Foot Orthoses
Roberta Nole, Donald S. Kowalsky, Juan C. Garbalosa, Elicia Pollard and John Thompson
Learning Objectives
On completion of this chapter, the reader will be able to do the following:
3. Explain strategies used to examine and evaluate intrinsic foot deformities.
6. Discuss controversy related to traditional orthotic theory.
7. Review literature related to the efficacy of foot orthoses for the management of common disorders.
History of the functional foot orthosis
The use of foot orthoses as an effective treatment tool for biomechanical dysfunction of the feet evolved during the twentieth century and continues to be the subject of research and technology. Early on, foot orthoses were used to redistribute plantar surface foot forces to alleviate discomfort in pressure- sensitive areas of the foot. Little consideration was given to the specific foot abnormality that led to the pathological condition.1 In the early 1900s metal foot braces began to be used to control motion at specific joints of the foot and prevent pathological conditions.2 These devices, although functional, were often not well tolerated because of the rigidity of the materials and the mismatch between brace design and foot pathokinesiology. In 1948, Schreber and Weineman first identified forefoot invertus (varus) and evertus (valgus) as primary foot deformities that required correction by an orthosis.3 In the 1960s, Merton Root developed neutral impression casting techniques, positive cast modifications, and posting (mechanical correction) techniques.4 The standards that Root established have enhanced orthotic comfort and function. Since then, functional foot orthosis use has increased considerably to address lower limb muscle activation pathology of the mechanics of the foot and ankle in musculoskeletal disorders and neurological disorders.5–11
Triplanar structure of the foot
The foot is a complex of bones interconnected by a series of multiplanar articulations supported by soft tissue structures. It is subdivided into three functional components: the rearfoot, the midfoot, and the forefoot.
Several important articulations of the foot (talocrural, subtalar, midtarsal, first and fifth rays) are triplanar; the axis of rotation in these joints is not perpendicular to any of the cardinal planes (sagittal, horizontal, frontal) of the human body. As a result, motion about triplanar joints leads to simultaneous movement in all three of these cardinal planes.4 The amount of motion evident in any single plane is related to the pitch (inclination) of the triplanar axis from the respective cardinal plane. Triplanar motion occurs in three-dimensional space; the breakdown of triplanar motion into its three constituent cardinal plane movements is artificial.
Because motion about a triplanar axis is three dimensional, motion occurs simultaneously in the three cardinal planes. Blocking any one component of triplanar motion in a single cardinal plane prevents movement in the other two planes as well. This “all-or-nothing” rule is the premise for orthotic posting or wedging.4 Theoretically, the addition of a post or wedge to an orthosis blocks the frontal plane component of triplanar motion, which in turn blocks or limits the triplanar motion of pronation. The design principles of foot orthoses are founded on knowledge of the functional anatomy of the foot.
Talocrural Joint
The talocrural joint (TCJ) (articulation between tibia, fibula, and talus, connecting the foot to the lower leg) has a triplanar axis of rotation. In neutral position, the TCJ axis passes through the tips of the medial and lateral malleoli, pitched 10 degrees from the transverse plane and 20 to 30 degrees from the frontal plane (Figure 8-1).12–14 Although sagittal plane plantarflexion and dorsiflexion are primary motions at this joint, the slight inclination of the TCJ axis of rotation leads to concomitant transverse and frontal plane motion. During plantarflexion, the foot adducts and inverts; with dorsiflexion it abducts and everts. Normal range of motion (ROM) of the TCJ is between 12 and 20 degrees of dorsiflexion and 50 and 56 degrees of plantarflexion.15 The medial (deltoid) and lateral collateral ligaments stabilize and limit motion that occurs at the TCJ.16
Rearfoot
The osseous structures of the rearfoot are the calcaneus (inferior) and the talus (superior) (Figure 8-2). The articulation between the calcaneus and talus is the subtalar joint (STJ). Three joint surfaces are present in this articulation: posterior, anterior, and middle. The posterior joint surface has a concave talar and convex calcaneal portion, whereas the anterior and middle joint surfaces have convex talar and concave calcaneal arrangements. This structurally based articular geometry, along with the interosseous talocalcaneal ligament, limits the amount and type of motion occurring at the STJ.17,18 The medial and lateral collateral ligaments and the posterior and lateral talocalcaneal ligaments also offer support to the STJ.19
At the STJ, the triplanar axis of rotation is oriented in an anterosuperior to posteroinferior direction, pitched approximately 42 degrees from the transverse plane, 48 degrees from the frontal plane, and 16 degrees from the sagittal plane (see Figure 8-2). The location of the STJ axis in the human foot varies greatly. Manter20 reported that the inclination from the transverse and sagittal planes varies from 29 degrees to 47 degrees and from 8 degrees to 24 degrees, respectively.
The triplanar motions at the STJ are supination and pronation. Supination of the weight-bearing foot leads to dorsiflexion and abduction of the talus with simultaneous inversion of the calcaneus. Pronation of the weight-bearing foot results in plantarflexion and adduction of the talus and eversion of the calcaneus.4 Because of the variability in location of the axis of rotation of the STJ, the component motions of supination and pronation vary as well. As the axis becomes more perpendicular to a particular cardinal plane, the motion occurring in that plane becomes more pronounced, whereas the other motions become less prominent.21,22 This variability affects coupled motion between the joints of the foot and the lower leg. During pronation and supination of the rearfoot, the tibia and fibula rotate internally and externally in the transverse plane.4,23,24 An increase in the frontal plane motion of the rearfoot could cause a simultaneous increase in the transverse plane motion of the lower leg.
Midfoot
The midfoot is composed of two bones: the cuboid and the navicular. The talonavicular and calcaneocuboid articulations between the midfoot and rearfoot form an important composite joint: the midtarsal joint (MTJ) or transverse tarsal joint. The articular surfaces of the talonavicular joint are convex-concave, whereas the surfaces of the calcaneocuboid joint are sellar shaped.13,20 MTJ movement is supported and restricted by the bifurcate, short and long plantar, and plantar calcaneonavicular (spring) ligaments. The short and long plantar ligaments and the plantar calcaneonavicular ligaments also support the longitudinal and transverse plantar arches of the foot.25
Because the MTJ is a composite joint, motion occurs about two separate triplanar joint axes: a longitudinal and an oblique axis (Figure 8-3). Movement of the forefoot about each joint axis can occur independently of the other. Manter20 reported that the longitudinal axis is inclined superiorly 15 degrees from the transverse plane and medially 9 degrees from the sagittal plane, whereas the oblique axis is pitched superiorly 52 degrees from the transverse plane and medially 57 degrees from the sagittal plane. The predominant motion about the longitudinal axis is frontal plane inversion and eversion. Because of the slight deviation of the longitudinal axis from the three cardinal planes, small amounts of forefoot plantarflexion and dorsiflexion and adduction and abduction occur during inversion and eversion. Plantarflexion and dorsiflexion and abduction and adduction are the predominant movements around the oblique MTJ axis, with little concomitant inversion and eversion.4,18
These two joint axes produce the combined motion of supination and pronation of the MTJ. During supination and pronation, the forefoot inverts and everts about the longitudinal axis. The motion around the oblique axis is plantarflexion with adduction and dorsiflexion with abduction. The amount of motion possible at these MTJ axes is determined by the position of the STJ. In STJ supination, the two joint axes are nearly perpendicular so that MTJ mobility is restricted. This mechanism helps convert the forefoot into a rigid structure for propulsion during the push-off phase of gait (from heel rise through toe-off).20,26 When the STJ is pronated, the joint axes are more parallel, allowing a greater degree of MTJ mobility.
Forefoot
The forefoot includes all structures distal to the navicular and cuboid bones; it is subdivided into five rays and toes. The first through third rays consist of a cuneiform and its associated metatarsal bone; the fourth and fifth rays consist only of a metatarsal. The tarsometatarsal joints, the primary joints of the ray complexes, have two opposing planar surfaces.4,25 The hallux, or first toe, has two bones (a proximal and distal phalanx) and two corresponding joints (metatarsophalangeal [MTP] and interphalangeal [IP]). The lesser toes have three bones (proximal, middle, and distal phalanges) and three associated joints. The proximal articular surfaces of the MTP and IP joints are convex, and the distal articular surface is concave.4 Numerous soft tissue structures support these joints.27,28
Although each ray has its own axis of motion, the first and fifth rays are of particular interest. The triplanar axes of rotation of these two joints are nearly perpendicular. The axis of the first ray is pitched at a 45-degree angle from the sagittal and frontal planes; the primary motions possible are plantarflexion with eversion and dorsiflexion with inversion. Because the axis of the first ray is minimally pitched from the transverse plane, insignificant transverse motion occurs.4 In contrast, the axis of rotation of the fifth ray is oriented at a 20-degree angle from the transverse plane and a 35-degree angle from the sagittal plane. The resulting motions combine inversion with plantarflexion and eversion with dorsiflexion. Less motion is present about the axis of rotation of the fifth ray than of the first ray.4 MTP joints have two separate axes of rotation: the vertical axis (abduction and adduction) and the transverse axis (plantarflexion and dorsiflexion).4,13,25 Little frontal plane motion at MTP joints is normal; frontal plane motion leads to subluxation.4
Plantar Fascia and Arches of the Foot
The plantar aponeurosis, one of the most functionally important soft tissue structures of the foot, is a sheath of fascia spanning most of the foot’s plantar surface. Arising from the medial process of the calcanean tuberosity, it passes distally along the plantar aspect of the foot, then divides into five slips for its distal attachment at the base of the proximal phalanges by the plantar pads.29 This fascial sheath plays an extremely important role in providing the stability needed by the foot during the toe-off phase of stance during gait and in supporting the longitudinal arch of the foot.
The medial longitudinal and transverse arches are formed by the ligamentous and osseous structures of a “normal” foot. 30 The medial longitudinal arch (MLA) extends from the calcaneus (posterior) to the first metatarsal head (anterior) and is supported by the plantar aponeurosis, short and long plantar ligaments, and the spring ligament. During weight bearing, the height of the arch is reduced as the supporting ligamentous structures are elongated. The transverse arch reaches across the foot from medial to lateral borders. The height of the arch varies along the length of the foot: Its maximum height occurs at the cuboid-cuneiform bones of the midfoot, and its lowest point is at the metatarsal heads.
Function of the foot in gait
The foot and ankle complex has three major functions in the gait cycle: attenuating the impact forces, maintaining equilibrium, and transmitting propulsive forces. For optimal biomechanical and energy-efficient performance, the joints of the foot and ankle must work in harmony. In early stance, the foot-ankle complex absorbs energy generated at initial contact and decreases forces transmitted to proximal structures during loading. The foot and ankle must also adapt to surface conditions encountered by the foot as stance begins. In late stance, the foot and lower leg transmit propulsive forces generated by muscles of the lower extremity onto the ground. The ability of the foot and lower leg to accomplish these functions depends on the integrity of the various structures of the foot. Gait abnormalities occur when the foot and ankle complex is unable to compensate for deficits in motion or structure.
The kinematic, kinetic, and neuromuscular events of the normal human gait cycle have been described in many ways.4,31,32 Most focus on five distinct events: initial contact (IC) or heel strike, loading response (LR) or foot flat, midstance (MSt), terminal stance (TSt) or heel-off, and preswing (PSw) or toe-off. See Chapter 5 for a more detailed description of the gait cycle.
Shock Absorption
Musculoskeletal structures of the lower limb act from IC to MSt to attenuate impact forces.4,31,33 Force plate recording of ground reaction forces (GRFs) estimates the foot’s ability to absorb energy and decelerate the lower leg. The push of the foot against the floor creates a GRF with three components: vertical, medial and lateral, and fore and aft forces. The vertical component of a typical GRF record has a bimodal shape (Figure 8-4). The brief first peak results from the impact of the heel with the ground. Some of the vertical GRF is attributed to acceleration of the centers of mass of the foot and shank of the leg.
In early stance, from IC to LR, the STJ moves into pronation as the TCJ is plantarflexing.4,31,34,35 The fibula and tibia internally rotate with respect to the foot.22,23 Pronation of the STJ is controlled by eccentric contraction of the tibialis anterior, posterior tibialis, flexor hallucis longus, and flexor digitorum longus muscles.4,31,36 Plantarflex of the foot is controlled primarily by eccentric action of the tibialis anterior.4,35 The combined muscle activity decelerates plantarflex and pronation motion of the TCJ, STJ, and MTJ, slowing vertical and anterior movement of the center of mass of the foot and shank and decreasing impact forces encountered at IC.
The viscoelastic plantar fat pad absorbs some of the energy generated between IC and LR.37 Pronation of the STJ flattens the arches of the foot, elongating plantar connective tissue structures. Because these tissues are viscoelastic, they also absorb some of the energy generated from IC to LR.
Adaptation to Surfaces
In everyday walking, the foot must be able to adapt quickly to many types of terrains and uneven surfaces. The key contributor to surface adaptation is STJ pronation, which unlocks the MTJ, permitting the joints of the foot to function in loose-packed positions and enabling the osseous elements to shift their relative positions.
At IC, the forefoot is in a supinatory twist (inverted) about the longitudinal MTJ axis. Eccentric action of the anterior tibialis decelerates plantarflex of the forefoot, lowering it to the ground. The MTJ becomes fully supinated at LR, as a result of eversion of the STJ and GRFs acting upward on the foot’s medial border. Contraction of the extensor digitorum longus and peroneus tertius abducts and dorsiflexes (pronates) the forefoot, locking it about the oblique MTJ axis and preparing the forefoot to receive the loading forces encountered at MSt.4
Propulsion
During MSt (LR to PSw), the STJ is maximally pronated and begins to resupinate. At this time, the GRF maintains the MTJ in a pronated position about its oblique axis. At the same time, a pronatory twist is initiated at the longitudinal axis by concentric action of the peroneals. The MTJ locks in a fully pronated position around the longitudinal axis just before heel rise, as the STJ reaches its neutral position. The MTJ must remain locked in this position throughout propulsion, as the peroneals contract to lift the lateral side of the foot from the ground and transfer weight medially to the other foot. As the heel is raised from the ground, the rearfoot continues to supinate (talus abducts and dorsiflexes) as the lower limb rotates externally. This coupled motion necessitates supination of the MTJ about the oblique axis to maximize joint stability and convert the foot into a rigid lever for propulsion.
Supination of the STJ occurs with concentric action of the tibialis posterior, flexor hallucis longus, and flexor digitorum longus and soleus, as well as the antagonistic functioning of the peroneus brevis.4,31 The concentric activity of the gastrocnemius and soleus muscles causes vertical acceleration of the foot and lower leg. Propulsive forces generated by the foot and lower leg are transmitted to the floor.4,31,35 The second peak of a GRF curve corresponds to propulsion in late stance phase (see Figure 8-4).
Supination of the STJ and locking of the MTJ about the longitudinal axis place the foot in a closed-packed position, transforming the foot into a rigid lever.4,14,23,31 This transformation is aided by the action of the plantar aponeurosis as it wraps around the metatarsal heads. During TSt, the MTP joints extend (dorsiflex), creating a “windlass effect.” This action compresses joints of the midfoot and forefoot, facilitating the transition from flexibility to rigidity required for effective push-off.
Biomechanical examination
The biomechanical examination of the foot and ankle has three components: a non–weight-bearing assessment, a static weight-bearing assessment, and a dynamic gait analysis (Figure 8-5). Five common intrinsic foot deformities are identified in the biomechanical examination: rearfoot varus, forefoot varus, forefoot valgus, equinus deformity, and plantar flexed first ray.
The theoretical model of biomechanical foot and ankle examination is based on the work of Root and colleagues.4 Debate continues about the validity of Root’s criteria for normalcy and the assumption that the STJ is in neutral position from MSt to TSt of the gait cycle. Reliability of the measurement techniques used to determine STJ neutral position has also been questioned.38–40 Although controversial, Root’s theory and his biomechanical evaluation and treatment techniques are used by many clinicians.
Root describes deviations from normal foot alignment as “intrinsic” foot deformities, which can lead to aberrant lower extremity function and musculoskeletal pathological conditions.4,41 To prescribe an appropriate biomechanical foot orthosis, the source of the pathological condition or deformity must be determined by a detailed patient history and a comprehensive biomechanical examination.42 This helps the clinician identify resultant pathomechanical abnormalities and determine the benefits of orthotic intervention.
Non–weight-bearing open chain examination
During the non–weight-bearing open chain examination, the basic architecture of the foot and ankle is assessed. Any bony deformities or prominence of the joints, rays (toes), and callosities are noted. The examiner uses a goniometer to locate the subtalar neutral (STN) position and identify any intrinsic foot deformities.
The non–weight-bearing goniometric examination is performed with the patient in the prone position, with the targeted lower extremity positioned with extended knee, and the foot 6 to 8 inches off the treatment table. Placing the contralateral lower extremity in a figure-four position orients the ipsilateral lower extremity in the frontal plane, reducing the influence of proximal rotational limb disorders on measurement.43
Examination of the Rearfoot
Goniometric measurements of the non–weight-bearing examination assess the rearfoot with respect to STN position, as well as STJ mobility, based on calcaneal positioning in the frontal plane. Calcaneal frontal plane motion is the most readily examined component of triplanar STJ motion. To perform the examination, the stationary arm of a goniometer is aligned with an imagined bisection of the lower third of the limb (tibiofibular complex), the mobile arm is aligned with an imaginary bisection of the posterior surface of the calcaneus, and the axis of the goniometer is aligned at the STJ axis, just above the superior border of the calcaneus but beneath the level of the medial and lateral malleoli (Figure 8-6).43,44
Subtalar Neutral Position
STN position can be manually estimated by palpation or by using a mathematical model developed by Root. If using palpation, the examiner identifies the anteromedial andanterolateral aspects of the talar head with the thumb and index fingers of the hand closest to the patient’s midline, placing the thumb just proximal to the navicular tuberosity, approximately 1 inch below and 1 inch distal to the medial malleolus (Figure 8-7). In STJ pronation, the anteromedial talar head is most prominent beneath the thumb, and an anterolateral sulcus (the sinus tarsi) is apparent. The index finger is placed in this sulcus, where the talar head is found to protrude when the foot is fully supinated. The thumb and index finger of the other hand grasp the fourth and fifth metatarsal heads, moving the foot in an arc of adduction and inversion (supination) and abduction and eversion (pronation). STN is the point where the talar head is equally prominent anteromedially and anterolaterally.41,43,44 The examiner then “loads” the foot by applying a dorsally directed pressure against the fourth and fifth metatarsal heads until slight resistance is felt. The loading procedure locks the MTJ against the rearfoot, mimicking GRFs of MSt.4,45 The angular relation between the bisection of the calcaneus and the bisection of the lower third of the leg is measured with a goniometer (see Figure 8-6), recorded on the evaluation form as rearfoot STN position.
Root’s mathematical model for determining STN position uses a quantitative goniometric formula.4,41 First, end ROM calcaneal inversion and eversion are determined by goniometric measurement. Total calcaneal ROM is the sum of the inversion and eversion values. The STN position is determined as the calcaneus is moved into inversion at one third of the total calcaneal ROM. If end-range calcaneal inversion is 25 degrees and end-range calcaneal eversion is +5 degrees, total calcaneal ROM would be 30 degrees. STN position is calculated to be at 5 degrees calcaneal inversion, one third of the distance from its fully everted position.
Reliability and clinical validity of both models have been debated.40,41,43,46–48 Although acceptable reliability is possible, it is influenced by examiner experience. Palpation to determine STN position is efficient in terms of time but requires more advanced manual skills and experience than the mathematical model. The mathematical model may be more reliable for the entry-level practitioner.
Calcaneal Range of Motion
Calcaneal inversion and eversion occur primarily at the STJ, with lesser contributions from the TCJ. Calcaneal ROM is assessed with the patient in the prone position with the same anatomical landmarks and lines of bisection as for STN assessment. The examiner grasps the calcaneus in one hand, fully inverts it in the frontal plane until end ROM is achieved, and then takes a goniometric measurement.48 The procedure is repeated for calcaneal eversion. The TCJ must be maintained in a neutral to slightly dorsiflexed position while measuring to lock it in a closed-packed position and better isolate STJ motion.49 Normative values of 20 degrees for calcaneal inversion and 10 degrees beyond vertical for eversion have been reported.4
Because values of calcaneal eversion are larger when assessed in a full weight-bearing position, some clinicians suggest that this position is more clinically valid.38,48 Assessment of calcaneal eversion in the weight-bearing position represents a total “functional” pronation and eversion that is the summation of motion occurring at the STJ and compensatory motion occurring extrinsic to the STJ (e.g., TCJ, MTJ). Passive assessment of calcaneal eversion in non–weight bearing remains the most accurate method to determine the degree of composite pronation acquired from the STJ itself.
Talocrural Joint Range of Motion
In normal walking, the TCJ is maximally dorsiflexed just before heel rise when the knee is fully extended and the STJ is in a nearly neutral position.4,50 When and whether an actual STN position ever occurs during gait is disputed.38 The use of a standard position of knee extension and STN, however, offers a consistent point of reference when assessing TCJ dorsiflexion. According to most sources, a minimum of 10 degrees TCJ dorsiflexion is required for normal gait; anything less is classified as an equinus deformity.4,50–53 A minimum of 20 degrees of plantarflex is also required for normal gait.4
Ankle dorsiflexion is measured in a non–weight-bearing position with the STJ held in the neutral position and the knee extended. The examiner forcefully dorsiflexes the ankle with active assistance from the patient. Active assistance encourages reciprocal inhibition of the calf muscle group and is essential for accurate measurement.50 The proximal arm of the goniometer is positioned along the lateral aspect of the fibula, the distal arm along the lateral border of the fifth metatarsal, and the axis distal to the lateral malleolus.54 An alternative placement of the distal arm of the goniometer along the inferolateral border of the calcaneus may more effectively isolate true TCJ dorsiflexion (Figure 8-8). This value is recorded as rearfoot dorsiflexion. Forefoot dorsiflexion is measured by repositioning the distal arm along the lateral aspect of the fifth metatarsal. This method allows the examiner to identify contributions or restrictions in sagittal plane motion from the oblique axis of the MTJ.
If ankle dorsiflexion is less than 10 degrees when measured with the knee extended, remeasurement with the knee flexed may rule out soft tissue restriction of the gastrocnemius-soleus complex.4,50 If dorsiflexion values are consistent in both positions, the limitation is likely a result of osseous equinus formation of the ankle.
During gait, ankle dorsiflexion occurs in a closed kinetic chain as the tibia and fibula rotate forward over a fixed foot. On the basis of this, some have suggested that assessing ankle dorsiflexion may be more accurate with the patient in a weight-bearing position.38,52 The weight-bearing technique measures the angle between the tibia and the floor as the patient leans forward with the foot flat on the floor. Unwanted compensations are often difficult to control during weight bearing and may mask true TCJ limitations. The non–eight-bearing technique allows the examiner to assess end feel and joint play, as well as mechanical blocks or joint laxity, providing additional information not accessible in the weight-bearing examination.
Rearfoot Deformities
Normal rearfoot position is one in which STN is 1 to 4 degrees of varus.39,40,47,48 Values of more than 4 degrees are described as a rearfoot varus deformity. This deformity may be the result of ontogenetic failure of the calcaneus to derotate sufficiently during early childhood development.4,45,55 Because this deformity is a torsional structural malalignment of the calcaneus, not a joint-related problem, the TCJ and STJ lines remain congruent when observed in the non–weight-bearing STN position. As a structural deformity, it cannot be corrected or reduced by joint mobilization or a strengthening program. Instead, it is managed with a functional foot orthosis that partially supports the calcaneus in its inverted alignment while preventing excessive STJ pronation.
Assessment of calcaneal ROM predicts quality of motion and the integrity of the STJ. Measuring calcaneal motion into eversion allows the examiner to determine whether a rearfoot deformity is compensated or uncompensated (Figure 8-9).
In a compensated rearfoot varus deformity, the calcaneus fully everts to vertical or beyond in weight bearing because the STJ possesses an adequate amount of pronatory motion to compensate for the deformity. A compensated rearfoot varus deformity of 10 degrees (STN position) requires that the STJ pronate or evert at least 10 degrees to enable the medial condyle of the calcaneus to achieve ground contact in weight bearing. Such excessive pronatory motion causes medial gapping and lateral constriction at the STJ line and a medial bulge of the talus as it moves into adduction and plantarflex.
In an uncompensated rearfoot varus deformity, the calcaneus remains fixed in its inverted STN position with no eversion motion at the STJ. A partially compensated rearfoot varus deformity allows for partial STJ eversion so that the medial condyle of the calcaneus does not make complete contact with the ground on weight bearing. Alternative compensatory motion, extrinsic to the STJ, is necessary to achieve weight bearing on the medial aspect of the foot. One common compensation is an acquired soft tissue (valgus) deformity of the forefoot caused by a plantar flexed first ray. Other sources of compensatory motion can occur at the MTJ or proximally at the knee, hip, or sacroiliac joints.
An equinus deformity occurs when fewer than 10 degrees of ankle dorsiflexion are available as a result of osseous or muscular problems.51,53,56,57 Clubfoot (talipes equinovarus) is a congenital osseous deformity that includes varus deformity of both rearfoot and forefoot, rearfoot equinus, and an inverted and adducted forefoot.58,59 The angular relation between the body and the head and neck of the talus is decreased, and the navicular is shifted medially. Muscular forms of equinus include congenital or acquired soft tissue shortening or muscle spasm.53 Tissue contracture or shortening occurs in both contractile tissues (gastrocnemius, soleus, and plantaris) and noncontractile tissues (teno-Achilles and plantar fascia).51,53
Compensation for an equinus deformity occurs at the foot through pronation, perpetuating soft tissue contractures. The STJ is forced to pronate maximally to gain as much sagittal plane dorsiflexion as possible. Although foot pronation allows some dorsiflexion from the STJ, the amount is often inadequate. Pronation of the STJ unlocks the MTJ, creating an unstable midfoot while allowing further dorsiflexion and forefoot abduction from the oblique axis of the MTJ.53 Other compensatory strategies for equinus deformity include knee flexion (especially in individuals with cerebral palsy), early heel rise, toe walking, shortened stride length of the contralateral lower limb, and toe-out walking.31,41,53 Clinical consequences of long-term ankle equinus include many conditions normally associated with the excessively pronated foot: plantar fasciitis, heel spurs, bunions, and capsulitis.53
Examination of the Forefoot
Forefoot position is assessed with the STJ in neutral position. Because the first and fifth rays have independent axes of motion, forefoot orientation is defined by the planar relation of the second, third, and fourth rays to the bisection line of the calcaneus.
Neutral Forefoot Position
If the forefoot is properly balanced, the plane of the three central metatarsals is perpendicular to the bisection of the calcaneus when in STN (Figure 8-10). In a forefoot varus deformity, the forefoot is excessively supinated or inverted, whereas in a forefoot valgus the forefoot is excessively pronated or everted.
Mobility Testing: Locking Mechanism
To isolate STN position in the non–weight-bearing examination, the examiner attempts to lock the MTJ by applying a dorsally directed loading pressure with the thumb and index fingers over the fourth and fifth metatarsal heads of the patient’s foot (see Figures 8-6 and 8-7). Loading force must be gently applied over the fourth and fifth metatarsal heads until tissue slack is taken up from the normally plantar flexed resting position of the ankle.4,55 Overload of the forefoot leads to dorsiflexion and abduction of the foot, placing the forefoot in an excessively pronated position and giving a false valgus orientation.
Although many forefoot measurement devices are available, forefoot orientation can be accurately assessed with a standard goniometer.60 To assess the forefoot to rearfoot relation, the proximal arm of the goniometer is aligned along the bisection of the calcaneus, with the axis just below its distal border. The distal arm is positioned in the plane of the three central metatarsal heads (Figure 8-11). The angular displacement is recorded on the evaluation form under STN position for the forefoot assessment (see Figure 8-5).
In normal walking, the MTJ locks as heel rise begins so that the foot is converted into a rigid lever for propulsion. This lock requires that the STJ be in the neutral position. Clinical assessment of MTJ mobility and the locking mechanism is an advanced manual skill. Observations made during weight-bearing assessment (e.g., toe sign, navicular drop test, talar bulge) provide an elementary method to identify an MTJ unable to lock.
An ineffective locking mechanism at the MTJ is often more clinically significant than the absolute degree of forefoot deformity. For example, a forefoot varus deformity of 3 degrees with a poor MTJ locking mechanism may be symptomatic, whereas an 8-degree forefoot varus deformity with a normal MTJ locking mechanism may not be.
Identifying Forefoot Deformities
Although the prevalence of forefoot deformity, with or without symptoms, is well documented, less agreement exists regarding which types of deformity are most common.47,61 If an individual has bilateral forefoot deformity, the deformities may not be of the same severity or type. MTJ deformities change the location of the lock of the forefoot against the rearfoot.4 Although these osseous frontal plane deformities alter the direction of motion, they do not limit the total ROM of the MTJ.4 In forefoot varus, locking of the forefoot occurs in an inverted position relative to the rearfoot.4 Forefoot varus results from ontogenetic failure of the normal valgus rotation of the head and neck of the talus in relation to its body during early childhood development.4,45,55
Compensations for foot deformities are viewed in a relaxed weight-bearing position referred to as relaxed calcaneal stance (RCS). When excessive pronation of the STJ compensates for the deformity on weight bearing, the condition is called a compensated forefoot varus (Figure 8-12A). When the STJ cannot adequately pronate to accommodate an inverted forefoot, an uncompensated forefoot varus is present. The medial forefoot does not make contact with the ground, and the lateral forefoot is subjected to excessive pressure. Thick callus develops beneath the head of the fifth metatarsal, and the risk of stress fracture is increased. Plantarflex of the first ray and pronation at the MTJ are common compensations that allow the medial forefoot to make contact with the ground (see Figure 8-12B). Persistent MTJ stress may lead to joint damage and excessive forefoot abduction and eversion.
Forefoot valgus occurs in the frontal plane deformity, locking the forefoot in eversion relative to the rearfoot.4 Root suggests that this deformity results from ontogenetic overrotation of the talar head and neck in relation to its body during early childhood development.4,45,55 Forefoot valgus can be a rigid or flexible deformity. In rigid forefoot valgus, the compensatory weight-bearing mechanism occurs at the STJ as excessive supination or calcaneal inversion. It is a result of excessive premature GRFs at the first metatarsal head, causing rapid STJ inversion and increasing loading forces beneath the fifth metatarsal head. Thick callosities are often present beneath the first and fifth metatarsal heads. In contrast, flexible forefoot valgus is usually an acquired soft tissue condition. It most often occurs as a consequence of uncompensated rearfoot varus as an attempt to increase weight bearing along the medial foot. Because this deformity is flexible, no compensatory mechanism is necessary. Contact force beneath the first metatarsal head simply pushes it up out of the way, and the foot functions as if this condition were not present.
The First Ray
Assessment of first ray position is also carried out in STN position. Ideally, the first ray lies within the common transverse plane of the lesser metatarsal heads. To examine mobility of the first ray, the examiner holds the first metatarsal head between the thumb and index finger and performs a dorsal and plantar glide while stabilizing the lesser metatarsal heads with the other hand. Normally, first ray movement is at least one thumb width above and below the plane of the other metatarsal heads.4
As stance phase is completed, activity of the peroneus longus creates a pronatory twist of the forefoot, stabilizing the medial column of the foot on the ground, locking the MTJ about its longitudinal axis, and converting the foot to a rigid lever for propulsion. Adequate plantarflex of the first ray must be present for conversion from flexible forefoot to a rigid lever. Three factors determine how much first ray plantarflex must occur: amount of inversion of the foot at propulsion, width of the foot, and length of the second metatarsal.4 The more the foot inverts during propulsion, the further the first ray must plantarflex to make ground contact. Elevation of the medial forefoot is related to foot width; wide feet require more first ray plantarflex. An excessively long second metatarsal also increases the distance that the first ray must plantarflex to make ground contact.
In some instances, the first ray is inappropriately dorsiflexed above the plane of the other metatarsals, resulting in restriction of plantarflex and impeding normal propulsion. The first ray may also be plantar flexed below the plane of the other metatarsals. In uncompensated rearfoot varus, for example, the eversion motion of the calcaneus is insufficient, the medial condyle fails to make contact with the ground, and excessive weight bearing is present on the lateral border of the foot. The peroneus longus contracts to pull the first metatarsal head toward the ground in an attempt to load the medial side of the foot. This action is possible because of the cuboid pulley system (Figure 8-13).4 When the STJ remains abnormally pronated in late stance phase, orientation of the cuboid tunnel is altered and the mechanical advantage of the peroneus longus is lost. The MTJ cannot lock, and the foot is unstable throughout propulsion.
The presence of a rigid plantar flexed first ray sometimes results in a functional forefoot valgus (Figure 8-14). The compensatory mechanism for this condition is similar to that for rigid forefoot valgus: STJ supination or calcaneal inversion on weight bearing to lower the lateral aspect of the foot to the ground.
The Hallux
In normal gait, dorsiflexion of the hallux occurs during the late propulsive phase as the body moves forward over the foot. Sagittal plane motion of the hallux is assessed as passive ROM. The stationary arm of the goniometer is positioned along the medial first metatarsal and the mobile arm along the medial proximal phalanx of the hallux. The axis is medial to the first MTP joint.54 Sufficient force is applied to bring the hallux to its end ROM. Normal range of hallux dorsiflexion is between 70 and 90 degrees.4,54
In hallux limitus deformity, pathomechanical functioning of the first MTP joint prevents the hallux from moving through its full range of dorsiflexion during propulsion. Repetitive trauma to the first MTP joint can lead to ankylosis, or hallux rigidus. Functional hallux limitus is a condition in which full first MTP ROM is present when non–weight bearing, but a functional restriction of hallux dorsiflexion occurs during gait. Functional hallux limitus disrupts the normal windlass mechanism previously described.62,63
Limitation of hallux dorsiflexion prohibits the normal progression of the foot and interferes with propulsion of the body over the hallux. Several gait compensations can overcome this limitation.62,63 An abducted or toe-out gait pattern shifts propulsion to the medial border of the hallux. A pinched callus then develops from friction between the hallux and shoe during propulsion. Alternatively, the IP joint of the hallux may hyperextend, causing a callus in the sulcus of the IP joint.
Hallux abductovalgus (HAV) is a progressive, acquired deformity of the first MTP joint that eventually results in a valgus subluxation of the hallux.4,45 This deformity is caused by abnormal STJ pronation with hypermobility of the first ray.4 A common misconception is that HAV is hereditary. Although the congenital osseous abnormalities that lead to aberrant STJ pronation are hereditary, HAV occurs to compensate for these other deformities. Another misconception is that HAV is caused by restrictive footwear. Although inappropriate or restrictive footwear can accentuate or speed the progression of HAV deformity when present, the deformity is frequently observed in populations that do not typically wear shoes.4,45
Additional Observations
Several other important observations are made as the non–weight-bearing examination is completed. Non–weight-bearing arch height is observed for later comparison to weight-bearing arch height as a composite estimate of foot pronation. Toes are inspected for positional deformities such as hammertoe, claw toe, crossover deformity, and the presence of bunions or bunionettes. The plantar foot is checked for callus, plantar warts, or other signs of excessive pressure. The shoes are inspected for excessive or uneven wear patterns.
Static weight-bearing closed kinetic chain examination
The open chain kinetic motion evaluated in the non–weight-bearing examination is dramatically different from the functional sequence of events in the closed kinetic chain of standing and walking. Open kinetic chain pronation (calcaneal dorsiflexion, eversion, and abduction) and supination (calcaneal plantarflex, inversion, and adduction) are triplanar motions around the STJ.4,13,29 During closed kinetic chain pronation, internal rotation of the leg is coupled with talar adduction and calcaneal plantarflex and eversion. Closed kinetic chain supination couples external rotation of the leg with talar abduction and calcaneal dorsiflexion and inversion. In the open kinetic chain, movement is initiated in the distal segment (the foot). In the closed kinetic chain, motion is initiated proximally (at the tibia and talus). A thorough closed kinetic chain examination includes static postural observations, dynamic motion testing, and gait assessment.
Compensatory mechanisms that result from intrinsic deformities are assessed as the foot is subjected to GRFs during the static weight-bearing examination. This provides valuable insight regarding how the body compensates for the intrinsic foot deformities or impairments of normal foot joint function identified in the non–weight-bearing examination. Improper foot functioning can lead to a complex series of compensations that influence the mobility patterns of the foot and lower leg, as well as the knee, hip, pelvis, and spine.
The patient stands in a relaxed, weight-bearing posture (RCS). The examiner observes the patient’s preferred stance, noting postural alignment and foot placement angle. The patient then adjusts the stance position, if necessary, to assume equal weight-bearing double-limb support, with feet 5 to 10 cm apart and oriented in neutral toe-in and toe-out foot placement angle. This adjusted posture, with neutral foot placement angle, offers a better frame of reference for assessing planar alignment and enhances the reliability and consistency of the measurement.41 Postural alignment or body symmetry of the patient is evaluated in the frontal, sagittal, and transverse planes.
Frontal Plane
Static weight-bearing examination in the frontal plane focuses on the angular relation of the calcaneus and the tibia and fibula with respect to the floor and the relation between the pelvis and the lower leg.
Calcaneal Alignment to the Floor
With the patient in double-limb stance posture, a line bisecting the posterior surface of the calcaneus is visualized and the angular relation between the line and the floor taken. Because the infracalcaneal fat pad often migrates (related to prolonged weight bearing), care must be taken to avoid errors in visual assessment (Figure 8-15). Palpation of the osseous medial, lateral, and inferior borders of the calcaneus helps factor out fat pad migration and improve measurement accuracy. Calcaneal alignment can also be quantified with a protractor to measure the degree of calcaneal tilt relative to vertical.64
The key question to answer is whether the calcaneus is inverted, vertical, or everted relative to the floor during stance; the actual angular degree is not as important as the relative orientation of the calcaneus. This component of the examination assesses the ability of the STJ to provide enough pronation to compensate for its neutral position. In the normal closed chain STN position, the calcaneus is in 1 to 4 degrees of varus (inversion). The STJ must have an equal amount of compensatory pronation to lower the medial condyle to the ground for a vertical calcaneus. If a patient has uncompensated rearfoot varus of 10 degrees in STN as well as restricted calcaneal motion (−4 degrees) eversion, the STJ would not be able to achieve sufficient pronation or eversion in stance for normal calcaneal alignment. Instead, the calcaneus would be in an inverted alignment relative to the floor. Inverted calcaneal position also occurs when a rigid forefoot valgus or rigid plantarflexed first ray deformity is present. STJ supination is a compensatory mechanism for both deformities.
In contrast, forefoot varus deformity requires excessive compensatory STJ pronation; calcaneal eversion occurs in weight bearing. The position of the calcaneus with respect to the floor provides insight into the type of STJ compensation present and can be correlated with the biomechanical findings of the non–weight-bearing examination. If the STJ is unable to pronate enough to compensate completely for a deformity, additional pronatory motion occurs at the MTJ or by eversion tilting of the talus within the ankle mortise.4,41,48 The functional rearfoot unit (calcaneus and talus) may assume a valgus (everted) position relative to the floor, even if calcaneal eversion is restricted.
Tibiofibular Alignment
Proximal structural malalignments, such as tibial varum or valgum, contribute to abnormal foot pronation and overuse injuries. In osseous congenital tibial varum, the distal third of the tibia is angled medially in the frontal plane, whereas in tibial valgum, the distal tibia inclines away from the midline.41
Tibial alignment can be measured with either a standard goniometer (Figure 8-16) or a bubble inclinometer; both assess the angular relation between the bisection of the distal third of the lower leg relative to the supporting surface.41,65 Radiographic measurement of lower leg position is better correlated with clinically assessed tibiofibular position values than with isolated tibial position. Radiographic measurement may be the most accurate method to isolate true tibial varum.66
Test position is critical because variation in STJ alignment greatly influences tibiofibular varum measurement values. Tibiofibular varum values are larger in RCS than in the STN position because of the combined effects of osseous malalignment and varus leg alignment associated with compensatory STJ pronation in stance.65–67 The incidence of tibiofibular varum appears to be high, although no clear normative values have been established.
The alignment of the distal third of the leg relative to the floor more accurately represents tibiofibular position than tibial position. Values assessed in STN reflect neutral tibiofibular alignment, whereas values assessed in RCS represent compensatory tibiofibular repositioning in response to STJ and MTJ pronation. High tibiofibular varum values measured in STN elevate the medial foot from the supporting surface, requiring excessive compensatory foot pronation during gait. High tibiofibular varum values in RCS suggest excessive foot pronation, although the source of that pronation cannot be isolated.
Alignment of the Pelvis and Lower Leg
The final component of the frontal plane assessment evaluates symmetry of the anterosuperior iliac spines, iliac crests, greater trochanters, gluteal folds, popliteal creases, genu varum or valgum deformities, fibular heads, patellae, and malleolar levels. Asymmetry often indicates sacroiliac joint dysfunction or leg length discrepancy, influencing foot position and function in the closed kinetic chain.
Sagittal Plane
The second component of the weight-bearing examination considers function in the sagittal plane. The examiner looks for evidence of genu recurvatum or excessive knee flexion, navicular drop, talar bulge, and inadequate or excessive height of the longitudinal arch.
Knee Position
Viewing the patient’s stance from the side, the examiner observes the verticality of the tibia. In genu recurvatum, the proximal tibia is aligned behind the axis of the TCJ, resulting in hyperextension of the knee and plantarflex of the TCJ with relative shortening of the limb. Genu recurvatum also occurs as a compensation for equinus deformity at the ankle. When true leg-length discrepancy is present, two types of compensation are possible. If limb length difference is small, genu recurvatum may adequately shorten the longer limb. For larger limb length differences, knee flexion of the longer limb is often used to minimize asymmetry.
Navicular Drop
The position of the navicular is examined by using the navicular drop test, a composite measure of foot pronation focusing on displacement of the navicular tuberosity as a patient moves from closed kinetic chain STN to RCS position.68 Excessive navicular displacement is associated with collapse of the MLA and may be correlated with midfoot pain or other symptoms of excessive foot pronation.41 Subotnick69 established an interdependency between MTJ and STJ function based on articulation of the navicular and cuboid with the talus and calcaneus. Brody68 suggested that the navicular drop test is a valid assessment of STJ function in the closed kinetic chain. Although navicular drop occurs with STJ pronation that stems from intrinsic foot deformity, it can also be the result of muscle insufficiency or ligamentous laxity.41
To measure navicular drop, an index card is held perpendicular to the medial foot, the level of the navicular tuberosity is marked in STN position and RCS, and the distance between the marks is calculated.38 Normative studies report a mean navicular drop of between 7.3 and 9 mm.39,70 A navicular drop of more than 10 mm is considered abnormal.70
Talar Bulge and Arch Height
When excessive STJ pronation is present in stance, the talus moves into adduction and plantarflex. Displacement of the talar head causes an observable medial bulge in the region of the talonavicular joint.71 The height of the MLA normally decreases moderately in weight bearing as a result of normal STJ pronation. Pes planus deformity (flatfootedness) is characterized by excessive collapse of the MLA. In hereditary rigid flatfoot, the MLA is low or absent in non–weight-bearing and weight-bearing positions. In flexible flatfoot, the height of the MLA is normal in non–weight bearing but drops excessively in weight bearing because of abnormal STJ pronation. In normal foot alignment, the medial malleolus, navicular tuberosity, and first metatarsal head fall along the Feiss line.71 In a severely pronated foot, the navicular tuberosity lies below the Feiss line. In extreme cases the tuberosity may even rest on the floor.71,72
Transverse Plane
The final component of the static weight-bearing examination considers foot function in the transverse plane. The examiner looks for signs of excessive pronation or forefoot adduction and torsional deformities of the lower extremities.
Toe Sign
A positive toe sign indicates excessive pronation or abduction of the foot in the transverse plane (Figure 8-17). The sign is determined by the number of toes that can be seen, in a posterior view, when the patient is standing in RCS with a neutral foot placement angle.63 Normally no more than 1.5 toes are visible beyond the lateral border of the foot. If more toes can be seen, abnormal pronation may be present, causing excessive transverse plane motion or abduction of the foot. A false-positive toe sign can occur in the presence of a relative toe-out foot placement angle associated with lateral rotational deformities (e.g., femoral retroversion) or muscle imbalances that limit internal rotation of the hip (e.g., tight piriformis). Ensuring that the patient’s patellae are oriented in the frontal plane before assessing toe sign reduces the risk of false-positive findings.
< div class='tao-gold-member'>
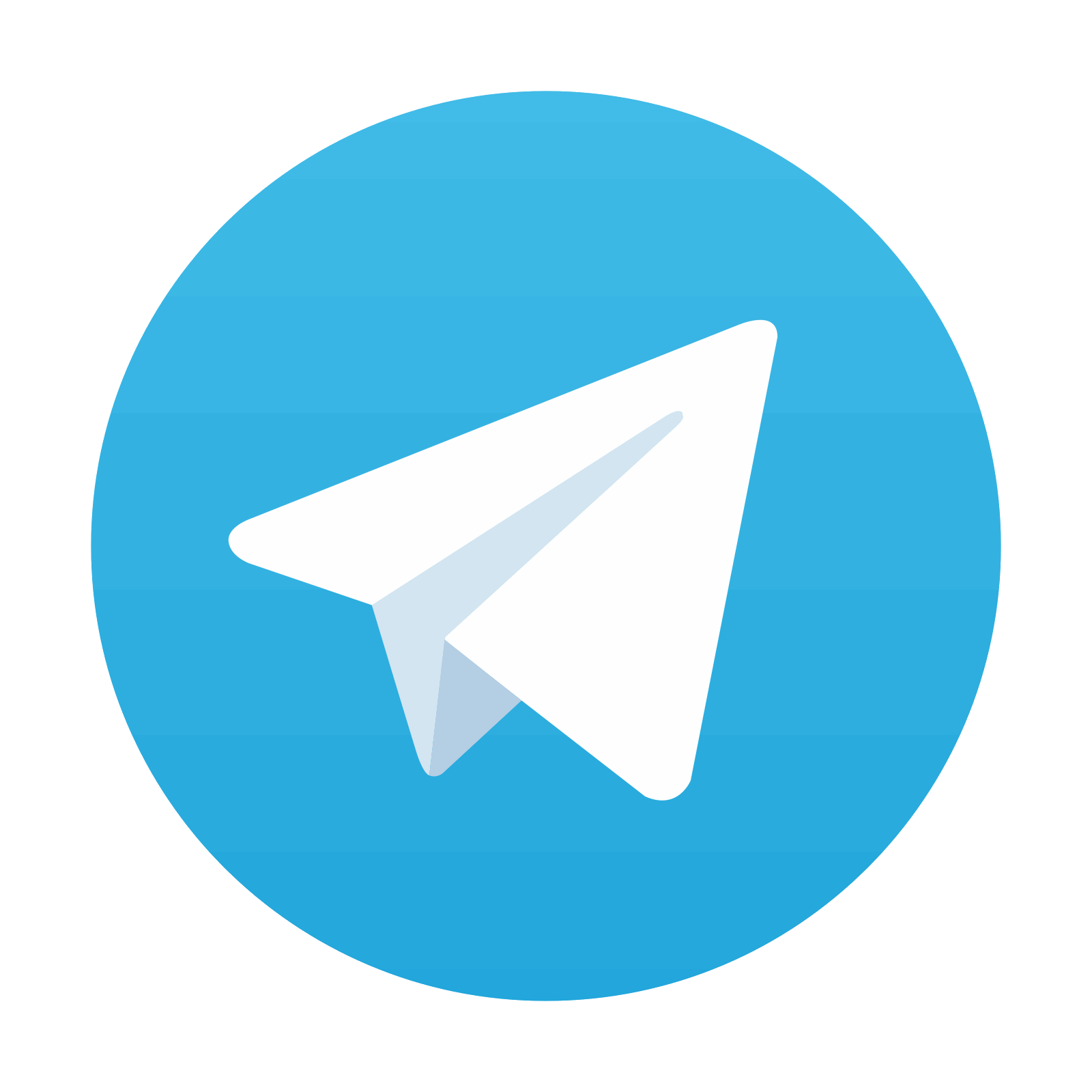
Stay updated, free articles. Join our Telegram channel

Full access? Get Clinical Tree
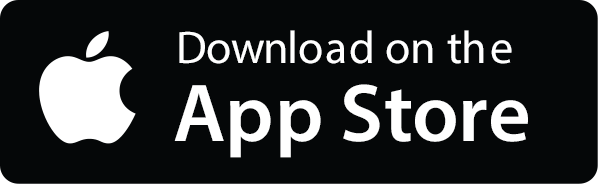
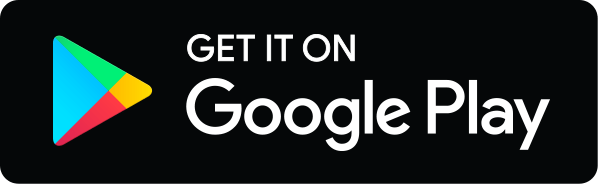