22 OCULAR INJURIES
Ocular injury is the leading cause of monocular blindness in the United States and is second only to cataracts as the most common cause of visual impairment.1 Trauma is the most frequent reason for eye-related visits to hospital emergency departments. Most eye injuries are minor and result in no permanent visual damage. For severe injuries, a substantial proportion of patients experience poor visual outcomes.2
As of 2004 the majority of eye injuries (37.8%) occurred in the home. Blunt objects (32.3%) account for the most common source of injury. Fifty-six percent of eye injuries occur in people younger than age 30, and males outpace females by a 4:1 ratio for eye trauma. The leading cause of bilateral eye injury remains motor vehicle crash. Construction injuries account for the majority of occupational-related injuries. Baseball and softball (34.6%) are two of the most common causes of sports-related eye injuries.3 Falls account for the majority of injuries in the elderly (Table 22-1).
The immediate goals of eye injury management are:
• Protection of intact visual structures
• Prevention of further ocular damage
• Accurate assessment of the injury and appropriate triage or referral of the patient
• Timely initiation of the best possible care by qualified medical personnel
The Ocular Trauma Classification (OTS) Group developed a standardized system for classifying mechanical eye injuries based on the Birmingham Eye Trauma Terminology (BETT). BETT makes descriptions of eye trauma consistent and accurate by providing a clear framework for defining each type of injury4 (Table 22-2). OTS categorizes both open globe and closed globe injuries by four parameters: type of injury, grade or visual acuity, pupil function, and zone of the injury.5 Under the open-globe classification for injury type, penetrating injuries are limited to those that only have an entry site, whereas perforating injuries have entry and exit sites and rupture indicates the cause of injury is due to blunt compression of the globe.6
TABLE 22-2 Ocular Trauma Classification System
Open-Globe Injury Classification |
Type |
Grade |
Visual acuity*: |
Pupil |
Zone |
Closed-Globe Injury Classification |
Type |
Grade |
Visual acuity*: |
Pupil |
Zone‡ |
* Measured at distance (20 ft, 6 m) using Snellen chart or Rosenbaum near card with pinhole when appropriate.
† Confirmed with bright light source and fellow eye well occluded.
‡ Requires B-scan ultrasonography when media opacity precludes assessment of more posterior structures.
Kuhn F, Pieramici D, editors: Ocular Trauma Principles and Practice, New York, 2002, Thieme.
ANATOMY AND PHYSIOLOGY
INTRAOCULAR STRUCTURES
The eye and its adnexal structures are as complex anatomically and functionally as they are in embryonic development. Light rays enter the eye through the cornea, pupil, and lens and fall on the diaphanous retina (Figure 22-1), which activates the retinal photoreceptor elements, the rods, and cones. Rods are responsible for night vision and function best in dim lighting. Cones (there are three types: red, blue, and green) are responsible for color and detailed vision; they function best in bright light. Cones predominate in the macula. The macula is the only site capable of 20/20 vision. Through complex synaptic interconnections among a variety of cell types, the rods and cones transmit the light messages they receive to the 1 million retinal ganglion cells, whose axons are gathered together at the optic disc and form the optic nerve. The optic disc (Figure 22-2) measures 1.5 mm in diameter and contains a central depression, or cup, which averages one third the disc diameter. As the axons leave the globe, they travel for approximately 1 mm through the sclera. They are then covered by dura and arachnoid while extending 25 to 30 mm through the orbit, 4 to 9 mm through the optic canal, and 10 mm intracranially before forming the optic chiasm and finally terminating deep in the brain substance.
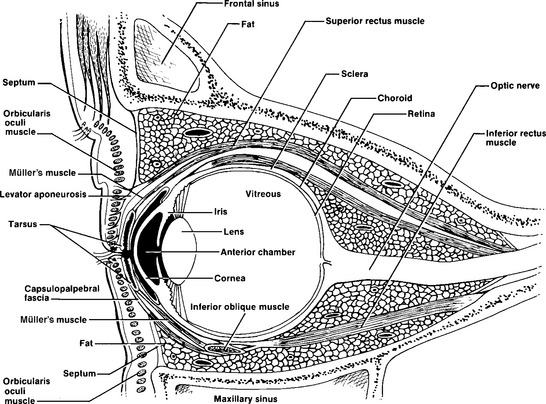
FIGURE 22-1 Ocular and periocular anatomy, including vascular supply and innervation of ocular structures.
In front of the iris lies the anterior chamber, which contains the aqueous fluid produced by the ciliary body. Aqueous humor is continuously produced by the ciliary body. It moves forward through the posterior chamber and pupil into the anterior chamber, where it drains out via the trabecular meshwork and Schlemm’s canal. These structures are located in the angle created by the junction of the iris, cornea, and sclera. The sclera blends into the cornea, an avascular, crystal-clear, convex disc-like structure. It is covered by a layer of epithelium five to six cells thick, which can regenerate in 24 to 48 hours when scratched or abraded. The cornea becomes continuous with the epithelium of the conjunctiva, the mucous membrane that lines the posterior surface of the eyelids and the anterior portion of the sclera. Overlying the cornea and conjunctiva is the tear film, which is composed of lacrimal, mucinous, and lipid gland secretions. These secretions are produced by the lacrimal and accessory lacrimal glands, conjunctiva goblet cells, and meibomian glands. An adequate tear film evenly distributed across the cornea and an intact corneal epithelium are essential factors for achieving clear vision.
PERIOCULAR AND ORBITAL STRUCTURES
The eyelids cover and protect the globe. They also distribute the tear film across the cornea and aid in the removal of excess tears and tear film debris. The eyelids can be divided into five layers. Most posterior is the conjunctiva. Anterior to this in the upper lid is Müller’s muscle, a structure that is partially responsible for eyelid elevation. Müller’s muscle is attached to the tarsus, a dense, fibrous connective tissue structure containing the meibomian glands, that provides structural support for the upper and lower eyelids. Anterior to Müller’s muscle and the tarsus is the levator muscle complex in the upper eyelid. The capsulopalpebral fascia is an analogous structure in the lower eyelid that attaches to the inferior border of the tarsus. The third cranial nerve innervates the levator muscle, which is responsible for elevating the eyelid. For this reason, ptosis (droop) (Figure 22-3) may be present with third nerve paresis. Because parasympathetic fibers to the iris sphincter muscle also travel in the third nerve, a dilated (mydriatic) pupil may be associated with third nerve paresis. Anterior to the levator muscle complex is the orbicularis muscle, a structure innervated by the seventh cranial nerve. When this nerve is paretic, as in Bell’s palsy (Figure 22-4), the eyelids cannot close, resulting in tear film evaporation and corneal epithelial damage. Corneal ulceration can occur if this is not treated promptly. Skin is the final structure covering the eyelid. A component of the eyelid that cannot be overlooked is the orbital septum, which is a continuation of the periosteum covering the bony orbit. This structure extends from the orbital rim and attaches to the levator muscle complex in the upper lid and the capsulopalpebral fascia in the lower lid. The orbital septum represents the boundary between the orbital and periorbital structures. Violation of this protective barrier exposes the orbital contents to external forces, specifically infectious agents. Infection can involve the entire orbit, causing subsequent visual loss and possible intracranial spread, resulting in meningitis and abscess formation.
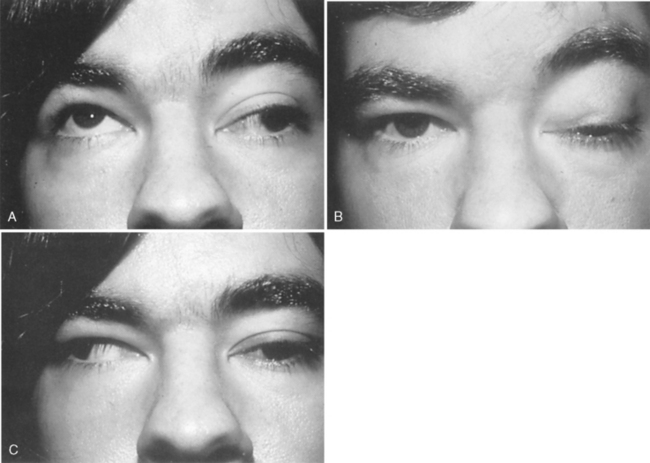
FIGURE 22-3 Ptosis associated with left third nerve paresis. A, Upgaze. B, Primary gaze. C, Right gaze.
The globe lies within the bony orbit, which consists of the maxillary, lacrimal, ethmoid, greater and lesser sphenoid, frontal, palatine, and zygomatic bones. These bones are joined to form a quadrilateral pyramid with its apex located posteriorly. The anterior opening of the adult orbit measures approximately 35 mm in height and 40 mm in width. The orbit is 40 to 45 mm deep. Superior to the orbit are the frontal sinus anteriorly and the anterior cranial fossa posteriorly. Medial to the orbit are the ethmoid and sphenoid sinuses and the nasal cavity. Inferiorly is the maxillary sinus. Laterally are the temporalis fossa anteriorly and the middle cranial fossa, temporal fossa, and pterygopalatine fossa posteriorly. Posterior to the orbital apex are the clinoid processes, pituitary gland, cavernous sinus, carotid arteries, middle cranial fossa, and optic chiasm. Because the globe and orbit are close to many important nonocular structures, severe ocular injury is often seen in conjunction with serious nonocular injury.
Tear secretions exit through the lacrimal drainage system (Figure 22-5). Any damage to this system may result in epiphora (excessive tearing) and infection. Puncta (orifices leading to the lacrimal drainage system) are present in the medial aspect of the upper and lower eyelids. These lead to the canaliculi, which connect to the lacrimal sac. This latter structure lies within the lacrimal sac fossa and posterior to the medial canthal tendon. Tear secretions flow from the lacrimal sac into the bony nasolacrimal duct within the maxillary bone before entering the nose.
PREVENTION
The majority of eye injuries are preventable if existing protective devices, such as seat belts, safety helmets, and protective eyewear, are used. Seat belts have reduced the incidence of eye injuries from 65% to 47%.5 Airbags have been identified as culprits of ocular injury, although it is often not possible to determine if airbag deployment coexists with or actually is the cause of eye injury. In many cases airbags can serve to protect the eyes. Without airbags the possibility exists that injury may be more serious or even fatal.5,7
Eye protection devices are available for specific work and recreational activities. Neither regular glasses nor contact lenses alone provide adequate protection for environments involving projectile objects, chemicals, particulate matter, intense heat or radiation energy. Polycarbonate lenses in polyamide frames with a posterior retention rim are the most effective in preventing eye injury. Solid wrap-around frames should be used (rather than hinged frames) because they better withstand lateral blows.8 Certain athletic guards without lenses do not provide adequate protection. A high incidence of eye injuries in ice hockey players led to the mandated use of face visors in youth hockey, and, a subsequent reduction in the incidence of injuries.6 Standards for protective eyewear for specific sports have been defined by the American Society for Testing Materials and the Canadian Standards Association.
A variety of ocular safety protectors are available for occupational uses. The United States Department of Labor Occupational Safety and Health Administration (OSHA) sets and enforces standards to improve workplace safety and health, which includes recommended protective eyewear for specific occupations. Information about occupation-specific OSHA recommended protective eyewear can be accessed on-line at www.osha.gov. In the industrial setting eye protection devices must meet the standards of American National Standards Institute (ANSI) Z87 Standard Practice for Occupational and Educational Eye and Face Protection. In addition to goggles, a face shield offers best protection for specific occupations, such as those involving radiation and light (welding) or flying particles (grinding).
Despite many educational campaigns and legislative efforts, the compliance with protective gear is low. For example, welders and grinders often lift up the face shield to inspect work or to perform touch-up grinding. A majority of injuries occur in the home, where regulations regarding protective eyewear are not enforced. It is important for educational campaigns to emphasize use of appropriate properly maintained eye protection while working in industry or the home and for consumer products to contain information regarding the need for eye protection on the labeling.
TRAUMA MANAGEMENT
In some areas, centers that specialize in the care of complex ocular injuries have been established and referral to such a center may be made once other life-threatening injuries are ruled out. Initial assessment and classification of patients presenting to the ophthalmic emergency room, known as ocular triage, is performed to determine the priority of treatment. An ocular triage exam consists of obtaining (1) vital signs, (2) a brief description of the injury, and (3) information about known allergies and level of pain. During triage health care professionals should initiate immediate therapy when required, for example, irrigating the eye in the setting of a chemical injury, or immobilizing or protecting an object protruding from the globe to prevent further trauma. Once the ocular triage is complete, the patient should receive an ocular screening exam, which consists of obtaining a pertinent ocular history and external examination of the eye including the periorbital area.9
ASSESSMENT
An ocular trauma assessment begins with an ocular history. The ocular history provides information that can help in diagnosis, initial management, and prognosis of eye injury. Patient-reported symptoms of vision loss or blurred vision that does not improve with blinking, double vision, sectorial visual loss, or ocular pain are important findings and require immediate referral to an ophthalmologist. The mechanism, time and location of injury and course of events, symptoms, and any therapeutic interventions provided must be determined. It is important to ascertain whether eye injury, ocular disease (e.g., glaucoma, macular degeneration), systemic disease that may impair sight (e.g., diabetes, hypertension), or visual impairment was present before the traumatic event. The history should also include medications the patient is taking and specifically whether the patient has received or is currently receiving any ocular therapy (i.e., medication or surgical intervention).9 When the patient cannot provide an accurate history, it may be necessary to obtain an ocular history from other sources, when available, such as family, friends, or the patient’s personal ophthalmologist.
A few instruments are needed to examine the patient with ocular trauma. A Snellen chart, near-vision card and pinhole occluder are useful to assess visual acuity. A penlight with a cobalt blue filter, tonometer, Hertel exophthalmometer, and ophthalmoscope will help in other parts of the examination. A slit-lamp biomicroscope, will aid in the examination of the conjunctiva, cornea, anterior chamber, iris, lens and anterior vitreous cavity. Topical anesthetic agents such as Proparacaine will help in examining patients who are unable to open their eye due to a corneal abrasion. A lid retractor (Figure 22-6) may be required for evaluating infants or patients with corneal injury. Sodium fluorescein test strips are needed to evaluate the extent of the corneal epithelial loss. These are used with a cobalt blue filter covering a penlight. Short acting dilating drops such as 2.5 percent phenylephrine or 1 percent tropicamide (Table 22-3), both of which have a dilatation action lasting 3 to 6 hours, are essential for adequate examination of the ocular fundus. These agents must be used with great caution with any patients with known or suspected intracranial trauma.
Visual Acuity
The most common eye chart utilized for evaluating visual acuity is the Snellen chart. The Snellen chart displays lines of block letters of diminishing size, each defined according to the distance at which the line of letters can be read by a person with normal visual acuity. Visual acuity measured using the Snellen chart is expressed by a numerator, indicating the distance the patient is from the chart during testing (20 feet), and a denominator which is the smallest line of the print the patient can read at that testing distance.10 If a standardized distance or near visual acuity chart is not available, a newspaper or some other printed material can be substituted. Visual acuity can then be recorded as the size of print (e.g., newspaper headline, print at the top of an order sheet) the patient sees at a specified distance, usually 14 inches. For children or patients who are illiterate, a picture chart can be used.
For children between the ages of 6 months and 2 years, vision is assessed using the fix-and-follow method. Using this method, first observe the patient with both eyes open; then, with one eye occluded at a time, determine whether the patient stares or fixates on a stationary object (e.g., face, light) and pursues or follows a moving target at arm’s length.10 If the patient is unable to appropriately fixate and follow, the response is documented as abnormal.
Pupils
Last, the response to the “swinging flashlight” test is observed. In a dimly lit room with the patient fixating on a distant object, light is shined on one eye for 3 seconds and is then quickly moved in a swinging motion across the bridge of the nose into the opposite eye while pupil response is noted. In patients without visual pathway disturbances and normal iris function, the eye will not constrict or dilate to the swinging light. This is because direct and consensual responses are equal in the normal patient. The examiner swings a light back and forth over the bridge of the nose from one pupil to the other. If the eye paradoxically dilates when exposed to the light source, the eye has a relative afferent pupillary defect and is sometimes known as a Marcus Gunn pupil. In trauma patients, if the optic nerve is damaged, the pupil on that side will paradoxically dilate. In some cases of severe retinal damage or intraocular hemorrhage, there can be some afferent pupil defect, although not always.
Ocular Motility
Examination of ocular motility screens for abnormal eye movements and ocular malalignment. Instruct the patient to follow your finger or a penlight, which is moved from straight ahead to the far right and left and then up and down. The eyes should move an equal amount at the same speed in each gaze direction. There are nine cardinal gaze positions: straight ahead, directly up, up and to the right, directly lateral, lateral and down, directly down, down and laterally left, directly left, and up and to the left. However, in most clinical situations, measuring straight ahead, directly up, left, right, and down provides enough information to assess for the presence of a deviation. In an unconscious patient with an uninjured cervical spine, the “doll’s eye” maneuver, or oculocephalic reflex, can be tested to evaluate ocular motility. Rapid, passive side-to-side head turning is performed; the normal response is movement of the eyes in the direction opposite the head movement. Forced duction testing (Figure 22-7) may be indicated to rule out muscle entrapment if an orbital floor fracture is suspected. This involves anesthetizing the eye with topical anesthesia, grasping the limbal conjunctiva and sclera with toothed forceps, and rotating the globe up and down and right and left to test the muscles that control ocular motility while noting any restriction to free movement of the globe. This test is contraindicated in cases of open globe injury. Common causes of abnormal ocular movements include orbital edema or hemorrhage; extraocular muscle entrapment; and damage to cranial nerves III, IV, or VI.
< div class='tao-gold-member'>
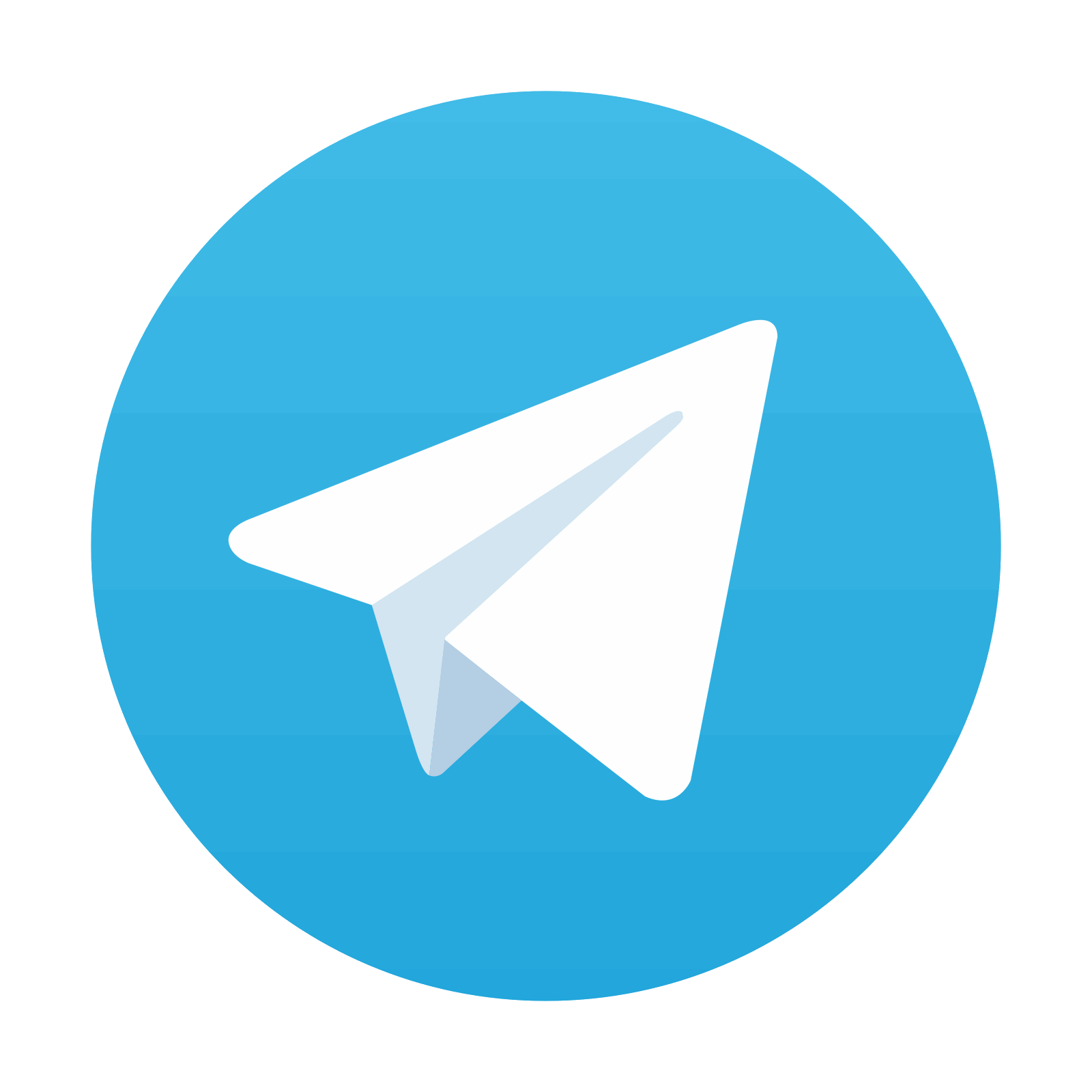
Stay updated, free articles. Join our Telegram channel

Full access? Get Clinical Tree
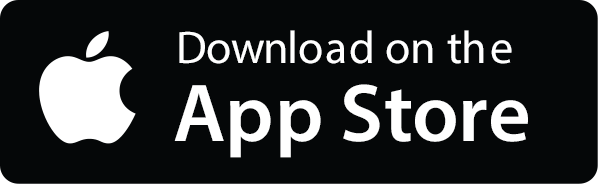
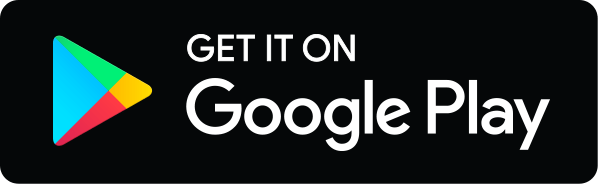