Orthoses for Knee Dysfunction
Anthony E. “Toby” Kinney and Ellen Wetherbee
Learning objectives
On completion of this chapter, the reader will be able to:
2. Appreciate the anatomical structures of the knee that provide intraarticular stability.
4. Describe the various classification systems used to describe knee orthoses.
6. Identify the force vectors used to control knee motion used in various designs of knee orthoses.
9. Provide clinical rationale for utilizing bracing as an intervention for impairments at the knee.
Knee braces have been used as a common intervention in the protection and stabilization of the knee joint to decrease a person’s pain and improve their functional and recreational activities (Figure 11-1). It has become commonplace for anyone to walk into a pharmacy or drug store and purchase a knee brace such as a neoprene sleeve (Figure 11-2). However, their effectiveness in providing ligamentous stability and optimal patellofemoral tracking has been debated.1,2
In 1984, the American Academy of Orthopaedic Surgeons developed a classification system that grouped knee orthotics by their intended function: prophylactic, rehabilitative, and functional knee braces.3 Currently, patellofemoral braces and unloader knee braces are additional classification categories.4,5 It could be debated that unloader braces are merely functional osteoarthritis (OA) braces. Prophylactic knee orthoses are designed to reduce the risk of knee injury for those individuals who are engaged in “high-risk” activities, especially those individuals who have a history of previous knee dysfunction. Rehabilitative knee orthoses are used to protect a knee that has been injured or surgically repaired until adequate tissue healing has occurred. Functional knee orthoses (FKOs) attempt to provide biomechanical stability when ligaments are unable to do so during daily activities. Patellofemoral knee braces are intended to optimize tracking of the patella and decrease anterior knee pain. Unloader knee braces are meant to decrease compressive forces across the tibiofemoral joint and decrease arthritic knee pain. This functional classification system continues to be helpful for health care providers who work with patients who have knee dysfunction.
It is imperative during the clinical decision making process that clinicians use a patient-centered approach while at the same time incorporating the best available scientific research and their own clinical expertise. Patient goals, function, preferences, and impairments should be factors in deciding the type of knee orthosis to use. For instance, a patient who has anterior cruciate ligament (ACL) deficiency will use a different brace than a patient with medial compartment knee OA. In all circumstances, it is important to understand the anatomy and biomechanics of the knee joint and subsequent knee dysfunction when providing a knee orthosis to a patient.
In order for a clinician to select the appropriate knee orthosis for the patient, the clinician needs a mastery of normal knee structure and function. This chapter reviews the anatomy and biomechanical stability of the knee and patellofemoral joints and the physiological and accessory motions of the tibiofemoral and patellofemoral joints. The implications of knee pathology on knee biomechanics are discussed, and the design and functional goals of prefabricated and custom-made knee orthoses are examined. Indications for knee orthoses in the management of common knee injuries and dysfunction are also discussed. Finally, the chapter concludes with discussion about strategies for clinical decision making in choosing an appropriate functional orthosis for active patients and athletes with ligamentous instability of the knee.
Anatomy of the knee
The articulations at the tibiofemoral joint and the patellofemoral joint form the knee complex. An understanding of the anatomy and biomechanics of each respective joint is critical in knowing the potential stresses and implications of knee pathology that can occur at the knee complex.
The Tibiofemoral Joint
The knee joint is a hinge-like articulation between the medial and lateral condyles of the femur and the medial and lateral tibial plateau (Figure 11-3). Because of the shape and asymmetry of the condyles, the instantaneous axis of knee flexion/extension motion changes through the arc of motion. As the knee moves from extension to flexion, the instant center moves posteriorly.6 In open chain movements (non–weight-bearing activities), the tibia rotates around the femoral condyles. In closed chain movements (weight-bearing activities), an anatomical locking mechanism is present in the final degrees of extension as the longer medial femoral condyle rotates medially on the articular surfaces of the tibia. Consequently, if the instant center of pathway changes, it will alter the optimal joint mechanics and therefore result in abnormal knee stressors. The alignment between an adducted femur and relatively upright tibia creates a vulnerability to valgus stress in many weight-bearing activities. The capsule that encases the knee joint is reinforced by the collagen-rich medial and lateral retinaculum. The medial and lateral menisci rest on the tibial plateau. They are fibrocartilaginous, nearly ring-shaped disks that are flexibly attached around the edges of the tibial plateau (see Figure 11-3). These menisci increase the concavity of the tibial articular surface, enhancing congruency of articulation with the femoral condyles to facilitate normal gliding and distribute weight-bearing forces within the knee during gait and other loading activities.7 The menisci also play an important role in nutrition and lubrication of the articular surfaces of the knee joint.
Stability to the tibiofemoral joint is provided by sets of ligaments. The medial (tibial) collateral ligament and the lateral (fibular) collateral ligament are extrinsic ligaments. The collateral ligaments counter valgus and varus forces that act on the knee. In addition, two intrinsic ligaments of the tibiofemoral joint, the anterior cruciate and posterior cruciate ligaments check translatory forces that displace the tibia on the femur. The location of attachments makes each of these ligaments most effective at particular places in the knee’s normal arc of motion.7 Additionally, contraction of the quadriceps and knee flexor muscle groups produce compressive forces that help stabilize the knee. Muscles of the hip and lower leg also make contributions to the mechanics of the femur and tibia, respectively, which impact the movements of the knee complex.
Medial Collateral Ligament
The medial (tibial) collateral ligament (MCL) is a strong, flat membranous band that overlays the middle portion of the medial joint capsule (Figure 11-4). It is most effective in counteracting valgus stressors when the knee is slightly flexed to fully extended. Approximately 8 to 10 cm in length, it originates at the medial epicondyle of the femur and attaches to the medial surface of the tibial plateau. The MCL can be subdivided into a set of oblique posterior fibers and anterior parallel fibers.
A bundle of meniscotibial fibers, also known as the posterior oblique ligament, runs deep to the MCL, from the femur to the midperipheral margin of the medial meniscus and toward the tibia. These fibers connect the medial meniscus to the tibia and help form the semimembranosus corner of the medial knee. Additionally, the medial patellar retinacular fibers play a reinforcing role.8
Lateral Collateral Ligament and Iliotibial Band
The lateral (fibular) collateral ligament (LCL) resists varus stressors and lateral rotation of the tibia and is most effective when the knee is slightly flexed. The LCL runs from the lateral femoral condyle (the back part of the outer tuberosity of the femur) to the proximal lateral aspect of the fibular head (see Figure 11-4). The tendon of the popliteus muscle and the external articular vessels and nerves pass beneath this ligament.
Another lateral structure that acts on the knee complex is the iliotibial band (ITB). The ITB is positioned slightly anterior to the LCL and is taut in all ranges of knee motion. Although the position of the ITB allows it to stabilize against varus forces, as does the LCL, the ITB also appears to assist the ACL, preventing posterior displacement of the tibia when the knee is extended.9
Anterior Cruciate Ligament
The ACL runs at an oblique angle between the articular surfaces of the knee joint and prevents forward shift and excessive medial rotation of the tibia as the knee moves toward extension (Figure 11-5). The ACL attaches to the tibia in a fossa just anterior and lateral to the anterior tibial spine and to the femur in a fossa on the posteromedial surface of the lateral femoral condyle. The ACL’s tibial attachment is somewhat wider and stronger than its femoral attachment. Some authors divide the fasciculi that make up the broad, somewhat flat ACL into two or three distinct bundles. The ligament’s anteromedial band, with fibers running from the anteromedial tibia to the proximal femoral attachment, is most taut in flexion and relatively lax in extension. The posterolateral bulk (PLB), which begins at the posterolateral tibial attachment, is most taut in extension and relatively lax in flexion. An intermediate bundle of transitional fibers between the anteromedial band and PLB tends to tighten when the knee moves through the midranges of motion. This arrangement of fibers ensures tension in the ACL throughout the entire range of knee motion. The ACL is most vulnerable to injury when the femur rotates internally on the tibia when the knee is flexed and the foot is fixed on the ground during weight-bearing activities.10
Posterior Cruciate Ligament
The posterior cruciate ligament (PCL) restrains posterior displacement of the tibia in its articulation with the femur, especially as the knee moves toward full extension.7 The PCL is shorter and less oblique in orientation than the ACL; it is the strongest and most resistant ligament of the knee. PCL fibers run from a slight depression between articular surfaces on the posterior tibia to the posterolateral surface of the medial femoral condyle (see Figures 11-3 and 11-5). Like the ACL, the PCL can be divided into anterior and posterior segments. The larger anterior medial band is most taut between 80 and 90 degrees of flexion and is relatively lax in extension. The smaller PLB travels somewhat obliquely across the joint, becoming taut as the knee moves into extension. The PCL plays a role in the locking mechanism of the knee, as tension in the ligament produces lateral (external) rotation of the tibia on the femur in the final degrees of knee extension. The PCL may also assist the collateral ligaments when varus or valgus stressors are applied to the knee.7
Coursing along with fibers from the MCL is the meniscofemoral ligament, which stretches between the posterior horn of the lateral meniscus and the lateral surface of the medial femoral condyle. The meniscofemoral ligament has sometimes been described as a third cruciate ligament.11 The anterior meniscofemoral band (ligament of Humphry) runs along the medial anterior surface of the PCL and may be up to one-third its diameter. The posterior meniscofemoral band (ligament of Wrisberg) lies posterior to the PCL and may be as much as one-half its diameter. The meniscofemoral ligaments pull the lateral meniscus forward during flexion of the weight-bearing knee to maintain as much articular congruency as possible with the lateral femoral condyle.
Posterolateral Corner of the Knee
The lateral meniscus is somewhat more mobile than the medial meniscus because of the anatomy of the posterolateral corner of the knee. The arcuate complex and posterolateral corner run from the styloid process of the fibula, joining the posterior oblique ligament on the posterior aspect of the femur and tibia. The arcuate ligament is firmly attached to the underlying popliteus muscle and tendon. The tendon of the popliteus muscle separates the deep joint capsule from the rim of the lateral meniscus.
Patellofemoral Joint
The patella, a sesamoid bone embedded in the tendon of the quadriceps femoris, is an integral part of the extensor mechanism of the knee. The patella functions as an anatomical pulley, increasing the knee extension moment created by contraction of the quadriceps femoris by as much as 50%. It also guides the forces generated by the quadriceps femoris to the patellar ligament, protects deeper knee joint anatomy, protects the quadriceps tendon from frictional forces, and increases the compressive forces to which the extensor mechanisms can be subjected.12–15
Although the anterior surface of the patella is convex, the posterior surface has three distinct anatomical areas: a lateral, medial, and odd facet. The lateral and medial facets are separated by a vertical ridge. The odd facet articulates with the medial condyle at the end range of knee extension (Figure 11-6). The posterior patellar surface is covered with hyaline articular cartilage, except for the distal apex, which is roughened for the attachment of the patellar tendon. Pressure between the patella and trochlear groove of the femur increases substantially as the knee flexes. During knee flexion, the patella moves in a complex but consistent three-dimensional pattern of flexion/extension rotation, medial/lateral rotation, medial/lateral tilt (also described as wavering), and a medial/lateral shift relative to the femur.13,15 These motions occur biomechanically in the X, Y, and Z planes.
The stability of the patella is derived from the patellofemoral joint’s static structural characteristics and dynamic (muscular) control. Static stability is a product of the anatomy of the patella, the depth of the intercondylar groove, and the prominent and longer lateral condyle of the femur. The sulcus angle, formed by the sloping edges of the condyles, is normally between 114 and 120 degrees; however, it can vary significantly from person to person.16 Wiberg17 divides the patellofemoral joint into six types based on the size and shape of facets (Table 11-1). The depth of the patellar trochlea and the facet pattern are important in patellar stability.
Table 11-1
Classification of Patellar Types, Listed from Most to Least Stable17
Patellar Type | Description |
I | Equal medial and lateral facets, both slightly concave |
II | Small medial facet, both facets slightly concave |
II/III | Small, flat medial facet |
III | Small, slightly convex medial facet |
IV | Very small, steeply sloped medial facet, with medial ridge |
V (Jagerhut) | No medial facet, no central ridge |
Dynamic stability of the patellofemoral joint is derived primarily from activity of the quadriceps femoris as well as from the tensile properties of the patellar ligament (Figure 11-7). The four components of the quadriceps muscle act together to pull the patella obliquely upward along the shaft of the femur, whereas the patellar ligament anchors it almost straight downward along the anatomical axis of the lower leg. The tibial tubercle is typically located at least 6 degrees lateral to the mechanical axis of the femur.
Because the structure of the patellofemoral articulation and the muscular/ligamentous forces that act on the patella are complex, patellar dynamics involve much more than simple cephalocaudal repositioning as the knee is flexed or extended. Van Kampen and Huiskes15 describe the three-dimensional motions of the patella as flexion rotation, medial rotation, wavering tilt, and lateral shift. All of these patellar movements (except flexion) are influenced by the rotation of the tibia and the dynamic stabilization of the muscles that act on the patella.
Biomechanics of knee motion
Although it is beyond the scope of this book chapter to comprehensively cover the biomechanics of knee motion, it is important to have a basic foundation of knee biomechanics to understand the use of knee orthoses as an intervention for knee pathology. Evaluating and managing injuries of the knee requires an in-depth understanding of the biomechanical characteristics of the knee joint. The kinematics of the knee describe its motion in terms of the type and location and the magnitude and direction of the motion.18 The kinetics of the knee describe the forces that act on the knee, causing movement.18 Kinetic forces are classified as either external forces that work on the body (e.g., gravity) or as internal, body-generated forces (e.g., friction, tensile strength of soft tissue structures, muscle contraction).
Motion in the tibiofemoral joint can be best understood by separating the motion into its physiological and accessory components. Physiological motion can be controlled consciously, most often through voluntary contraction of muscle. Osteokinematic (bone movement) and arthrokinematic (joint surface motion) are examples of physiological motion. Accessory motion occurs without conscious control and cannot be reproduced voluntarily. Joint play, which is elicited by passive movement during examination of a joint, is an example of an accessory motion. The magnitude and type of accessory motion possible are determined by the characteristics of a particular articulation and the properties of the tissues that surround it. The arthrokinematics of the tibiofemoral joint will vary depending upon whether the lower-extremity is in a weight-bearing or loaded position. For example, with tibial-femoral extension the tibia moves anteriorly relative to the femur and with femoral on tibia extension the femoral condyles slide from anterior to posterior, while rolling anteriorly.8
One of the important accessory component motions of the tibiofemoral joint is its screw home or locking mechanism. In the final degrees of knee extension, the tibia continues to rotate around the large articular surface of the medial femoral condyle. This motion cannot be prevented or changed by volitional effort; it is entirely the result of the configuration of the articular surfaces. When the knee is flexed to or beyond 90 degrees, however, conscious activation of muscles can produce physiological (osteokinematic) external (lateral) or internal (medial) rotation of the tibia on the femur.
Three osteokinematic motions are possible at the tibiofemoral joint. Knee flexion/extension occurs in the sagittal plane around an axis in the frontal plane (x-axis). Internal/external rotation of the tibia on the femur (or vice versa) occurs in the transverse plane around a longitudinal axis (y-axis). Abduction and adduction occur in the frontal plane around a horizontal axis (z axis). The arthrokinematic movements of the tibiofemoral joint are rolling, gliding, and sliding (Figure 11-8). It is important to note that the roll-glide ratio is not constant during tibiofemoral joint motion: Approximately 1:2 in early flexion, the roll-glide ratio becomes almost 1:4 in late flexion.19 Rolling and gliding occur primarily on the posterior portion of the femoral condyles. In the first 15 to 20 degrees of flexion, a true rolling motion of the femoral condyles occurs in concert with the tibial plateau. As the magnitude of flexion increases, the femur begins to glide posteriorly on the tibia. Gliding becomes more significant as flexion increases.11
From a kinematic standpoint, the ACLs and PCLs operate as a true gear mechanism controlling the roll-glide motion of the tibiofemoral joint. With rupture of either or both of the cruciate ligaments, the gear mechanism becomes ineffective, and the arthrokinematic motion is altered. In an ACL-deficient knee, the femur is able to roll beyond the posterior half of the tibial plateau, increasing the likelihood of damage or tear of the posterior horn of the medial or lateral meniscus.
Because the knee has characteristics of a hinge joint and an arthrodial joint, two types of motion (translatory and rotatory) can occur in each plane of motion (sagittal, frontal/coronal, transverse). For this reason, knee motion is described as having six degrees of freedom. The three translatory motions of the knee include anteroposterior translation of 5 to 10 mm, mediolateral translation of 1 to 2 mm, and compression-distraction motion of 2 to 5 mm. The three rotatory motions occur in flexion/extension, varus/valgus, and internal (medial)/external (lateral) rotation.7,19 Hinge design of an orthosis will be discussed further in this chapter to help control these motions. Single, dual, or multiple hinged orthoses that do not conform with the A/P translation (or roll back) and the rotation of the joint, will exert adverse mechanical effects.20
Orthoses for the Knee
As discussed previously, there are a few different categories of orthoses for the knee. A sample of the many types of knee orthoses that are commercially available to health professionals is listed in Table 11-2. The clinician should be aware that the brace names tend to change. Some are designed to immobilize the knee after surgical repair of damaged or ruptured ligaments or menisci during the acute phases of healing, whereas other knee orthoses are used to improve biomechanical efficiency at the knee joint and/or prevent injury. Rehabilitative orthoses are designed to protect the knee and allow progressive increase in active range of motion during rehabilitation. FKOs provide additional protection as rehabilitation is completed and a patient returns to normal activities. Prophylactic knee orthoses, attempt to prevent injury (or at least lessen the extent of injury) in athletes who are at risk of injury during competition. A patellofemoral knee bracing allows for improved tracking of the patella during knee motions, whereas unloader knee braces dissipate increased varus and valgus forces across the tibiofemoral joint. The complexity of the knee joint (with its polycentric axis of rotation for flexion/extension, asymmetry of the lateral and medial compartments, and the arrangement of its muscular and ligamentous attachments) creates a challenge when trying to design a knee orthosis that is able to support, protect, and stabilize the knee in a multiplanar fashion with the same efficiency as its own physiological motion.
Table 11-2
A Few Examples of Knee Orthoses by Manufacturer and Category
Manufacturer | Rehabilitative Knee Orthoses | Functional ACL/PCL | Functional Osteoarthritis | Prophylactic | Patellofemoral |
Bauerfeind USA Kennesaw, Ga. (800) 423-3405 www.bauerfeindusa.com | Softech GenuSecutech Genu | Softtech OA | Genutrain P3 | ||
Bledsoe by Medical Technology Grand Prairie, Texas (888) 253-3763 www.bledsoebrace.com | Extender Plus Leverlock Revolution 3 | Ultimate Dynamic U2CI Kneecage Forcelite | Thruster RLF Aligner ESE | 20.50 Patella- femoral | |
Brace International Atlanta, Ga. (800) 545-1161 www.braceint.com | FLUK Infrapatellar Strap | ||||
Cropper Medical, Inc./Bio Skin Ashland, Ore. (800) 541-2455 www.bioskin.com | Gladiator Sport | Standard Knee Skin | Q Lok Patella Stabilizer Q Baby | ||
dj Orthopedics, Inc. Vista, Calif. (800) 336-6569 www.djortho.com | IROM TelescopingIROM Bilateral with Shells | Defiance III Armor Legend 4titude | OA DefianceOA Full Force OA Assist | Protective-Knee Guard Playmaker | TruPull Wraparound H-Buttress |
Generation II USA, Inc./Ossur Bothell, Wash. (800) 462-7252 www.gen2.com | Generation II 3DX Matrix Extreme Select GII Trainers | Unloader Select Unloader Adj Unloader Spirit Unloader Bi-Com | Patellar StabilizerPS1 | ||
Innovation Sports, Inc. Irvine, Calif. (800) 222-4284 www.isports.com | Sentry Knee MD Quicklock Option | CTi Edge C180 XCI Aspire CTI2 | CTI2OA | Powerflex Donut Stabilizer Lateral J Stabiliz | |
McDavid Sports/Medical Products Woodridge, Ill. (800) 237-8254 www.mcdavidusa.com | 428R Mcdavid Level 3 Knee Brace with Polycentric Hinges | 419R McDavid Level 2 KneeSupport | |||
Pro Orthopedic Devices, Inc Tuscon, Ariz. (800) 523-5611 www.proorthopedic.com | PRO 190 Hinged Stabilizing Knee Brace | PRO 105 Spiral Knee Support Sleeve Patellar Strap PRO 135 | |||
Townsend Design Bakersfield, Calif. (800) 700-2722 www.townsenddesign.com | ROM-SS EZ-Pads | Premier Series Rebel Series Sports Series | RelieverOne |
ACL, Anterior cruciate ligament; PCL, posterior cruciate ligament.
Rehabilitation Knee Orthoses
Orthoses used in the postoperative and early rehabilitation of patients who have had surgical repair of damaged cartilage, ligaments, or bone are designed to control knee motion carefully to minimize excessive loading on healing tissues (Figures 11-9 and 11-10).3 Although many rehabilitation knee orthoses are on the market, an effective orthosis has several important characteristics. The orthosis must be adjustable to accommodate changes in limb girth due to edema or atrophy. It must remain in the desired position on the limb during functional activities in stance and in sitting. It must be comfortable, easy to don and doff, durable, and economical. Most physicians require an adjustable knee unit so that active range of motion can be increased incrementally as the tissue heals and the patient’s condition improves. The ability to move in carefully controlled ranges of motion is thought to improve ligamentous strength and to minimize the risk of scar formation in the intracondylar notch that is associated with flexion contracture.19
Functional Knee Orthoses
Use of knee orthoses as functional braces parallels the development of the discipline of sports medicine since the early 1970s.21 In the years before 1970, orthoses that were designed for patients with neuromuscular dysfunction were adapted to meet the needs of injured athletes with a functionally unstable knee. Typically, the adapted orthosis was cumbersome and significantly impaired the quality of the athlete’s performance. To assist professional athletes in quickly and safely returning to competition, the sports medicine community explored alternative orthotic interventions to manage an unstable knee. The development of FKOs as alternatives to surgery (which often sidelined athletes for considerable periods of time) was especially welcomed.22
One of the first FKOs, the Lenox Hill Derotation knee brace, was developed by Nicholas and Castiglia of Lenox Hill Hospital in the early 1970s to protect the chronically unstable knees of football quarterback Joe Namath.23 The Lenox Hill orthosis was the primary design that was commercially available until the late 1970s. The original orthosis had medial and lateral uprights, a single-axis knee joint with a medial fulcrum pad, a broad elastic thigh and calf cuff/strap to hold the orthosis in place, and a set of derotation straps to control movement of the tibia on the femur. The intent was to control mediolateral instability, rotational instability, and multiple ligament impairment.24 Subsequent versions of the Lenox Hill orthosis are available in standard, lightweight, and ultra-lightweight versions, with a dial-lock option to adjust available range of motion and with protective undersleeves and oversleeves (Figure 11-11).
The original design has evolved considerably: More than three dozen functional braces are manufactured in today’s sports medicine market (see Table 11-2 for examples). Most are based on a lightweight rigid suprastructure made from carbon composite or titanium alloy. Many use adjustable elasticized or Velcro strapping to apply a four-point stabilizing force and hold the orthosis in position on the limb. Some have polycentric knee units; others allow variable flexion control or assisted extension. The basic design and components of most commercially available FKOs are shown in Figure 11-12.
According to a survey of orthopedic surgeons performed by Decoster and Vailas,25 87% of surgeons brace their patients who have had ACL reconstruction. In addition, DeCoster and Vailas found that typically the surgeons are more likely to prescribe “off the shelf” braces for patients with ACL deficiency compared to those patients with ACL reconstruction.25
Unfortunately, systematic critical evaluation of the efficacy of FKOs has not kept pace with the development of new designs; there is a paucity of clinical and laboratory research defining the indications for, roles of, or outcomes of use for these functional braces.22
Functional Knee Orthoses and Performance
Current available literature can be characterized by two major approaches to comparing braced and unbraced performances: (1) maximal effort tests, wherein subjects performed at maximal intensity and the criterion measure was an overall performance measure such as distance hopped or the time to run a specified distance; (2) matched effort tests, wherein subjects perform at submaximal intensity and the criterion measures tend to be specific variables such as muscle activation patterns determined via electromyography (EMG) or ground reaction forces determined via force plate.26 Nemeth and colleagues27 studied six expert downhill skiers who had sustained ACL injuries. Surface electrodes were used with an eight-channel telemetric EMG system to collect recordings from the vastus medialis, biceps femoris, semimembranosus, semitendinosus, and gastrocnemius medialis muscles from both legs. Without a brace, the EMG activity level of all muscles increased during knee flexion. With a brace, the EMG activity increased in midphase during the upward push for the weight transfer and the peak activity occurred closer to knee flexion in midphase. The authors suggest the brace caused an increase in afferent input from proprioceptors, resulting in an adaptation of motor control patterns secondarily modifying EMG activity and timing.27 Research has also shown that the use of functional knee bracing seems to protect the ACL from sagittal plane shearing in non–weight-bearing and weight-bearing, and internal torques in non–weight-bearing. However, the study also found that the braced knee did not have any decrease in strain in external torques or valgus-varus stressors in weight-bearing and non–weight-bearing when compared with the unbraced knee.28
Additionally, Sterett and colleagues29 found that following ACL reconstruction, those skiers who did not use a functional brace during skiing had a higher rate of reinjury.
A number of researchers have investigated the impact of functional braces on performance and endurance in noninjured and previously injured athletes. Stephens30 found little impact on speed of running in noninjured collegiate basketball players during straight line running tasks (end line to foul line, full court) when comparing performance in two functional braces with nonbraced speed. Although these results are positive, the in-brace time and types of activity used for the study were significantly different than those of an active, full length basketball game.
Highgenboten and colleagues31 found a 3% to 6% increase in metabolic cost during steady-state treadmill running when functional braces were worn. Subjects also had higher ratings of perceived exertion when exercising with the brace. These effects were attributed to the weight of the brace. In addition, research by Wojtys and colleagues32 showed that most braces appear to slow hamstring muscle reaction times at voluntary levels. The evidence from these maximal effort and matched effort performance tests suggest no advantage to use of FKOs.
Most functional knee braces weigh close to 1 pound. Despite the use of lightweight materials that are resilient to various forces, it is possible that long in-brace times can lead to fatigue or to injuries to other areas of the body that compensate for the added weight of the orthosis. This may explain the increase in injuries of the foot and ankle reported by Grace and colleagues33 in athletes who wore braces. Styf and colleagues34 studied changes in intramuscular pressures within the anterior compartment of the leg at rest, during exercise, and after exercise, comparing three orthoses. Intramuscular pressures at rest and muscle relaxation pressure during exercise were higher when subjects wore each of the orthoses. To evaluate whether distal strapping was responsible for this increase in pressure, distal straps were removed and subjects retested; intramuscular pressure and muscle relaxation pressure returned to levels that were similar to nonbraced levels. This study demonstrates the subtle yet potentially important impact of orthoses on muscle function that may contribute to injury; external compression elevates the intramuscular pressure beneath the thigh and leg straps. Local muscle blood flow is impaired by the increased intramuscular pressure, which leads to decreased oxygen tension and impaired muscle function.35 This could possibly be the reason for premature leg muscle fatigue that has been reported in other experimental studies on individuals using knee braces.34,36,37
In their randomized, multicenter trial, McDevitt and colleagues38 compared patients who wore an off-the-shelf knee brace daily for 6 months and for high-level activities thereafter with a group who did not wear any brace after 3 weeks postoperatively. The authors found that there were no significant differences between groups in multiple outcome measures, which included knee range of motion, knee stability, and function.38
Prophylactic Knee Orthoses
In the 1990s, considerable debate took place concerning the potential benefits or effectiveness of orthoses that were designed to prevent knee injuries or to minimize the severity of injury for athletes involved in high-risk injury activities.33,39–41 The objective of wearing a prophylactic knee orthosis (PKO) is to provide protection to the soft tissue restraints of the knee. Most commonly, these braces have been worn by athletes who are exposed to lateral impacts.
Lateral impacts can result in MCL and cruciate ligament injuries.42 Prophylactic orthoses are designed to protect the integrity of medial knee structures. Most prophylactic designs have a hinged lateral frame, held in place by a set of thigh and calf cuffs or straps. The hinge may be single axis, dual axis, or polycentric; many designs have also incorporated a hyperextension stop for further protection of the athlete’s knee against forceful hyperextension injury (Figure 11-13). Some designs use a plastic shell with medial and lateral uprights and polycentric hinges.43
Recommendations for the Patient with a Prophylactic Knee Orthosis
PKOs are available off-the-shelf or can be custom molded for the individual athlete. Clinical practice and research have not yet resolved the debate about the efficacy of prophylactic orthoses. Questions remain about the relationship between the biomechanical characteristics of the orthoses and the anatomical knee, the response of the orthosis to valgus loading, the impact of the orthosis on the continuum of locomotion (walking to running), and the ability of the orthosis to prevent injuries during activity as intended by its design.
Biomechanical Performance of Prophylactic Knee Orthoses
Many of the studies that have investigated the biomechanical performance of prophylactic braces have used either cadaver knees or mechanical surrogate models of the knee. Most have concluded that protection of the MCL has been inconsistent and only borderline at best.40,44–48 Many health care providers are concerned about how the orthosis might preload the MCL before normal physiologic loading.44,46,47 Our understanding of the interaction between the orthosis and the tissues of the knee is based on the properties of human tissue and of brace materials under static and dynamic loading conditions.
Manufacturers report high resistance of the braces to laterally directed impact loading; however, the research design and methodology on which these claims are based can be debated.
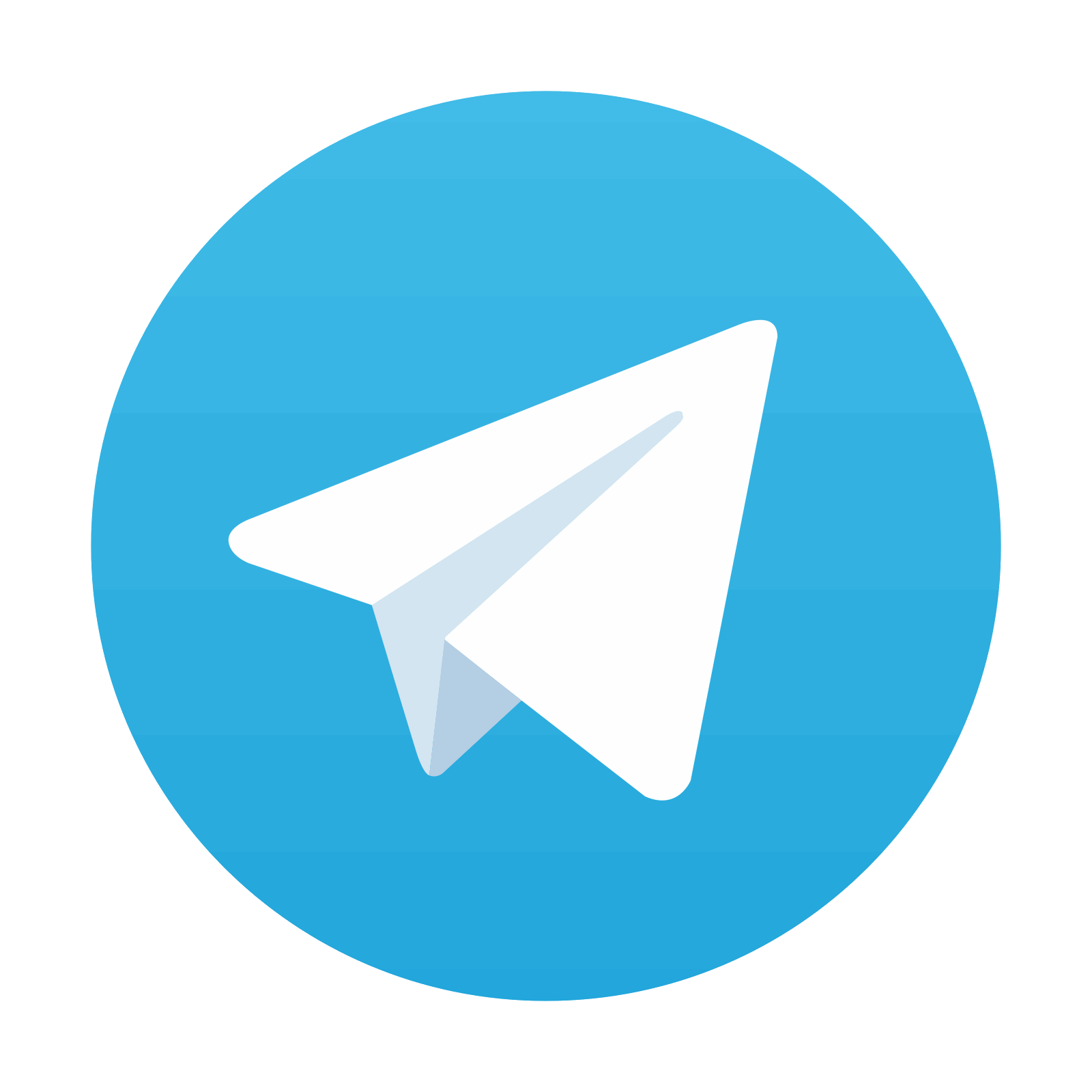
Stay updated, free articles. Join our Telegram channel

Full access? Get Clinical Tree
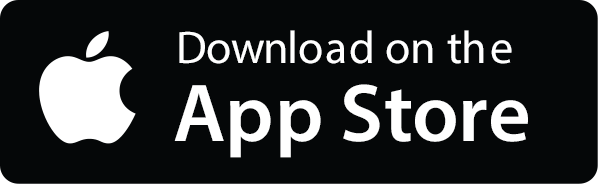
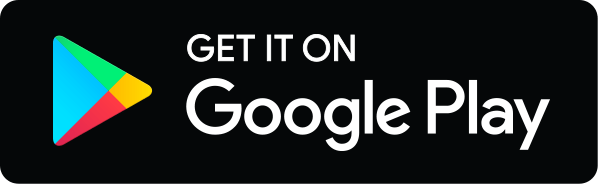