal orthoses.
12. Value the importance of a multidisciplinary team approach in treating disorders of the spine.
External orthoses are used to manage a variety of spinal conditions. The general purpose of a brace is to limit the motion of a spinal region, decreasing the amount of load applied to the region treated. Orthoses are most frequently used when there is concern that loading the spine may result in deformity (i.e., treating an unstable fracture), when the spine is compromised in a way that requires additional support for healing (i.e., postsurgical management or osteoporosis), when the patient is experiencing low back pain that can be relieved by either limiting motion or increasing abdominal support, or when there is existing spinal deformity such as scoliosis. Another major function of a spinal orthosis is to serve as a psychological reminder to restrict trunk or neck motion, or at least to encourage the patient to move cautiously.1
For spinal conditions, an orthosis is defined as an external device applied to the body to restrict motion in a particular body segment or spinal region. The American Academy of Orthopaedic Surgeons standardized the nomenclature used for describing orthoses in spinal management in 1973 and divided them broadly into five categories (Table 13-1)2:
Table 13-1
Nomenclature for Spinal Orthoses
Acronym | Name |
RIGID THERMOPLASTIC OR METAL ORTHOSES, OR BOTH | |
SIO | Sacroiliac orthosis |
LSO | Lumbosacral orthosis |
TLSO | Thoracolumbosacral orthosis |
CTLSO | Cervicothoracolumbosacral orthosis |
CTO | Cervicothoracic orthosis |
CO | Cervical orthosis |
SOFT GARMENTS AND SUPPORTS | |
SI belt | Sacroiliac belt |
LS corset | Lumbosacral corset |
DL corset | Dorsolumbar corset |
Soft collar | Nonreinforced cervical collars made from foam or any low modulus material |
Orthoses may also be classified by their rigidity (i.e., rigid, semirigid, or flexible) or by a combination of their materials and whether they are prefabricated or custom fit. Historically, orthoses have been named according to their inventor or city of invention. This chapter provides an overview of spinal anatomy and biomechanics as they apply to orthotic use, highlights tips to ensure optimal fit and avoid complications, and discusses each of the three major spinal regions that are amenable to orthotic management and the orthoses used in each region as well as the various types of pathology for which brace treatment is used. This chapter describes common spinal orthoses, grouped by region (cervical, thoracic, and lumbosacral) and describes the clinical conditions that are most often assisted by the use of spinal orthoses.
Managing a patient who may benefit from a spinal orthosis requires an interdisciplinary team approach. Direct communication between the patient, physician, orthotist, nurse, physical therapist, and other rehabilitation personnel is necessary to ensure that the health care professionals, the patient, and caregivers all understand the rationale, limitations, and expected outcomes when prescribing and using an orthosis. In the use of spinal orthoses there are specific instructions that accompany orthotic management to avoid complications and promote optimal care. Rehabilitation professionals are responsible for designing therapeutic interventions that optimize function while carefully observing precautions and treatment goals of patient management for persons with spinal orthoses. Understanding the design of and rationale for the orthosis being used is critical to the overall success of the program.
Anatomy and biomechanics
The spine consists of seven cervical vertebrae, 12 thoracic vertebrae, five lumbar vertebrae, five sacral vertebrae, and three to four coccygeal segments. Load sharing intervertebral discs are interposed between adjacent vertebrae in the cervical, thoracic, and lumbar segments; in the sacral and coccygeal regions, the segments are fused. Two adjacent vertebrae and the intervertebral disc define the functional spinal unit (FSU). Multiple FSUs are combined in a superstructure capable of lateral bending, flexing, extending, and axial rotation. Different levels of the spine vary in their contribution to overall spine range of motion. In the cervical spine, the majority of motion in the sagittal plane (flexion-extension) occurs through Occ-C2, C4-5, and C5-6 (Figure 13-1).3 The majority of axial rotation occurs at the level of C1-C2 and is made possible by the unique anatomy of the atlantoaxial articulations. Lateral bending of the cervical spine is more evenly distributed with the upper subaxial cervical spine contributing only slightly more than other regions. Sagittal motion in the thoracic spine increases in a cranial to caudal direction. The upper segments provide approximately 4 degrees at each level and the lower segments provide approximately 6 degrees and increase to approximately 12 degrees per level at the thoracolumbar junction.3 Axial rotation is greatest in the upper thoracic spine and gradually decreases caudally. Segmental contribution to lateral bending is fairly well distributed over the length of the thoracic spine. The lumbar spine contributes more flexion-extension (Figure 13-2) but significantly less axial rotation. These regional differences in motion are related to anatomical differences, primarily articular process orientation, between the thoracic and lumbar vertebrae. The ligamentous structures of the spinal column play an important role in spine kinematics by augmenting overall spinal stability while maintaining flexibility.
The normal spine is essentially vertical in the coronal plane but exhibits four curves in the sagittal plane. The terms kyphosis and lordosis are used to describe sagittal curves. Kyphosis refers to a curve in the sagittal plane with a posterior convexity (forward bend). The thoracic and sacral portions of the spine demonstrate kyphosis. The normal amount of thoracic kyphosis ranges from 20 to 50 degrees.4 The term lordosis describes a curve in the sagittal plane with an anterior convexity (posterior bend). The cervical and lumbar portions of the spine demonstrate lordosis. The mean lordosis in the cervical spine is 35 to 40 degrees.5,6 The normal range of lordosis in the lumbar spine is from 20 to 60 degrees.4 Although multiple curves are present, an overall sagittal balance is maintained (Figure 13-3). The sagittal balance of the spine can be assessed using a plumb-line, or gravity-line, technique. In a normal balanced spine, a plumb-line from the center of the C7 should fall ±2 cm from the sacral promontory in the sagittal plane (see Figure 13-3).7 These curves, as well as appropriate coronal and sagittal balance, allow for increased flexibility and shock-absorbing capacity while maintaining necessary stiffness and stability.3
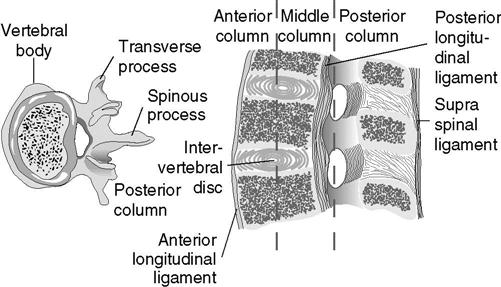
The major load on the spine under normal physiological conditions is axial. Axial loading causes compression of the vertebral column and its individual FSUs. The natural kyphotic and lordotic curves of the spine increase in magnitude and components of individual FSUs (vertebrae and intervertebral discs) deform slightly in response to compression.3 Soft tissue (ligaments and musculature) as well as bony architecture serve limit the degree to which the gross architecture of the spine can be deformed and the properties intrinsic to the vertebrae and intervertebral discs counter the effects of compressive forces. The spine must resist tension and shear forces in addition to axial loads. The properties of the spine conform to Wolff’s law, which states that form follows function. Studies of the spine reveal that the spine as a whole can resist higher compressive loads than tension or shear.3 Furthermore, segments of the spine that experience greater compressive loads in have been found to be capable of withstanding higher loads before failure. Biomechanical studies demonstrate that the ability of the spine to withstand forces increase in a cephalocaudal direction such that the lumbar spine, which must withstand the sum weight of the body above it, has the greatest compressive strength.8,9 The vertebral body (anterior column and anterior aspect of the middle column) provide the majority of this resistance to compression, which translates into increased overall stability in fractures in which the vertebral body is intact and a greater likelihood that bracing may not be necessary. This structure-function relationship is vital to understanding the rationale behind bracing and other treatments of back pain, spinal deformity, or spine injury.
The three-column concept
An understanding of spine injury and stability is vital to understanding the role that bracing and various orthoses play. The concept of the three-column spine was initially described by Denis10 in 1984 and is widely used as a means to help define spinal stability. Denis used the three-column model as a basis for his classification of traumatic spine injuries. The three columns are the anterior, middle, and posterior columns (Figure 13-3). The anterior column consists of the anterior aspect of the vertebral body, anterior annulus fibrosis, and anterior longitudinal ligament. The middle column includes the posterior longitudinal ligament, posterior annulus fibrosis, and posterior aspect of the vertebral body. The posterior column consists of the posterior vertebral arch as well as the supraspinous and interspinous ligaments, facet joints, and ligamentum flavum. Debate exists as to whether injury to the posterior column or the middle column is the main factor that destabilizes thoracolumbar spine fractures3,11–15; a combination of both, however, results in a highly unstable spine. Compression fractures and burst fractures are the most common clinical entities where orthoses are considered. Denis defined these types of fractures using the three-column concept (Box 13-1).
Compression fractures are defined as failure and wedging of the anterior column and occur with axial forces combined with spine flexion about an axis located in the middle column. The middle column remains intact, which provides stability and prevents retropulsion of bony fragments into the spinal canal. Some degree of partial failure of the posterior ligamentous structures may be present due to the tension forces present during the initial forced flexion. Depending on the degree of anterior column failure, some compression fractures may undergo progressive collapse and lead to increasing posttraumatic kyphosis.16 Compression fractures with greater than 50% of vertebral body height loss are more likely to have instability due to associated posterior ligamentous involvement, which may result in progressive injury and kyphotic deformity due to the compressive forces conveyed by an upright posture.3,16
Burst fractures generally involve failure of the anterior and middle columns due to axial loading with or without additional moments, depending on the fracture pattern.17 Although these fractures are defined as two-column involvement, Holdsworth13 described an associated greenstick fracture of the lamina (posterior column). These injuries are referred to as three-column burst fractures. Involvement of the middle column is significant because it may lead to retropulsion of bony fragments in the spinal canal and lead to spinal cord injury. Controversy exists regarding which burst fractures are “stable” and are amenable to bracing, and which require surgery. A recent randomized trial of hyperextension casting followed by brace management compared with operative management demonstrated that patients treated operatively experienced improved functionality more rapidly than those immobilized, but long-term outcomes in terms of deformity, chronic pain, and neurological status were equivalent.18
Fit and function of the spinal orthosis
All spinal orthoses have several common effects on the spinal region they treat. Their primary action is to reduce gross spinal motion, and the degree to which they accomplish this depends on both their materials and their design. Secondary effects include the stabilization of individual FSUs, reducing the range of motion of one vertebra relative to another. Spinal orthoses also apply closed chain forces designed to counter a deforming force, such as providing hyperextension to a fracture that is vulnerable in flexion. Finally, they reduce loads on the spine itself by preventing specific actions, such as preventing bending and twisting to reduce stress on surgical implants. Each region of the spine has specific needs based on its predominant motions, loads, anatomic features, and physical condition.
The shape of a spinal orthotic, much like the natural shape of the spine, has an intimate relationship with the desired function. Braces often help restore or exaggerate natural spine structure. When thoracolumbar body casts were more commonplace in clinical practice, the casts were applied with the patient positioned supine on a casting table with a belt under their lumbar spine such that they were casted in a position of hyperextension.19 This hyperextension (hyperlordosis) has been used in many types of braces (i.e., Jewett and CASH) because it removes or decreases the amount of flexion, which reduces compressive force on the fractured vertebral body and limits distraction of the posterior elements. Hyperextension braces achieve the intended positioning by using a three-point mold. A three-point mold refers to three points of applied force: one posteriorly and two anteriorly, with one located cephalad to the level of the posterior force and one caudal.3 An analogy to this concept is if one was trying to break a pencil using two hands with his or her thumbs in the center and fingers located at either end. The bending that results is the intended effect of the three-point mold. The desired result is prevention of the progressive kyphotic deformity that is associated with significant compression and burst fractures. Another example of three-point molding is seen in corrective braces used for scoliosis, except that the forces are directed in a coronal plane instead of a sagittal plane. It is imperative to ensure that the contour and fit of the brace promotes adequate alignment of the spine and avoids potential complications associated with forces applied to the body for long periods of time.
An orthosis must have intimate contact with bony prominences to be effective.20 The biomechanical principles of bracing the spine itself can be understood in these terms; in reality, however, using an orthosis to treat a human condition is subject to other variables. Compliance and the psychological effects of bracing can be significant issues including developing a psychological dependence on the orthosis.21–26 The financial burden can be significant, with costs ranging from $32 to greater than $5000.1 Physical issues also apply, particularly in an increasingly obese population. The ability of a brace to apply appropriate forces, either stabilizing or corrective, depends on its ability to act on the structures of the spine. This in turn depends on the soft tissue envelope in the contact areas around the spine. Too little or excessive pressures transmitted through soft tissues can result in significant physical complications including skin breakdown, loss of reduction or spinal alignment, pain due to the brace, weakening of the immobilized muscles, and soft tissue contractures.26–32 The prescription and use of a spinal orthotic should always be prescribed with an appropriate monitoring system and rehabilitation program. Orthoses must be modified periodically, and a comfortable and appropriate fit is critical to preventing skin problems and ensuring compliance.
Compliance with brace wear is a significant clinical issue, particularly because the orthosis is specifically designed to restrict motion, which is neither an accommodating nor pleasant approach to therapy. Studies in adolescent patients with scoliosis wearing “smart braces” that can track wear time indicate compliance rates of 9% to 30%; similar studies have not been done for orthoses used for other conditions. In the current health care environment most patients with significant spinal conditions recover at home rather than in rehabilitation facilities. Orthosis designs that increase the difficulty of donning or doffing decrease the patient’s ability to use the brace effectively if they are not supervised and assisted daily. The only orthoses that ensure compliance are fiberglass casts, which are very rarely used in current times.
Regional Orthoses
Cervical
The cervical spine is the most intuitively easy structure to consider bracing, in that the bony occiput, mandible, sternum, and clavicles provide appropriate supporting structures. Unfortunately this advantage is offset by the limited surface area available for contact and the fact that the mandibles and clavicles are often in motion during normal activities. The complexity of the cervical soft tissues—vessels, airway, esophagus—is a disadvantage, in that significant forces cannot be applied directly to the spine without significant visceral effects. Orthoses for the cervical spine can be limited to the subaxial spine itself—a cylinder that fits around the neck with a trimline at the occiput, mandible, and sternoclavicular structures—or may extend proximally and distally (halo vest) or distally onto the thoracic cage for additional control (cervicothoracic orthosis [CTO]).
Cylindrical cervical braces limit a variable degree of motion depending on the design and primarily provide proprioceptive feedback as a reminder to limit the range of motion of the neck. Available choices are many and this list is not exhaustive; commonly used choices include the soft collar, the Stifneck (Laerdal, Armonk, NY), the Philadelphia (Philadelphia Cervical Collar, Thorofare, NJ), and the Miami J (Össer, Foothill Ranch, CA) (Figure 13-4).
Soft collars tend to be the most comfortable of the available cervical collars but provide little stability to the cervical spine. The collar is a soft foam rubber covered with stockinet or other gentle fabric with a Velcro-type fastener. These collars have been shown to provide up to 10% restriction to cervical motion in all planes.33–35 Conversely, Miller et al36 analyzed differences in functional cervical spine motion between no collar, a soft collar, and a rigid collar while performing 15 different activities of daily living* (ADLs). The authors found that there was no significant difference in limitation of sagittal range of motion between the soft and rigid collars during 13 of the 15 ADLs tested (significant differences were noted while reversing a car and sitting down in a chair). No significant differences were noted during lateral bending, and the difference in rotational movement restriction was only significant while reversing a car. The soft cervical orthosis is used primarily as a comfortable reminder to the patient to limit exaggerated neck movements and may be useful in cases of minor whiplash, cervical spondylosis, or as a postoperative adjunct with a stable spine.3 Soft collars are inappropriate for an unstable cervical spine.
A great deal more support is required in patients with injuries that compromise spinal stability. This prompted the development of the reinforced cervical collar, which is a commercially produced, prefabricated orthosis that combines some of the soft materials found in soft collars with a semirigid, contoured plastic external frame. Many different types of reinforced collars are available, such as the Philadelphia, Aspen (Aspen Medical Products, Irvine, CA), Miami J, NecLoc (Össur, Foothill Ranch, CA), and Stifneck. Most reinforced collars have anterior and posterior shells with inner padding and trim that close around the neck and fasten with Velcro-type fasteners. The collars are contoured such that they abut the sternum, clavicles, trapezius, and upper thoracic spine inferiorly and the mandible and occiput superiorly, which provides some degree of end-point control. Most have openings anteriorly to accommodate respiratory and ventilator equipment.
Although there are many similarities between the different cervical collars, studies have found significant differences in the degree to which different collars restrict cervical spine motion. The ability for a cervical collar to provide cervical stability is very important in that approximately 3% to 25% of spinal cord injuries occur after the initial spine injury.37 Multiple studies have evaluated the various reinforced collars for their ability to promote cervical stability as well as avoid complications. Askins and Eismont37 studied five common reinforced cervical collars by using anteroposterior and lateral radiographs to assess cervical spine motion in normal, healthy volunteers. They found the NecLoc to be statistically superior with respect to limitation of cervical motion in all planes, followed by the Miami J collar, when compared the Philadelphia and Aspen collars. Kaufman and colleagues38 found the NecLoc to provide superior restriction of motion versus the Philadelphia collar and a soft collar. Ducker39 found the NecLoc and Miami J collars to be statistically superior to the Stifneck, Philadelphia, and soft collars.
The treating physician must consider fit and skin protection when selecting a reinforced cervical collar in addition to selecting a collar that is capable of providing adequate stability. Fisher29 studied the importance of proper fit of a cervical orthosis and found that an inappropriately fitted collar was equivalent to not wearing any collar at all. Bell and colleagues32 analyzed the effects that ill-fitted Miami J collars have on the degree of cervical motion restriction. They found that in flexion-extension, the braces that were too large and too small allowed increased motion but only extension in the too large brace produced a statistically significant increase in allowed motion. Both the too small and too large braces allowed significantly more motion in left and right axial rotation. The too large and too small braces allowed significantly more lateral bending motion to the right but only the too small brace allowed a significant increase in left lateral bending. Plaisier and colleagues28 evaluated common cervical collars and the pressures they exerted on craniofacial tissues with respect to capillary closing pressure. The pressure at the collar-skin interface was measured using an electropneumatic sensor placed between the collar and skin. This measurement was compared with a value of 32 mm Hg, which represents capillary closing pressure as defined by Berne and colleagues.40 They found significant differences between collars in the amount of pressure exerted on the tissues. The Stifneck collar produced pressures in excess of capillary closing pressure at most collar-tissue interfaces, whereas the Miami J collar exerted pressures well below the capillary closing pressure.
Braces that need to immobilize the occipitocervical spine require fixation to the skull and significant rotational control. The most commonly used example is the halo vest (Figure 13-5). Facial fracture fixation with traction applied through a skull ring was developed by Frank Bloom, MD, during World War II to treat pilots with facial fractures and severe burns. The device was then adapted by Vernon Nickel, MD, and first reported in 1959.8 Primary features include a ring anchored to the skull with skeletal pins and connected to a body jacket by four vertical uprights. Changes in material design and advances in plastic technology have allowed significant modifications to the halo vest—molded thermoplastic jackets, radiolucent rings and uprights, shaped rings open posteriorly for patient comfort while supine, and multidirectional adjustments in the connecting mechanisms—but the principles of fixation have remained the same. Six to eight transcranial pins provide secure proximal fixation (end point control), and full contact support around the thorax and torso provide the distal fixation.
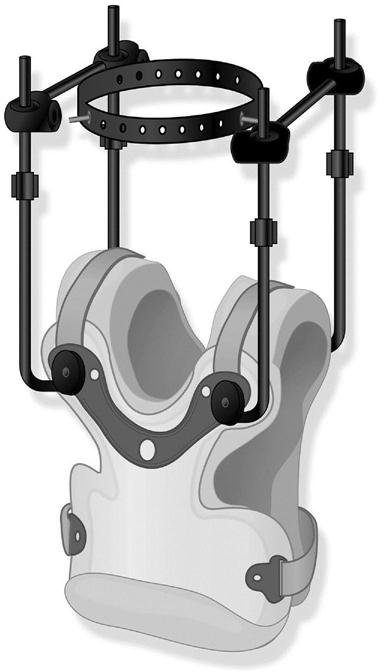
The ring of the halo is positioned 1 cm above the eyebrows and the tip of the ears, with care taken to keep the ring well clear of the skin surface. Four pins are inserted into the outer table of the skull with 6 to 8 lb/in of torque.41 The anterior pins are placed at the equator (widest point) of the skull above the lateral one third of the eyebrow to avoid the frontal sinus, supraorbital and supratrochlear nerves, and temporalis muscle. Posteriorly, pins are placed 1 to 2 cm posterior to the ear diagonally opposite the anterior pins. This provides secure fixation to allow for manipulation in both flexion/extension and in translation.
The halo vest itself was originally a plaster cast fit over the torso with a trimline at or slightly above the inferior costal margin of the last rib.42 Advances in materials allow for bivalved thermoplastic shells that can be released with the patient supine to allow for hygiene, and some designs are fleece lined. A four-pad halo vest that completely avoids the shoulder girdle has been proposed to avoid scapular movements transferring loads to the neck.43,44 Two anterior and two posterior rods link the vest to the ring through a series of connectors. Modern connectors allow translation in multiple planes with the ability to lock them into position once reduction has been achieved.
Complications can occur with halo vest use, including pressure sores, loss of reduction (particularly in injuries involving the posterior elements), and pin infection and loosening.45–49 A higher rate of complications occurs in older patients. In a review of 53 patients with a mean age of 79.9 years, Horn and colleagues50 recorded 31 complications in 22 patients. Serious complications included respiratory distress and dysphagia, and the cause of death in 6 of 8 patients who died within the treatment period was thought to have some relationship to the halo treatment. Modern day halo vests should always have a wrench attached to the front of the halo vest for quick removal in case of a cardiac emergency.
Studies comparing the halo to other types of cervical bracing with respect to the ability to limit motion have had variable findings. Johnson et al51 found that the halo allowed only 4% of normal sagittal motion, 1% of normal rotation, and 4% of lateral bending in normal subjects. A cadaveric study of simulated odontoid fractures demonstrated the halo was superior in all planes versus a Miami J collar, a Minerva brace, and a soft collar.34 In studies comparing subjects in various positions and activities demonstrated a maximum reduction of sagittal motion of only 30%.52 A study of injured patients by Benzel et al53 demonstrated paradoxically increased movement at the injured levels in the halo versus a Minerva brace, which they attributed to the pull of the neck muscles with attempted flexion or extension against the rigidly fixed head. Ivancic and Telles54 studied neck motion in a normal versus loosely applied halo vest in the supine and prone positions using a cadaveric cervical spine between an anthropometric dummy and surrogate head. Results showed significantly increased motion in the loose vest with respect to the normal vest. The authors conclude that such increased motion may play a role in delayed unions and nonunions of cervical spine fractures.
The halo vest is useful in providing reduction and provisional stabilization of injuries or conditions causing instability at the occipitocervical junction, and can be used to provide additional support after these patients have surgery. It is best used for reducing angular and translational deformities in cervical spinal fractures at the proximal and distal regions of the spine; in the midportion, studies have shown that a halo actually increases the forces on the injured vertebrae.53 It is commonly used for complex combined C1 and C2 fracture patterns where internal stabilization is not possible without extension to the occiput, which results in severe loss of motion for the patient.55,56 It is used to provide additional stability after complex surgical reconstructions or noninstrumented fusion or wiring constructs after surgery at C1-2, and may be used to supplement instrumented fusions in situations with poor bone quality or significant instability, such as in osteoporosis or rheumatoid arthritis patients.57 The halo is no longer used as frequently in subaxial (i.e., C3-C7) trauma due to the advances in spinal instrumentation. It is still considered the treatment of choice for some types of fractures of the axis (hangman’s fractures) and in some flexion-compression injuries.58,59 A fracture of the odontoid process at C2 is a common injury among older patients and historically has often been treated with a halo vest.60–62 Currently the optimal treatment for these patients is a topic of debate, with some authors reporting that simple collar immobilization provides equivalent results.63–69 Significantly higher mortality has been reported in older patients treated with a halo compared with those treated with a cervical collar.61,70 Daentzer and colleagues71 performed a retrospective study of 29 patients divided into two groups based on age less than or greater than 65 and found that while clinical and radiographic results were equivalent between the two groups, the interval to healing and rate of complications were higher in the older than 65 age group.
Rehabilitation of the patient with a halo is challenging. The fixed head position results in a different ability to use visual cues, and the weight of the vest and position of the head combine to change the patient’s center of mass. Ambulatory patients in halo vests may exhibit a forward-flexion posture to accommodate this; a cane or walker may be required while the halo is in place. Likewise, there is a readjustment period after the halo is removed that may require postural reeducation as part of the rehabilitation strategy.
Cervicothoracic and Thoracic
CTO can be divided into two categories: those that use the thoracic spine to support treatment of a subaxial cervical spine or upper thoracic spine problem, and those that support treatment at the upper cervical spine. Examples in the first category include thoracic extensions added to a cylindrical cervical orthosis (i.e., Extended Miami J), or an orthosis that utilizes pads on the chin and occiput to connect to the trunk by four stiff uprights or circumferential supports (i.e., Minerva). The Minerva brace (Figure 13-6) is the most effective method for immobilizing C1-2, and has been shown to limit flexion-extension by approximately 79%, axial rotation by 88%, and lateral bending by 51%.72 The cervicothoracic area is a particularly challenging area to immobilize in that it is a transitional area between the very mobile and lordotic cervical spine and the kyphotic thoracic spine. Little data exist in the literature regarding immobilization of the upper thoracic spine; for those conditions not requiring surgery, an extended cervical orthosis can be used.1 The Minerva brace, SOMI brace (Figure 13-7), or a custom-molded CTO can be used for conditions extending as far caudally as T5. In general, increasing the length of the orthosis down the trunk enhances its capabilities.51
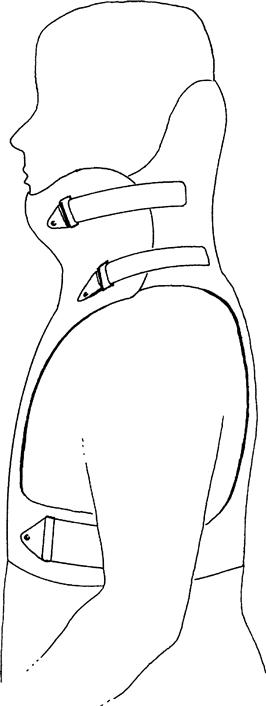
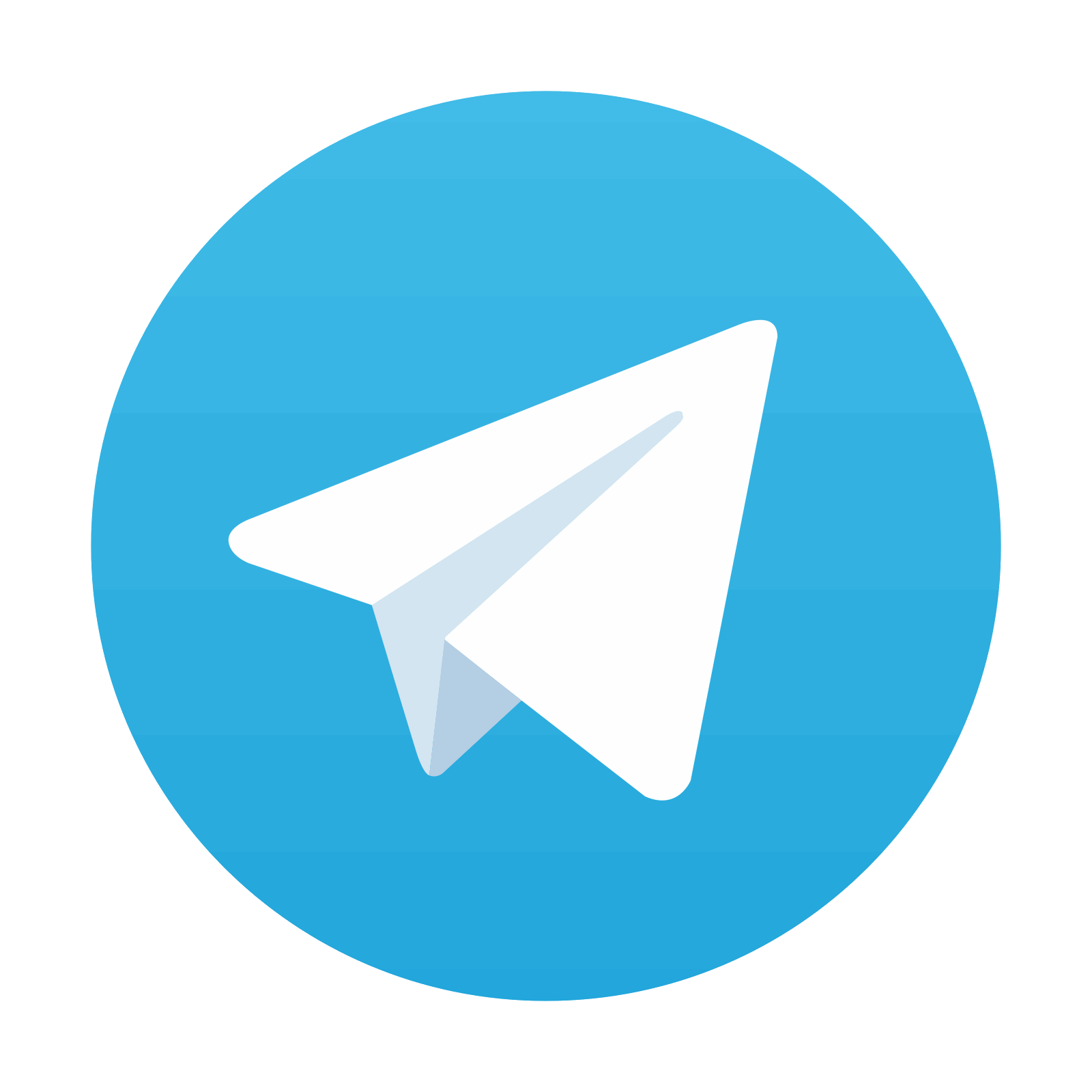
Stay updated, free articles. Join our Telegram channel

Full access? Get Clinical Tree
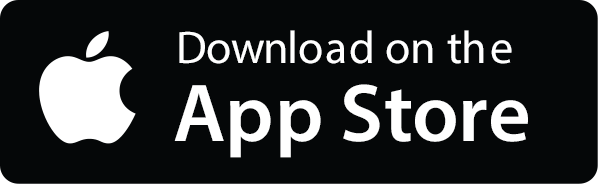
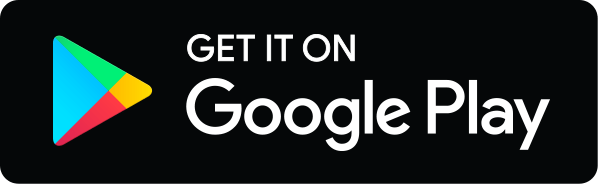