Orthotic Decision Making in Neurological and Neuromuscular Disease
Michelle M. Lusardi and Donna M. Bowers
Learning objectives
On completion of this chapter, the reader will be able to:
Movement impairment in neurological and neurneuromuscular pathology
Pathologies of the neuromuscular system manifest in a sometimes confusing array of clinical signs and symptoms. To select the most appropriate therapeutic intervention, be it exercise to promote neuroplasticity and recovery, functional training, or the use of various orthoses and assistive devices to accommodate for impairment of a body system or structure, the clinician must develop a strategy to “classify” the movement disorder that has produced the observed impairments and functional limitations.1 The clinician must understand the medical prognosis and potential progression of the disease process, as well as the lifestyles and risk factors that might contribute to secondary impairments that limit function over time (even if the disease is “nonprogressive”) and their impact on the individual’s growth and development.1–3
Health professionals use a number of organizational strategies as frameworks for decision making during rehabilitation of individuals with pathologies leading to neuromuscular dysfunction. Many neurologists use a medical differential diagnosis process to locate the lesion as being within the central nervous system (CNS) or involving structures of the peripheral nervous system (PNS) or the muscle itself.4 They do this by triangulating evidence gathered by examining tone and deep tendon reflexes, observing patterns of movement and postural control, and looking for specific types of involuntary movement.5,6 They may also interpret results of special tests such as nerve conduction studies, electromyography (EMG), computed tomography (CT), and magnetic resonance imaging (MRI).7,8 These tests might pinpoint areas of denervation, ischemia, or demyelinization and help the health professionals arrive at a medical diagnosis.
Rehabilitation professionals are most interested in the functional consequences associated with the various neuromotor conditions. They examine the ways in which abnormal tone (hypertonicity—excessive tone; hypotonicity—insufficient tone; or flaccidity—absence of tone) affects mobility and locomotion, postural control, motor planning and motor control during movement, coordination (error control), and muscle performance during functional activities.9,10 Rehabilitation professionals are not only concerned about function at the present time but also consider the long-term impact of neuromotor impairment on the person’s joints and posture, especially in children who are growing with abnormal tone and postures.11,12
This chapter considers the ways that key components of the CNS and PNS contribute to functional movement. We explore the concepts of muscle tone and muscle performance, considering how their interaction influences an individual’s ability to move. We investigate how abnormalities of tone resulting from CNS and PNS pathologies are described in clinical practice. We consider the determinants of postural control and of coordination and how CNS and PNS pathologies might lead to impairments of balance and movement. We provide an overview of the ways that commonly encountered CNS pathologies impact muscle tone, muscle performance, postural control, movement, and coordination as a way of understanding how an orthosis or adaptive equipment might help improve an individual’s ability to walk and participate in meaningful activities and roles. We consider how the physical therapy examination contributes to the determination of a need for an orthosis or adaptive equipment. We also explore the clinical decision-making process by asking key questions about how an orthosis might help (or hinder) function. Finally, we apply what we have learned using five clinical cases to develop orthotic prescriptions.
Differential Diagnosis: Where Is the Problem?
Most neurological and neuromuscular diseases affect either the CNS or the PNS; only a few diseases, such as amyotrophic lateral sclerosis, affect both CNS and PNS. Diseases of the CNS and of the PNS may contribute to motor or sensory impairment; however, there are patterns and characteristics of dysfunction that are unique to each. Selection of the appropriate orthosis, seating/wheelchair system, or assistive devices is facilitated when the therapist, orthotist, members of the rehabilitation team, patient, and patient’s family understand the normal function and consequences of the disease process of the neurological subsystem that is affected.
The Central Nervous System
The CNS is a complex of dynamic and interactive subsystems that mediates purposeful movement and postural control, vital autonomic vegetative and physiological functions, and learning of all types.13 Readers are encouraged to refer to a recent neuroanatomy or neuropathology textbook to refresh their understanding of CNS structure and function. Knowledge of the roles of various CSN structures and their interactions (for perception, problem solving, motor planning, and coordination) is foundational for clinical decision making when considering orthoses for individuals with neuromuscular dysfunction.
Some diseases affect a single CNS system or center (e.g., Parkinson’s disease affects the function of the basal ganglia in regulating agonist/antagonist muscle activity; a lacunar stroke in the internal capsule may interrupt transmission only within the pyramidal/corticospinal pathway) leading to a specific array of signs/symptoms characteristic of that system or center. Other pathologies disrupt function across several systems: a thromboembolic stroke in the proximal left middle cerebral artery may disrupt volitional movement and sensation of the right side of the body, as well as communication and vision. Several exacerbations of multiple sclerosis (MS) may lead to plaque formation in the cerebral peduncles/pyramidal system, superior cerebellar peduncle/error control system, restiform body/balance system, and fasciculus gracilis/lower extremity sensation; in this case, it can be challenging but imperative to sort through the various types of impairments that may result in order to select the most appropriate therapeutic or orthotic intervention for the individual.
Pyramidal System
The pyramidal system is responsible for initiation of volitional movement and plays a major role in the development of skilled and manipulative activities. The cell bodies of pyramidal neurons are located in the postcentral gyrus/primary motor cortex. The motor cortex in the left cerebral hemisphere influences primarily the right side of the body (face, trunk, and extremities); the right cortex influences the left body. The axons of pyramidal neurons form the corticobulbar and corticospinal tracts, projecting toward α motor neurons in cranial nerve nuclei and anterior horn of the spinal cord. To reach their destination, these axons descend through the genu and posterior limb of the internal capsule, the cerebral peduncles, the basilar pons, the pyramids of the medulla, and finally the opposite lateral funiculus of the spinal cord. A lesion at any point in the pyramidal system has the potential to disrupt volitional movement. The degree of disruption varies with the extent and functional salience of the structures that are damaged, manifest on a continuum from mild weakness (paresis) to the inability to voluntarily initiate and direct movement (paralysis).
Immediately following insult or injury of the pyramidal system, during a period of neurogenic shock, there may be substantially diminished muscle tone and sluggish or absent deep tendon reflexes.14 As inflammation from the initial insult subsides, severely damaged neurons degenerate and are resorbed, while minimally damaged neurons may repair themselves and resume function.15,16 The more neurons that are destroyed, the greater the likelihood that hypertonicity will develop over time due to the altered balance of descending input of pyramidal and extrapyramidal systems. As the recovery period continues, individuals may begin to move in abnormal synergy patterns whenever volitional movement is attempted.17,18 When the damage to the system is less extensive, individuals may eventually recover some or all volitional motor control; the more extensive the damage to the system, the more likely there will be residual motor impairment.15,18
Extrapyramidal System
The extrapyramidal system is made up of several subcortical subsystems that influence muscle tone, organize patterns of movement from among the many possible movement strategies, and make both feedforward adjustments (in anticipation of movement) and refining feedback adjustments (in response to sensations generated as movement occurs) during performance of functional tasks.19 The motor planning subsystem is a series of neural loops interconnecting the premotor and accessory motor cortices in the frontal lobes, the nuclei of the functional basal ganglia (caudate, putamen, globus pallidus, substantia nigra, subthalamus), and several nuclei of the thalamus. Damage to the premotor and accessory motor cortex leads to apraxia, the inability to effectively sequence components of a functional task and to understand the nature of a task and the way to use a tool in performance of the task.20 If there is damage to the caudate and putamen (also called the corpus striatum), underlying muscle tone may fluctuate unpredictably (athetosis) and involuntary dancelike movements (chorea) are likely to occur.21 Damage to the subthalamic nuclei can lead to forceful, often disruptive, involuntary movement of the extremities (ballism) that interrupts purposeful activity.22 Damage to the substantia nigra characteristically leads to resting tremor, rigidity of axial and appendicular musculature (hypertonicity in all directions), and bradykinesia (difficulty initiating movement, slow movement with limited excursion during functional tasks), which are most commonly seen in persons with Parkinson’s disease.23 Motor impairments resulting from damage to the ventral anterior nucleus and related thalamic nuclei are less well understood but may contribute to less efficient motor planning, especially when the individual is learning or performing novel tasks.24
Extrapyramidal structures influence muscle tone and readiness to move via a network of interconnections among motor centers in the brainstem. The reticulospinal tracts, originating in the lower pons and medulla, are thought to influence tone by acting on γ motor neurons and their associated muscle spindles. They play a major role in balancing the stiffness required for antigravity position and the flexibility necessary for movement of the limbs through space during functional activity and are likely the effectors for tonic hindbrain reflexes.19,25 The vestibulospinal tracts, also originating from the pons and medulla, influence anticipatory postural adjustment in preparation for movement and reactionary postural adjustments as movement occurs. These tracts are thought to be the “effectors” for postural control and balance.19,26 The tectospinal tracts, originating in the collicular nuclei of the dorsal midbrain, influence linkages between the head and extremities (especially arms and hands) so that the visual and auditory systems can be used effectively to orient the head and body during tasks that require visual (eye-hand) and auditory (ear-hand) guidance.16,27
Coordination Systems
The error control, or coordination subsystem, has several interactive components.28 Feedforward information (how movement is likely to occur) from the forebrain’s motor cortex is relayed through the thalamus to the deep nuclei of the cerebellum via the middle cerebellar peduncle (brachium pontis). Feedback information generated by movement travels from the muscle spindle and anterior horn of the spinal cord via the inferior cerebellar peduncle (restiform body), as does sensory information from static and dynamic vestibular receptors (head position and movement in space) and the vestibular nuclei in the brainstem. Through interaction of Purkinje cells in the cerebellar cortex and neurons in the deep cerebellar nuclei, the cerebellum judges how “in sync” these various types of information are (essentially asking the questions, “Did the movement occur as planned? Was the outcome of the movement as intended?”) and suggests refinements for more precise and coordinated movement.29 These adjustments are relayed to the red nucleus in the midbrain via the superior cerebellar peduncle (brachium conjunctiva) and are forwarded back to the thalamus and the motor cortex, as well as to the spinal cord via the rubrospinal tract. The rubrospinal tract is thought to be essential for refinement and correction of direction and control of movement as it occurs.29
Somatosensory and Perceptual Systems
The somatosensory system is composed of a set of ascending pathways, each carrying a specific sensory modality from the spinal cord and brainstem to the thalamus and postcentral gyrus of the cerebral cortex, reticular formation, or cerebellum. The anterolateral (spinothalamic) system carries exteroceptive information from mechanoreceptors that monitor protective senses (e.g., pain, temperature, irritation to skin and soft tissue).30 This tract originates in the dorsal horn (substantia gelatinosa) of the spinal cord and the spinal trigeminal nucleus, crosses the midline of the neuraxis to ascend in the lateral funiculus of the spinal cord to the contralateral ventral posterior thalamus, and then continues to the postcentral gyrus. The dorsal column/medial lemniscus carries information from encapsulated receptors that serve as internal monitors of body condition and motion.31 This tract ascends from the spinal cord to reach the nuclei gracilis and cuneatus in the medulla of the brainstem, then crosses midline to ascend to the contralateral ventral posterior thalamus and on to the posterior central gyrus. The postcentral gyrus (somatosensory cortex) is organized as a homunculus, with each region of the body represented in a specific area.32 Sensation from the lower extremities (lumbosacral spinal cord) is located at the top of the gyrus near the sagittal fissure. Moving downward toward the lateral fissure, the next area represented is the trunk (thoracic spinal cord), followed by upper extremities and head (cervical spinal cord), and finally face, mouth, and esophagus (trigeminal nuclei) just above the lateral fissure. A lesion such as an MS plaque in one of the ascending pathways may result in a discrete area of loss of exteroception or of conscious proprioception in one area of the body; a lesion on the somatosensory cortex can lead to a more profound, multimodality impairment on the opposite side of the body.
Although sensory information is logged in at the postcentral gyrus, the location of the somatosensory cortex, interpretation and integration of this information occurs in the somatoperceptual system in the parietal association areas, with specialization in the right hemisphere.32 These association areas give meaning to the sensations that are generated as people move and function in their environments. This is where people understand the relationships among their various extremities and trunk (body schema), as well as their relationship to and position within our physical environment. Damage to the parietal lobes leads to problems ranging from left-right confusion to the inability to recognize and monitor the condition of a body part (neglect, agnosia), depending on how much of the association area is involved.33,34
Visual and Visual-Perceptual Systems
The visual system begins with processing of information gathered by the rods and cones in the multiple layers of specialized neurons in the retina, located in the posterior chamber of the eye. Axons from retinal ganglion cells are gathered into the optic nerve, which carries information from that eye toward the brain. At the optic chiasm there is reorganization of visual information, such that all information from the left visual field (from both eyes) continues in the right optic tract and that from the right field continues in the left tract. This information is relayed, through the lateral geniculate body of the thalamus, via the optic radiations, to the primary visual cortex on either side of the calcarine fissure of the midsagittal occipital lobe.35 Damage to the retina or optic nerve results in loss of vision from that eye. Damage to the optic chiasm typically leads to a narrowing of the peripheral visual field (bitemporal hemianopsia); a lesion of one of the optic tracts or radiations leads to loss of part or all of the opposite visual field (homonymous hemianopsia). Damage to the visual cortex can result in cortical blindness, in which visual reflexes may be intact but vision is impaired.36
Visual information is interpreted in the visual association areas in the remainder of the occipital lobe.37 The visual association areas in the left hemisphere are particularly important to interpretation of symbolic and communication information, while spatial relationships are of more interest in the right hemisphere. Specific details about the environment, especially about speed and direction of moving objects with respect to the self and of the individual with respect to a relatively stationary environment, are important contributors to functional movement and to the development of skilled abilities.38 Interconnections between the parietal and occipital association areas serve to integrate visual and somatic/kinesthetic perception and provide important input to motor planning and motor learning systems.39
Effective visual information processing is founded on three interactive dimensions: visual spatial orientation, visual analysis skills, and visual motor skills.40 Developmentally, visual spatial orientation includes spatial concepts used to understand the environment, the body, and the interaction between the body and environment that are part of functional activity (e.g., determining location or direction with respect to self, as well as respect to other objects or persons encountered as people act in their environment). Visual analysis skills allow people to discriminate and analyze visually presented information, identify and focus on key characteristics or features of what people see, use mental imagery and visual recall, and respond or perceive a whole when presented with representative parts. The visual motor system links what is seen to how the eyes, head, and body move, allowing one to use visual information processing skills during skilled, purposeful activities. This also provides the foundation for fine manipulative skills requiring eye-hand coordination.37
Executive Function and Motivation
The ability to problem solve, consider alternatives, plan and organize, understand conceptual relationships, multitask, set priorities, and delay gratification, as well as the initial components of learning are functions of the frontal association areas of the forebrain.41,42 These dimensions of cognitive function are often described by the phrase higher executive function. Quantitative and other analytical skills are thought to be primarily housed in the frontal association areas of the left hemisphere, while intuitive understanding and creativity may be more concentrated in the right hemisphere. Most people tap the resources available in both hemispheres (via interconnections through the corpus callosum) during daily life, although some may fall toward one end or another of the analytical-intuitive continuum. Individuals with acquired brain injury involving frontal lobes often exhibit subtle deficits that have a significant impact on their ability to function in complex environments, as well as under conditions of high task demand; difficulty in these areas certainly compromises functional efficiency and quality of life.43,44
The neuroanatomical structures that contribute to the motivational system include the nuclei and tracts of the limbic system, prefrontal cortex, and temporal lobes; all play major roles in managing emotions, concentration, learning, and memory.45 The motivational system not only has an important impact on emotional aspects of behavior but also influences autonomic/physiological function, efficacy of learning, interpretation of sensations, and preparation for movement (Figure 10-1).46 Dimensions of limbic function that influence motivation and the ability to manage challenges and frustration include body image, self-concept, and self-worth as related to social roles and expectations, as well as the perceived relevance or importance (based on reward or on threat) of an activity or situation.47,48 Central set is a phrase used to describe the limbic system’s role as a motivator and repository of memory on readiness to move or act.46 Central set helps people predict movement needs relevant to a given situation or circumstance (considering both the physical and affective dimensions of the environment in which they are acting) from past experience.49
Consciousness and Homeostasis
The ability to be alert and oriented when functioning in a complex environment is the purview of the consciousness system and is a function of interaction of the brainstem’s reticular formation, the filtering system of thalamic nuclei, and the thought and problem solving that occur in the association areas of the telencephalon, especially in the frontal lobes.50 The reticular activating system, found in the inferior mesencephalon and upper pons of the brainstem, is the locus of sleep-wake and level of alertness.51 The thalamus and the reticular formation help people habituate to repetitive sensory stimuli while they focus on the type of sensory information that is most germane to the task at hand.52 The frontal association areas add content to one’s consciousness: the ability to reason and to adapt to challenges encountered as one moves through daily life.53 Alteration in quality and level of consciousness and behavior are indicators of evolving problems within the CNS.54 Increasing intracranial pressure, the result of an expanding mass or inflammatory response following trauma or ischemia, may be initially manifest by confusion or agitation; progression into a state of lethargy, stupor, or unresponsiveness (coma) indicates deteriorating compromise of the CNS structures.55
Homeostasis and the ability to respond to physiological stressors are functions of the components of the autonomic nervous system.56 The nuclei of the hypothalamus serve as the command center for parasympathetic and sympathetic nervous system activity via projections to parasympathetic cranial nerve nuclei in the brainstem, the sympathetic centers of the intermediate horn in the thoracic spinal cord, and the parasympathetic centers in the lumbosacral spinal cord. The hypothalamus has extensive interconnections with the limbic system, bridging physiological and emotional/psychological aspects of behavior and activity.57 The hypothalamus also integrates neural-endocrine function through interconnections with the pituitary gland.58 Clearly, this relatively small area of forebrain plays a substantial integrative role in physiological function of the human body. Damage or dysfunction to this area therefore has significant impact on physiological stability and stress response.
Peripheral Nervous System
The PNS serves two primary functions: to collect information about the body and the environment and to activate muscles during functional activities. Afferent neurons collect data from the various sensory receptors distributed throughout the body and transport this information to the spinal cord and brainstem (sensory cranial nerves) for initial interpretation and distribution to CNS centers and structures that use sensory information in the performance of their various specialized roles.33 The interpretation process can have a direct impact on motor behavior at the spinal cord level (e.g., deep tendon reflex) or along any synapse point in the subsequent ascending pathway (e.g., righting and equilibrium responses) as sensory information is transported toward its final destination within the CNS.59 Efferent neurons (also described as lower motor neurons or, more specifically, α and γ motor neurons) carry signals from the pyramidal (voluntary motor) and extrapyramidal (supportive motor) systems to extrafusal/striated and intrafusal (within muscle spindle) muscle fibers that direct functional movement by enacting the CNS’s motor plan.26,60,61
The cell bodies of these α and γ motor neurons live in the anterior horn of the spinal cord and in cranial nerve somatic motor nuclei. In the spinal cord, α and γ axons project through the ventral root, are gathered into the motor component of a spinal nerve, and (in cervical and lumbosacral segments) are reorganized in a plexus before continuing toward the targeted muscle as part of a peripheral nerve. In the brainstem, α and γ axons project to target muscles via motor cranial nerves (oculomotor, III; trochlear, IV; motor trigeminal, V; abducens, VI; facial, VII; glossopharyngeal, IX; spinal accessory, XI; hypoglossal, XII).62 When α and γ axons reach their target set of muscle fibers (motor unit), a specialized synapse—the neuromuscular junction—triggers muscular contraction.63
Pathologies of the PNS can be classified by considering two factors: the modalities affected (only sensory, only motor, or a combination of both) and the anatomical location of the problem (at the level of the sensory receptor, along the neuron itself, in the dorsal root ganglion, in the anterior horn, at the neuromuscular junction, or in the muscle itself).64,65 Poliomyelitis is the classic example of an anterior horn cell disease; Guillain-Barré syndrome is a demyelinating infectious-autoimmune neuropathy that impairs transmission of electrical impulses over the length of motor and sensory nerves. The polyneuropathy of diabetes (affecting motor, sensory, and autonomic fibers) is the classic example of a metabolic neuropathy. Radiculopathies (e.g., sciatica) result from compression or irritation at the level of the nerve root, while entrapment syndromes (e.g., carpal tunnel) are examples of compression neuropathies over the more distal peripheral nerve. Myasthenia gravis, tetanus, and botulism alter function at the level of the neuromuscular junction. Myopathies and muscular dystrophies are examples of primary muscle diseases.65
Determinants of effective movement
Regardless of the underlying neurological or neuromuscular disease, rehabilitation professionals seek to understand the impact of the condition on an individual’s underlying muscle tone and motor control (ability to initiate, guide, sustain, and terminate movement), muscle performance (strength, power, endurance, speed, accuracy, fluidity), and postural control and balance (ability to stay upright, to anticipate how to make postural adjustment during movement, and to respond to unexpected perturbations) in order to move effectively during goal-directed, functional movement necessary for daily life.
Muscle Tone and Muscle Performance
Effectiveness of movement is determined by the interaction of underlying muscle tone and muscle performance. Muscle tone can be conceptualized as the interplay of compliance and stiffness of muscle, as influenced by the CNS. Ideally the CNS can set the neuromotor system to be stiff enough to align and support the body in functional antigravity positions (e.g., provide sufficient baseline postural tone) but compliant enough in the limbs and trunk to carry out smooth and coordinated functional movement and effectively respond to changing environmental conditions or demands as daily tasks are carried out.66,67 At the center of the tone continuum, the interplay of stiffness and compliance is optimal, such that motor performance is well supported (Figure 10-2, horizontal continuum). At the low-tone end of the continuum, where there is low stiffness and high compliance, individuals are challenged by inadequate postural control and inability to support antigravity movement. At the high tone end of the continuum, where there is high stiffness and low compliance, freedom and flexibility of movement are compromised. Among individuals, postural tone varies with level of consciousness, level of energy or fatigue, and perceived importance (salience) of the tasks they are involved with at the time.68,69
Effective movement occurs when muscle performance meets the demands of the task. The components of muscle performance are the ability to (1) generate sufficient force (strength); (2) at the rate of contraction required for the task at hand (speed and power); (3) to sustain the concentric, holding/isometric, or eccentric contraction necessary to meet task demands (muscle endurance); (4) to ramp up or dampen force production in response to task demands (accuracy); and (5) coordinate mobility and stability of body segments to complete the task (fluidity). Muscle performance can also be conceptualized as having a continuum with optimal control of its components around the center and inadequate control on either side: hypokinesis (little movement) at one extreme and hyperkinesis (excessive movement) at the other (see Figure 10-2, vertical continuum).
While muscle tone and muscle performance are distinct contributors to movement, they are certainly interactive.70,71 Movement is most effective and efficient if an individual’s resources fall at the center of each continuum. A problem with muscle tone, or with muscle performance, or a combination of both leads to abnormal and less efficient movement. Consider what will happen if there is a combination of low tone and inadequate hypokinetic muscle performance: individuals will have difficulty with force production and power, as well as with eccentric and isometric control, such that movement tends to occur in shortened ranges. For example, an infant with Down syndrome (with low tone) struggles to sustain an upright head in prone on elbows position in order to visually interact or reach for a toy (hypokinesis).
In the presence of low tone and hyperkinetic muscle performance, movement is fast but imprecise, with bursts of power that cannot be sustained. For example, a toddler with Down syndrome (with low tone) who is beginning to walk takes rapid and inconsistent steps (hyperkinesis). In contrast, the presence of high tone and hypokinetic muscle performance, movement is slow and stiff, with inadequate force production, compromised segmentation, impaired eccentric control (difficulty letting go), and constrained range. For example, an individual with Parkinson’s disease takes short steps and has little reciprocal arm swing when walking.
In the presence of high-tone and hyperkinetic muscle performance, there tends to be “all or nothing” force production, with somewhat ballistic and inaccurate movement occurring between the extremes of ranges. For example, a child with spastic quadriplegic cerebral palsy (CP) rising from sitting to standing often employs rapid mass extension (lower extremity and trunk), compromising his or her ability to move toward flexion in order to effectively rise; the child would also have difficulty lowering back into sitting without collapsing. Although muscle performance, especially the ability to generate force, does tend to decline with aging, the impact of inactivity is even more profound; all aspects of muscle performance can improve with appropriate training, even in the very old.72–74
Traditionally, an individual’s muscle tone has been described clinically as hypertonic or spastic, rigid, hypotonic or low, flaccid, or fluctuating.
Hypertonus
Hypertonus is a term used to describe muscles that are influenced to be too stiff or are excessively biased toward supporting antigravity function. Spasticity is a type of hypertonus that typically occurs when there is damage to one or more CNS structures of the pyramidal motor system and is encountered as a component of many neuromuscular pathologies.75–79 Decrements in underlying tone and muscle performance, in bipedal humans with impairment of the pyramidal system, most often occurs in a decorticate pattern: The upper extremity is typically biased toward flexion, such that the limb can be easily moved into flexion but not into extension (decreased compliance of flexors). The lower extremity is biased toward extension, such that the impaired compliance of extensors makes movement into flexion difficult (Figure 10-3).80 In persons with severe acquired brain injury the entire body may be biased toward extension, a condition or posture described as decerebrate pattern spasticity (Sherrington originally described this phenomenon as decerebrate rigidity).80 Both decorticate and decerebrate conditions are unidirectional in nature; there is an increase of muscle stiffness and resistance to passive elongation (impaired compliance) in one group of muscles (agonists) with relatively normal functioning of opposing muscle groups (antagonists).
Spasticity is a velocity-dependent phenomenon. Under conditions of rapid passive elongation, spastic muscle groups “fight back” with increased stiffness, a result of a hypersensitive deep tendon reflex loop (Figure 10-4). This has been described as clasp-knife spasticity, in which the spastic limb “gives” after an initial period of resisting passive movement in the way that a pocket knife initially resists opening when near its initially closed position but then becomes more compliant once moved past a threshold position as it is opened. Growing evidence indicates that the stiffness encountered during passive movement has both neurological (spasticity) and musculoskeletal (changes in muscle and associated soft tissue) components that combine to increase the risk of contracture development.81,82
Given the unidirectional nature of severe hypertonus, it is common for persons with severe hypertonus to develop chronic abnormal postures. The limb or body segment assumes an end-range position that the limb would not normally be able to assume (e.g., extreme equinovarus with marked supination in an individual with severe acquired brain injury, equinovalgus with marked pronation in a child with CP).80,81 If persistent, these fixed postures are associated with a significant likelihood of secondary contracture development.83
Hypertonicity is also associated with deficits in muscle performance, most notably diminished strength, diminished ability to produce power (generate force quickly), diminished ability to effectively isolate limb and body segments, diminished excursions of movements within joints (i.e., moving within a limited range of motion [ROM]), and inefficiency with altering force production or timing of contractions to meet changing (fluid) demands of tasks.84–86 Muscle performance deficits can contribute to an imbalance of forces around a joint that leads to habitual abnormal patterns of movement. These habitual patterns are often biomechanically inefficient and, over time, contribute to the development of secondary musculoskeletal impairments such as adaptive shortening or lengthening of muscles and malalignment of joints.83 Strengthening exercises can have a positive impact on function, even in the presence of hypertonicity.85–87
Rigidity
Individuals with Parkinson’s disease and related neurological disorders often demonstrate varying levels of rigidity: a bidirectional, co-contracting hypertonicity in which there is resistance to passive movement of both agonistic and antagonistic muscle groups.88 Co-contraction of flexor and extensor muscles of the limbs and trunk creates a bidirectional stiffness that interferes with functional movement. Rigidity is often accompanied by slowness in initiating movement (bradykinesia), decreased excursion of active ROM, and altered resting postures of the limbs and trunk (Figure 10-5). The rigidity of Parkinson’s disease can be overridden under certain environmental conditions: Persons with moderate to severe disease can suddenly run reciprocally if they perceive danger to themselves or a loved one; once this initial limbic response has dissipated, they will resume a stooped and rigid posture, with difficulty initiating voluntary movement, limited active ROM, and bradykinesia. Because rigidity creates a situation of excessive stability of the trunk and limbs, orthoses are not typically a component in the plan of care for persons with Parkinson’s disease.89
Hypotonus
Hypotonus (low muscle tone) describes a reduced stiffness of muscle that does not effectively support upright posture against gravity or to generate force during contraction; as a result, hypotonic muscles are more compliant than they are stiff.90 In children, hypotonia can arise from abnormal function within the CNS (approximately 75%) or from problems with peripheral structures (peripheral nerve and motor units, neuromuscular junction, the muscle itself, or unknown etiology).91 Hypotonia can be congenital (seen as “floppy” infants), transient (e.g., in preterm infants), part of the clinical presentation of CP, Down syndrome and other genetic disorders, as well as Autism spectrum disorders.91–96
Hypotonic muscles are considerably more compliant on rapid passive elongation (i.e., less resistant to passive stretch) than muscles with typical tone, as well as those with hypertonus/spasticity. Because their postural muscles are less stiff, individuals with hypotonicity often have difficulty when assuming and sustaining antigravity positions.97 To compensate for their reduced postural tone, persons with hypotonicity may maintain postural alignment by relying on ligaments and connective tissue within joint capsule and muscle to sustain upright posture (Figure 10-6). With overreliance on ligaments in extreme ends of range, further degradation to joint structures often occurs.
In addition to postural control dysfunction, individuals with hypotonia often have difficulty with coordination of movements. This may be due to the decreased efficacy of afferent information collected by a lax muscle spindle during movement execution.98 Children with hypotonia often have impaired control of movements at midrange of muscle length, suggesting that kinesthetic information is not being used efficiently to guide movement or that the ability to regulate force production throughout movements is compromised. In either case, muscle performance is notably less efficient, especially in activities that require eccentric control (e.g., controlled lowering of the body from a standing position to sitting on the floor).97 Individuals with hypotonia have difficulty regulating force production and collaboration between agonists and antagonists is not well coordinated.97
Immediately after an acute CNS insult or injury there is often a period of neurogenic shock in which the motor system appears to shut down temporarily, with apparent loss of voluntary movement (paralysis) and markedly diminished or absent deep tendon reflex responses.98–100 This phenomenon is observed following cervical or thoracic spinal cord injury and early on following significant stroke. During this period, individuals with extreme levels of hypotonus are sometimes (erroneously) described as having flaccid paralysis. Most individuals with acute CNS dysfunction will, within days to weeks, begin to show some evidence of returning muscle tone; over time, many develop hyperactive responses to deep tendon reflex testing and other signs of hypertonicity.99 If they continue to have difficulty activating muscles voluntarily for efficient functional movement, these individuals are described as demonstrating spastic paralysis.
Flaccidity
The term flaccidity is best used to describe muscles that cannot be activated because of interruption of transmission or connection between lower motor neurons and the muscles they innervate.101 True flaccidity is accompanied by significant atrophy of muscle tissue, well beyond the loss of muscle mass associated with inactivity; this is the result of loss of tonic influence of lower motor neurons on which muscle health is based.102
The flaccid paralysis seen in persons with myelomeningocele (spina bifida) occurs because incomplete closure of the neural tube during the early embryonic period (soon after conception) prevents interconnection between the primitive spinal cord and neighboring somites that will eventually develop into muscles of the extremities.103 The flaccid paralysis observed in persons with cauda equina level spinal cord injury is the result of damage to axons of α and γ motor neurons as they travel together as ventral roots to their respective spinal foramina to exit the spinal column as a spinal nerve.104 After acute poliomyelitis, the loss of a portion of a muscle’s lower motor neurons will lead to marked weakness; the loss of the majority of a muscle’s lower motor neurons will lead to flaccid paralysis.65 In Guillain-Barré syndrome, the increasing weakness and flaccid paralysis seen in the early stages of the disease are the result of demyelination of the neuron’s axons as they travel toward the muscle in a peripheral nerve.105 After injection with botulinum toxin, muscle tone and strength are compromised because of the toxin’s interference with transmitter release from the presynaptic component of the neuromuscular junction.106
Fluctuating Tone: Athetosis and Chorea
Athetosis is the descriptor used when an individual’s underlying muscle tone fluctuates unpredictably.107 Athetosis is characterized by random changes in postural tone, with variations from hypertonus to hypotonus. Athetosis, although less common than spastic CP, can have as significant (and often more pronounced) an impact on daily function.108 Although etiology of athetosis is not well understood, it is most frequently described as a type of basal ganglia or thalamic dysfunction associated with bilirubin toxicity or significant perinatal anoxia.107 Persons with athetosis typically demonstrate truncal hypotonia, with fluctuating levels of hypertonus in antigravity musculature and the extremities. In some individuals, movements appear to have a writhing dancelike quality (choreoathetosis) in addition to the tonal influences. Others may compensate for postural instability by assuming end-range positions, relying on mechanical stability of joints during functional activity. Although persons with athetosis are less likely to develop joint contracture than those with long-standing hypertonicity, they are more likely to develop secondary musculoskeletal complications that compromise stability of joints, a result of extreme posturing, imbalance of forces across joint structures, and the need to stabilize in habitual postural alignment patterns for function.109
Postural Control
Postural control has three key dimensions: (1) Static postural control is defined as the ability to hold antigravity postures at rest; (2) dynamic anticipatory postural control is the ability to sustain a posture during movement tasks that shift (internally perturb) the center of mass; and (3) dynamic reactionary postural control is the ability to be able to withstand or recover from externally derived perturbations without loss of balance.110 One has functional postural control if the center of mass (COM) can be maintained within one’s base of support (BOS) under a wide range of task demands and environmental conditions. This requires some level of ability across the triad of static, anticipatory, and reactionary control.
The key interactive CNS systems involved in postural control include extrapyramidal and pyramidal motor systems, visual and visual-perceptual systems, conscious (dorsal column/medial lemniscal) and unconscious (spinocerebellar) somatosensory systems, the vestibular system, and the cerebellar feedback/feedforward systems.111,112 Clinical measures used to assess efficacy of static postural control include timing of sitting or standing activities and measures of center of pressure excursion in quiet standing.113 The Clinical Test of Sensory Interaction and Balance sorts out the contribution of visual, vestibular, and proprioceptive systems’ contribution to balance, as well as the individual’s ability to select the most relevant sensory input when there is conflicting information collected among these systems.114
Measures of anticipatory postural control consider how far the individual is willing to shift his or her center of mass toward the edge of his or her sway envelope.115,116 Clinically, anticipatory postural control is often quantified by measuring reaching distance in various postures.117–119 Measures of dynamic reactionary postural control consider the individual’s response to unexpected perturbations (e.g., when pushed or displaced by an external force, when tripping/slipping in conditions of high environmental demand).120,121 Although these three dimensions of postural control are interrelated, competence in one does not necessarily ensure effective responses in the others.122
Many individuals with neuromuscular disorders demonstrate inefficiency or disruption of one or more of the CNS subsystems necessary for effective postural control and balance.123 An individual with mild to moderate hypertonicity or spasticity often has difficulty with anticipatory and reactionary postural control, especially in high task demand situations within a complex or unpredictable open environment.124 Difficulty with muscle performance, such as impairment of control of force production or the imbalance of forces acting across a joint, might constrain anticipatory postural control in preparation for functional tasks such as reaching or stepping. Impairment of the ability to segment trunk from limb or to individually control joints within a limb may affect the individual’s ability to react to perturbations in a timely or consistent manner.125
Persons with hypotonicity, on the other hand, often have difficulty with sustaining effective postural alignment in antigravity positions such as sitting and standing. They are likely to demonstrate patterns of postural malalignment such as excessive lumbar lordosis and thoracic kyphosis.97 Because of difficulty with muscle force production (especially in midrange of movement), individuals with hypotonia also have difficulty with anticipatory postural control.90 A lack of on-demand motor control contributes to difficulty moving within one’s postural sway envelope; this may be observed as a tendency to stay in one posture for long periods of time, with infrequent alterations in position during tasks.94 Reactionary control may also be altered, particularly with respect to the use of equilibrium reactions and the reliability of protective responses. Individuals with hypotonia have difficulty when a task requires them to absorb small-amplitude perturbations; they must use equilibrium or balance strategies more frequently than persons with adequate muscle tone.90 Additionally, a lack of stability in joints, from both tonal and ligamentous laxity, contributes to an inability to safely stop an accelerating body part as it comes into contact with a surface during a loss of balance episode.94
Movement and Coordination
Many functional activities require us to move or transport the entire body (e.g., mobility or locomotion) or a segment of the body (e.g., using one’s upper extremity to bring a cup toward the mouth to take a drink) through space.126,127 The locomotion task that receives much attention in rehabilitation settings is bipedal ambulation—the ability to walk. For full functional ability, individuals must be able to manage a variety of additional locomotion tasks: running, skipping, jumping, and hopping.128 To fully understand an individual’s functional ability, therapists and orthotists must also consider the environmental context in which ambulation is occurring.129 What are the physical characteristics of the surface on which the individual needs to be able to walk or traverse? Is it level, unpredictably uneven, slippery or frictional, or structurally unstable? Is the ambulation task occurring where lighting is adequate for visual data collection about environmental conditions? Does it involve manipulation of some type of object (e.g., an ambulatory assistive device, a school backpack, shopping bags, suitcases)? Is it occurring in a familiar and predictable environment (e.g., at home) or in a more unpredictable and challenging open environment such as a busy school, supermarket, mall, or other public space? The task demands of locomotion in complex and challenging environments create more demand on the CNS structures involved in motor control (perceptual-motor function, motor planning, cerebellar error control), as well as on the musculoskeletal effectors (muscles and tendons, joints, ligaments, and bones) that enact the motor plan necessary for successful completion of the task that relies on body transport. Individuals with neurological and neuromuscular system problems related to muscle tone, muscle performance, and postural control are typically less efficient, less adaptable, and more prone to fall when walking, especially if there are competing task demands and the environment is complex and challenging.130
The use of a limb segment can also be defined by the nature of the task and the circumstances in which the movement is performed. Upper extremity functional tasks can involve one or more components: reaching, grasping, releasing, manipulating, or any combination of these four purposes.126 Upper extremity tasks can also be defined by considering the function to be accomplished by the movement: grooming, dressing, meal preparation, self-care, or writing. Many upper extremity mobility tasks are founded on effective postural control (e.g., making appropriate anticipatory postural adjustments as the COM shifts while throwing, lifting, lowering, or catching an object).126 Complex mobility tasks require simultaneous locomotion and segmented use of extremities (e.g., reaching for the doorknob while ascending the steps toward the front door, throwing or catching a ball while running during a football or baseball game). For individuals with neurological and neuromuscular system dysfunction, the ability to safely and efficiently perform complex mobility tasks is often compromised due to abnormal muscle tone, impaired muscle performance, and poor postural control.130
Coordination can be thought of as the efficacy of execution of the movement necessary to complete a task. A well-coordinated movement requires effective simultaneous control of many different dimensions of movement: the accuracy of a movement’s direction and trajectory; the timing, sequencing, and precision of muscle activation; the rate and amplitude of force production; the interaction of agonistic and antagonistic muscle groups; the ability to select and manage the type of contraction (concentric, holding, or eccentric) necessary for the task; and the ability to anticipate and respond to environmental demands during movement.131 Coordination can be examined by considering the individual’s ability to initiate movement, sustain movement during the task or activity, and terminate movement according to task demands.132
For movement and coordination to be functional, one must have muscle performance that is flexible/adaptable to varying demands.133 Mobility or transport tasks cannot be performed independently (safely) unless the individual is able to (1) transition into and out of precursor positions (e.g., getting up from the floor into a standing position, rising from a chair), (2) initiate or begin the activity (e.g., take the first step), (3) sustain the activity (control forward progression with repeated steps), (4) change direction as environmental conditions demand (e.g., step over or avoid an obstacle), (5) modulate speed as environmental conditions demand (e.g., increase gait speed when crossing the street), and (6) safely and effectively stop or terminate the motion, returning to a precursor condition or position (quiet standing, return to sitting).133
For individuals with neuromuscular disorders who have difficulty with muscle performance, abnormal underlying tone, or poor postural control, coordination of functional movement can be compromised in several ways. In order to approach and complete a movement task, the individual might rely on abnormal patterns of movement with additional effort and energy cost.134 Many individuals with hypertonicity initiate movement with strong bursts of muscle contractions but have difficulty sustaining muscle activity and force of contraction through the full ROM necessary to perform a functional movement.98,108 Deficits in timing and sequencing of muscle contractions, as well as difficulty with dissociation of limb and body segments, also contribute to difficulty with performance of functional tasks.135 An adult with hemiplegia following a cerebrovascular accident may be able to initiate a reach toward an object but not be able to bring the arm all the way to the target.136 The same individual may have difficulty with timing and segmentation, leading to inability to open the hand before reaching the target in preparation for grasping the object.137,138 The muscle performance of many children with CP is compromised by inappropriate sequencing of muscle contractions when activation of synergists and antagonists happens simultaneously.108 Conversely, an individual with hypotonus who has difficulty with stabilization often moves quickly with diminished accuracy and coordination.90
The following tables provide an overview of incidence/prevalence, etiology and risk factors, clinical presentation, and impact on muscle tone, muscle performance, postural control, and movement for of the most common pathologies that might include use of an orthosis: stroke (Table 10-1), CP (Table 10-2), spina bifida (Table 10-3), MS (Table 10-4), and spinal cord injury (Table 10-5).
Table 10-1
Also known as | Brain attack, cerebrovascular accident (CVA) |
Incidence | Approximately 610,000 per year in the U.S.* |
Prevalence | 2.6% of total US population have had stroke* 8.1% of US population over age 65 have had stroke* |
ETIOLOGY AND RISK FACTORS | |
Ischemic Stroke (88%) | |
Thrombus | Hypertension, hypercholesterolemia, diabetes and metabolic syndrome, overweight and obesity, smoking, sedentary lifestyle* |
Embolism | Atrial fibrillation, atherosclerosis in carotid/vertebral arterial systems; previous embolic stroke |
Hemorrhagic Stroke (12%) | |
Intracranial hemorrhage | Uncontrolled hypertension, ruptured aneurysm |
STROKE SYNDROMES (BY ARTERY) | |
Middle cerebral (most common) | Contralateral hemiparesis/hemiplegia, lower face, UE > LE Contralateral sensory loss of lower face, UE > LE Contralateral homonymous hemianopia (optic tract) Possible dysarthria and dysphagia R hemisphere: visual-spatial or somatic perceptual impairment L hemisphere: communication impairment (various aphasias) |
Lenticulo-striate (lacunar MCA) | Contralateral hemiparesis/hemiplegia, lower face, UE = LE Cortical functions (perception, communication) intact |
Anterior cerebral | Contralateral hemiparesis/hemiplegia LE > UE Sensation often intact or only mildly impaired (contralateral) Incontinence “Alien hand” syndrome (involuntary/unintended movement) Motor (nonfluent/Broca’s) aphasia may occur |
Posterior cerebral | Contralateral homonymous hemianopsia (optic radiations) Visual inattention L hemisphere: alexia (unable to read), with ability to write preserved |
Thalamogeniculate (lacunar PCA) | Contralateral sensory loss, often severe Sensory ataxia (uncoordinated movement due to lack of proprioception) Thalamic pain and hyperpathia syndrome |
Basilar (complete) | Loss of consciousness, coma High mortality |
Superior cerebellar | Ipsilateral ataxia, falling to side of lesion Intention tremor Contralateral loss of pain temperature sensation from body Contralateral loss of proprioception Ipsilateral Horner’s syndrome (meiosis, ptosis, anhydrosis) |
Anterior-inferior cerebellar | Ipsilateral facial paralysis (both upper and lower face) Ipsilateral loss of pain and temperature of entire face Contralateral loss of pain and temperature from body Loss of taste sensation, loss of corneal reflex, ipsilateral hearing loss, nystagmus, vertigo, nausea Ataxia and incoordination of limb movement Ipsilateral Horner’s syndrome (meiosis, ptosis, anhydrosis) |
Vertebral | Contralateral loss of pain, temperature sensation from body |
Posterior-inferior cerebellar (Wallenberg’s syndrome) (lateral medullary syndrome) | Ipsilateral loss of pain and temperature sensation from face Ipsilateral Horner’s syndrome (meiosis, ptosis, anhydrosis) Dysphonia, dysphagia, dysarthria, diminished gag reflex Nystagmus, diplopia, vertigo, nausea Ipsiversive falling (toward side of lesion), incoordination, ataxia |
PROGNOSIS | Ischemic: severity depends on site of occlusion within arterial tree and the size of the area that is without blood flow Embolic: risk of recurrence is higher than in thrombosis; risk of hemorrhage at site of embolism Hemorrhagic: highest morbidity and mortality Static event, with evolving symptoms in weeks/months following initial damage, due to initial inflammatory response and subsequent tissue remodeling/healing. |
MUSCLE TONE | Initial hypotonus (sometimes appearing to be flaccid) due to neurogenic shock. Some individuals remain hypotonic, most develop various levels of hypertonus in weeks/months following event. Hyperactive deep tendon reflexes evolve over time. |
MUSCLE PERFORMANCE | Upper extremity often biased toward flexion, with lower extremity biased toward extension. Impaired force production, speed and power, eccentric/isometric control, accuracy, and fluidity. |
POSTURAL CONTROL | Frequently impaired, especially if lesion included gray matter of R hemisphere, with perceptual dysfunction. |
MOBILITY AND COORDINATION | Asymmetry in ability to use trunk, limbs during functional .activity; tendency to move in abnormal “synergy” (flexion UE, extension LE). Frequently require AFO and ambulatory device. |
*American Heart Association Risk Factors for Stroke. Available at: http://www.heart.org/HEARTORG/General/Risk-Factors_UCM_319118_Article.jsp; accessed 8/9/11.
Table 10-2
Incidence | Approximately 11,500 new cases diagnosed per year* |
Prevalence | 764,000 children and adults in the U.S.; 3.3 per 1000* |
Etiology | Unknown in most instances |
Risk factors | Prematurity, high-risk pregnancy, low birth weight, perinatal or neonatal respiratory distress/anoxia, mother’s substance abuse while pregnant |
TYPES OF CEREBRAL PALSY | |
Spastic (85%-93%)*,† | Quadriplegia/tetraplegia: global impact on both gray and white matter structures in forebrain. Some individuals have concomitant cognitive impairment and seizure disorders. Diplegia: usually due to damage of white matter of internal capsule/corona radiate, impacting on motor and sensory systems more than perceptual. Hemiplegia: may be due to intracerebral hemorrhage or ischemia affecting one hemisphere. |
Dyskinetic (5%-10%)*,† (choreoathetoid) | May be related to perinatal damage of basal ganglia Unpredictably fluctuating tone of head/neck, trunk, and extremities; may be associated with perinatal bilirubin toxicity |
Ataxic/cerebellar (5%) | May be related to perinatal damage of cerebellar cortex, nuclei, or peduncles |
PROGNOSIS | Static event occurring at or around the time of birth. Signs and symptoms become apparent over first 2 years of life as motor developmental delay. High risk of secondary musculoskeletal deformity due to impact of abnormal tone during periods of growth and repetitive impact of muscle imbalance on movement. Level of disability often becomes more pronounced as child moves into middle childhood, adolescence, and young adulthood due to increasing body size and weight. |
MUSCLE TONE | |
Spastic | Initial hypotonus in premature and low-birth-weight infants common. Hypertonus develops over time; severity of hypertonus varies depending on extent of central nervous system damage. Hypertonus may increase in times of growth, or in times of excitement and stress. Hyperactive deep tendon reflexes, as well as abnormal tonic developmental reflexes. |
Dyskinetic | Unpredictable fluctuation of muscle tone (from very low tone to significant hypertonus), affecting muscles of limbs, axial skeleton, respiration, and phonation. Often appears as writhing motions of trunk and extremity. Response to tendon reflexes varies due to changing tone. |
Ataxic | Frequently hypotonic with hyperflexible joints in both appendicular and axial skeleton. At risk for secondary overuse injury with habitual and long-term hyperflexibility during movement and in static postures. Often pendular deep tendon reflexes. |
MUSCLE PERFORMANCE | |
Spastic | May be hypokinetic (small excursion of movement) or hyperkinetic (large excursion of movement) with difficulty modulating force; force production can be great (strong forceful unrelenting contraction) or small (inability to sustain contraction for functional movements); difficulty with eccentric and isometric control, poor accuracy and precision of movement. |
Dyskinetic | May be hypokinetic (slow and minimal movement) or hyperkinetic (movements can be random and/or repetitive with minimal purpose); force production, speed, and timing of contractions do not match task demands; poor accuracy. |
Ataxic | May be hypokinetic or hyperkinetic; tasks demanding greater accuracy lead to faster and greater excursions, with worsening accuracy; tasks demanding less accuracy lead to slower and lesser excursions with some accuracy. Timing of agonist and antagonist sequencing is impaired. |
POSTURAL CONTROL | |
Spastic | Often impaired, due to influence of persistent tonic reflexes, and less efficient development of righting and equilibrium responses in trunk and affected limbs. |
Dyskinetic | May be significantly impaired; fluctuating tone challenges ability to attain and hold positions or move dynamically through space. |
Ataxic | Sitting and standing postures may be “floppy,” as child relies on soft tissue structures of the musculoskeletal system to support upright position because of inadequate antigravity postural control. |
MOBILITY AND COORDINATION | |
Spastic | Frequently employ movement strategies based on atypical and less variable patterns/synergies; over time may develop rotational deformity of lower extremity as well as joint contracture due to combination of preferred abnormal positions/patterns of movement and hypertonus. Often require ankle-foot-orthosis, assistive devices, or wheelchair for locomotion. Those with significant trunk and upper extremity involvement may require adaptive equipment for feeding, communication, and activities of daily living. Orthoses or resting splints may be used as a means of managing abnormal tone, minimizing risk of musculoskeletal deformity, or after orthopedic surgery to correct deformity. |
Dyskinetic | Often depend on external support for stability during functional tasks and locomotion. May benefit from use of ambulatory device, adaptive equipment, seating systems, or upper and lower extremity orthoses. |
Ataxic | Depending on severity of impairment, may have difficulty with trunk stability during functional tasks using upper extremity (activities of daily living), and lower extremity (walking). May use ankle-foot orthosis to provide external support/positioning during functional task. |
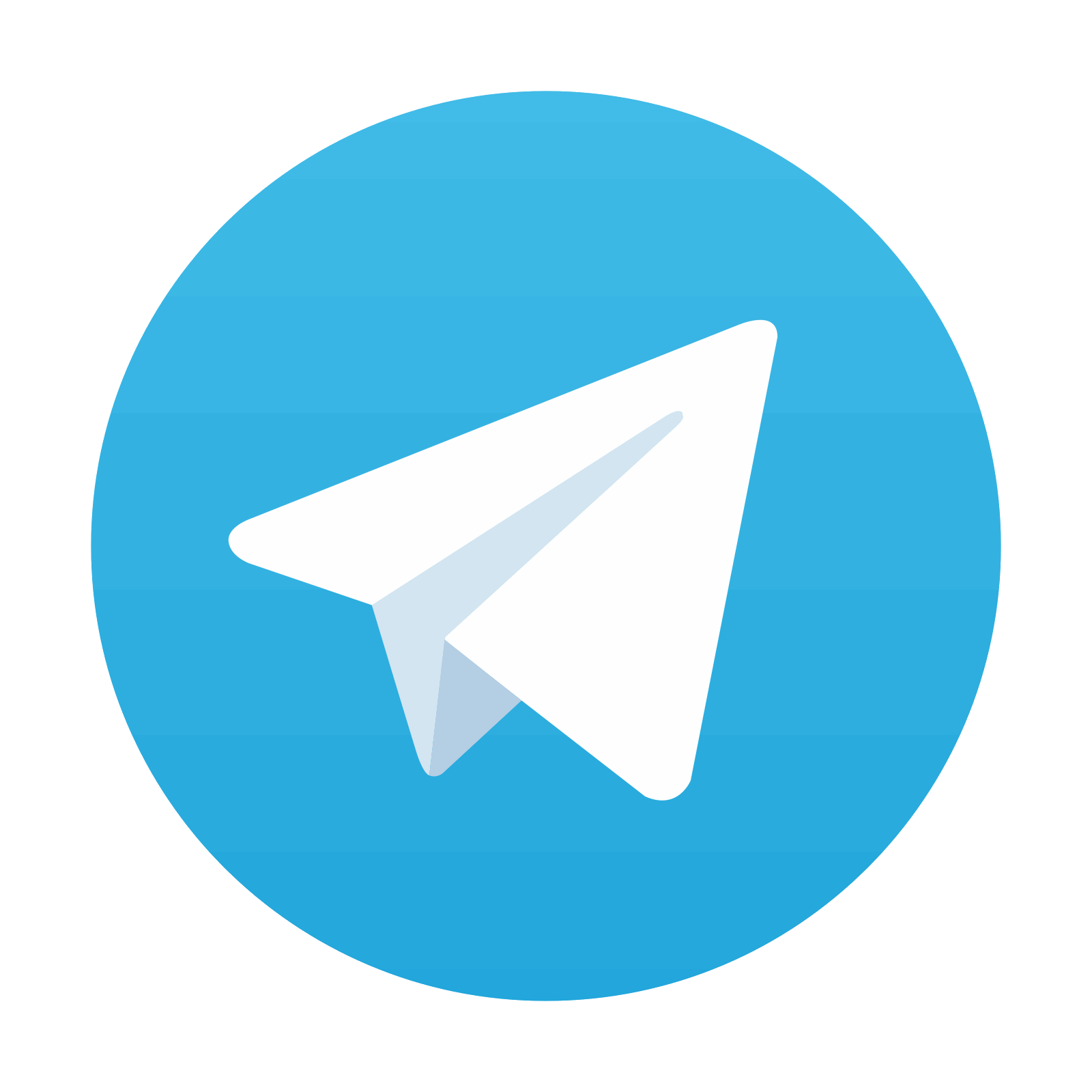
Stay updated, free articles. Join our Telegram channel

Full access? Get Clinical Tree
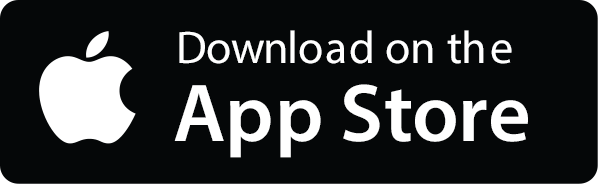
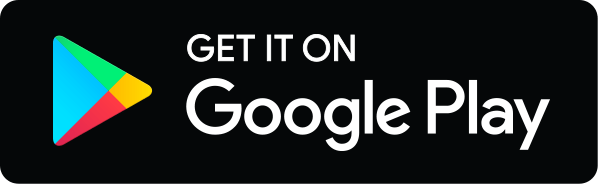