Energy and calories
Energy is measured in Joules, however the term most often used in dietetics and amongst athletes is the kcal (one kcal equals 4.2 kJoules). To maintain energy balance, the estimated average energy intakes required in ‘healthy’ adults are 2550 kcal/day for males and 1940 kcal/day for females (FSA 2008). Additional physical activity needs, however, are not accounted for in these estimated average requirements. Therefore athletes in training will have higher energy requirements and this will increase in proportion to the volume of training that the athlete performs.
Nutrients and where to get them
In addition to energy, food and drink provide nutrients. These are the raw materials, or ingredients, required by the body for optimal health and function.
There are six key nutrient classes. These are:
- carbohydrates
- fats
- proteins
- vitamins
- minerals
- water.
Each of these nutrient classes has specialised roles within the body that are essential for health and optimal performance. An inadequate intake of any of these will result in disease, or impaired physical and cognitive functioning, which can normally only be prevented by the nutrient. Of these nutrients, carbohydrates, fats and proteins provide energy. Water, vitamins and minerals provide no energy but play a vital role in the regulation of body processes. Alcohol is considered by some to be the seventh nutrient as it also provides a source of energy, but it is not essential.
The correct nutrient balance will help athletes remain healthy and perform optimally. Too much or too little of any one nutrient will affect both health and performance.
Carbohydrates
Carbohydrates are the single most important source of energy for athletes. They provide approximately four kcal per gram and are the primary fuel source for high intensity exercise (above 60% VO2max) (Coggan and Coyle 1988; Carter et al. 2003). Carbohydrates can be classified as simple and complex.
Simple carbohydrates are found in sugary foods such as fruit, fruit juices, sugar and honey and generally provide a quick but short-lasting source of energy. These can be further divided into: monosaccharides, which are simple, one-unit sugars such as glucose, fructose and galactose; and disaccharides, which are formed from two monosaccharides. For example sucrose – or common table sugar – is a combination of glucose and fructose.
Complex carbohydrates are found in starchy foods such as pasta, rice, potatoes, bread, vegetables, cereals, beans and pulses and provide a stable supply of longer-term energy. Complex carbohydrates can be further divided into: polysaccharides (containing more than two monosaccharides), which include starch (from plant sources) and glycogen (from animals), and dietary fibre (non-starch polysaccharide), which is found in plant foods, cereal, fruit and vegetables and is crucial to optimal health.
Foods, and in particular carbohydrates, can also be classified by their glycaemic index. The glycaemic index (GI) is a measure of the extent to which a certain food raises blood glucose (Jenkins et al. 1981). High GI foods, such as white bread, potatoes, cornflakes and jelly beans, cause a sharp rise in blood glucose, which is often short lasting and followed by a rebound drop in blood glucose. Low GI foods, such as porridge, high-fibre cereal, beans, peanuts and apricots, which are high in fibre and/or protein, give a slower, but more sustained release of glucose causing a slower rise in blood glucose and no rebound drop.
For the healthy population, carbohydrates should contribute approximately 50–60% of the energy intake in the diet, with low GI, high-fibre complex carbohydrates providing the majority of this requirement (Salmeron et al. 1997; Foster-Powell et al. 2002). This should increase to approximately 60–70% in an athletic population.
Protein
Protein plays a vital role in the maintenance of all body tissues. It is used as the structural basis of all cells, forms the contractile components of muscle, and is used to synthesise haemoglobin, enzymes, hormones, neurotransmitters and antibodies, helping to maintain the immune system and regulate all essential chemical reactions. Protein, which contains approximately 4 kcal/gram, is also used as an energy source, supplying about 5–10% of energy expenditure (Dohn 1986; Brooks and Mercies 1994).
Proteins are made up of amino acids, and it is these amino acids that are the real building blocks of the body. There are 20 amino acids, 12 of which can be synthesised from other amino acids and are considered non-essential and, and eight (nine for children) that are considered essential as they cannot be made within the body and therefore must come from the diet.
Protein is found in a variety of foods, however not all foods contain all of the essential amino acids. Those that do contain all the essential amino acids are termed complete proteins, which can be found in animal products such as meat, fish, poultry, eggs, milk and yogurt, as well as soya. Incomplete proteins have one or more of the essential amino acids missing. These are usually found in plant foods such as beans, pulses, vegetables, grains and rice. In order to get all of the essential amino acids athletes should either aim to eat complete proteins, such as those gained from animal sources, or combine other proteins to ensure that their essential amino acid needs are met.
The recommended intake of protein for a healthy sedentary person is 0.75g/kg.bw/day or approximately 15% of the diet (FSA 2008).
Fat
Fat has many essential functions within the body that include: energy provision, formation of cell membranes and nerve fibres, protection of vital organs, production of hormones, storage and transport of fat soluble vitamins, insulation, suppression of hunger and adding palatability to foods.
The body can store large amounts of energy as fat, mostly in the form of subcutaneous adipose tissue, which can be mobilised and transported to the working muscles as a fuel source during exercise, but also as intramuscular fat where it is more readily utilised within the muscles.
Fat contains approximately 9kcal/gram making it a very efficient way for the body to store large amounts of energy. High fat foods are therefore considered energy dense, making it easy for athletes to inadvertently consume too much energy from these foods. Managing fat intake is therefore a primary concern for athletes to prevent any unwanted increase in body fat.
Athletes should also consider the type of fat in the diet. Naturally occurring dietary fat can be either unsaturated or saturated. Unsaturated fatty acids contain one (monounsaturated) or more (polyunsaturated) double bonds between carbon atoms where each double bond replaces two hydrogen atoms. Saturated fatty acids contain no double bonds. Unsaturated fatty acids are generally liquid at room temperature and are found in plant sources and fish. Saturated fats tend to be solid at room temperature and come from animal sources. Saturated fats are associated with increased levels of low-density lipoproteins (LDL cholesterol) (Mustad et al. 1997) and an increased risk of coronary heart disease, whereas unsaturated fats are associated with a lower risk by reducing serum LDL (Kratz et al. 2002) and increasing high-density lipoproteins (HDL cholesterol) (Karmally and Goldber 2006).
Trans-fatty acids are formed by a process called hydrogenation. Vegetable oils are chemically changed to give them a higher melting point, effectively making them more solid at room temperature, and are used in the manufacture of margarine and other spreads. When unsaturated fatty acids are altered, and acquire some of the properties of saturated fatty acids through hydrogenation, they are termed trans-fatty acids.
All fats contain a mixture of fatty acid types, but it is the proportion of different fatty acids that makes some fats healthier than others. Fats associated with raised cholesterol levels and heart disease include those high in saturated animal fats (red meat, butter, cream, lard), and trans-fatty acids from hydrogenated vegetable oils (baked goods and some margarines) (Miettinen et al. 1972; Baer et al. 2004). Fats with a greater protective effect include monounsaturated and polyunsaturated fats found in olive oil and oily fish (Bucher 2002).
While most fatty acids can be synthesised within the body, there are two that are essential. The essential fatty acids are linolenic (omega-3) and linoleic (omega-6) fatty acids. Omega-3 fatty acids, in particular, are associated with beneficial effects on the cardio-vascular system (Simopoulos 1999). Linolenic fatty acids are found in oily fish and a range of vegetable oils such as sunflower and sesame. Linoleic fatty acids are found in most plant oils, especially corn and soybean oil.
Current guidelines for fat intake in a healthy diet recommend no more than 30% of energy intake as total fat, and less than 10% as saturated fat (DoH 1991, 1994).
Athletes should aim to avoid or reduce their intake of the following high fat foods: cake, biscuits, chocolate, fat on meat, sausages, pasties, pies, beef burgers, cheese, butter and cream. Moreover, athletes should aim to get the majority of their fat intake from oily fish, white fish, vegetable seeds and oils, soya beans and nuts.
Vitamins
Vitamins are essential organic molecules that cannot be synthesised in the body. Although they are only required in very small quantities, a deficiency can lead to symptoms of disease. Vitamins have a range of specific functions that are essential for health. These include: absorption of nutrients, anti-oxidants and protection of cell membranes, energy metabolism, catalysts for and regulation of chemical reactions and collagen synthesis. There are 13 compounds commonly identified as vitamins that are broadly categorised as either water-soluble or fat-soluble. A summary of the body’s requirements of these nutrients is provided in Table 14.1.
Table 14.1 Reference nutrient intakes for healthy adults
Vitamin | RNI (adult male) | RNI (adult female) |
A | 700μg | 600μg |
Thiamin (B1) | 1.0mg | 0.8mg |
Riboflavin (B2) | 1.3mg | 1.1mg |
Niacin (B3) | 1.7mg | 1.3mg |
Pyridoxine (B6) | 1.4mg | 1.2mg |
B12 | 1.5mg | 1.5mg |
Folic acid | 200mg | 200mg |
C | 40mg | 40mg |
D | 10μg (if limited exposure to sunlight) | 10μg (if limited exposure to sunlight) |
E* | RNI not established | |
K* | RNI not established | |
Adapted from DoH 1991 – requirements vary for children and pregnant women. |
Water-soluble vitamins include vitamin C and the B-complex. They cannot be stored within the body and must therefore be consumed regularly. Any excess intake is normally excreted in the urine.
Vitamin B refers to a host of different vitamins that play an essential role in the release of energy from carbohydrates, fat and protein, and the formation of haemoglobin. They are known as the B-complex and include thiamine (B1), riboflavin (B2), pantothenic acid, niacin (B3), piridoxine (B6), folic acid, cyanocobalamin (B12), and biotin. Deficiencies can lead to fatigue, gastro-intestinal problems, heart failure and nervous disorders (FSA 2008). Vitamin B is found in grains, milk products, eggs, green vegetables, fish, liver, nuts and wholegrain and fortified cereals.
Vitamin C (ascorbic acid) works as an antioxidant and is essential for collagen synthesis, protein metabolism, wound healing, functioning of the immune system and iron absorption. Deficiencies can lead to poor immune function, poor wound healing, and scurvy. Good sources of Vitamin C include citrus fruits, tomatoes and green vegetables.
Fat-soluble vitamins include vitamins A, D, E, and K. These are stored in the body and can accumulate in fatty tissue. Excessive intakes may accumulate to toxic levels.
Vitamin A (retinol and beta-carotene) has many roles. It is an important anti-oxidant, and is essential for vision, immune function, bone health and gene transcription. Deficiency leads to vision impairments, particularly night blindness. Excess can become toxic and lead to numerous problems that could include birth defects, kidney problems, nausea, hair loss, headache, irritability, susceptibility to infection, fissures of the lips, blurred vision, bone and joint pains, muscle pain and weakness (FSA 2003). Whilst it is not possible to establish a Safe Upper Limit for vitamin A, total intakes above 1500 micrograms should be avoided (FSA 2003). Good sources of vitamin A include liver, kidney, milk, and eggs. Good sources of beta-carotene (a precursor to vitamin A) include green leafy vegetables and carrots.
Vitamin D plays a central role in the absorption and regulation of both calcium and phosphorus and is therefore essential for optimum bone health. Deficiency can lead to rickets, osteomalacia, and osteoporosis. Vitamin D is synthesised by the skin in response to UV exposure but can also be gained from foods such as fish, eggs, fortified dairy products and breakfast cereals.
Vitamin E refers to a group of antioxidants that prevent cell-membrane damage by reacting with radicals produced by lipid peroxidation. Vitamin E is found in seeds, nuts, green leafy vegetables, avocado, olives and vegetable oils.
Vitamin K is primarily responsible for blood clotting. A deficiency is associated with severe and uncontrolled bleeding, malformation of developing bone and cartilage calcification. The main dietary sources include green leafy vegetables, avocado, and kiwifruit.
For those following a varied and balanced diet, deficiencies in the fat-soluble vitamins are rare.
Minerals
Minerals have several major roles including the formation of bones and teeth, formation of haemoglobin and hormones, muscular contractility, neural conductivity, regulation of acid-base balance, and metabolism. Some minerals occur in the body in relatively large amounts. These are known as the major minerals and include: calcium, phosphorus, sulphur, potassium, chlorine, sodium, magnesium, zinc and iron. Other minerals occur in minute quantities. These are called trace minerals and include: chromium, cobalt, copper, fluorine, iodine, manganese, molybdenum, nickel, selenium, silicon, tin and vanadium (see Table 14.2).
Table 14.2 Summary of the functions and sources of key minerals
Mineral | Function | Foods |
Boron | Promotes healthy bones, teeth; metabolism of other minerals | Fruits and vegetables |
Calcium | Blood clotting, intracellular signaling, muscle contraction | Milk, yogurt, cheese, other dairy products, sardines, bread, sultanas, vegetables |
Chromium | Insulin and glucose tolerance responses | Meat, wholegrain cereals, legumes and nuts |
Cobalt | Contained in vitamin B12 | Meat, dairy products, eggs |
Copper | Formation of haemoglobin; absorption and use of iron; skin, hair pigmentation | Shellfish, liver, meat, cereal products, vegetables |
Fluoride | Prevents dental carries; crystalline structure of bones and teeth | Water (variable), tea, seafood, |
Iodine | Contained in hormones thyroxine (T4) and triiodothyronine (T3) | Seafood, vegetables and cereals |
Iron | Contained in cytochromes, myoglobin, haemoglobin. | Beef, liver, eggs, sardines, apricots, fortified cereals, plain chocolate, bread, vegetables |
Magnesium | Mineral present mainly in the bones; maintains electrical potential in nerve and muscle cells | Cheese, milk, chicken, cod, peanuts, bread, marmite, cereal products, potatoes, vegetables |
Phosphorus | Contained in bones, teeth; role in energy metabolism | Cheese, eggs, milk, chicken, beef, ham, peanuts, marmite, meat and bread |
Potassium | Role in fluid and electrolyte balance; heart muscle activity; metabolism and protein synthesis | Fruit and vegetables, fruit juices, milk, fish, meat |
Selenium | Helps heart function; possibly prevents certain cancers | Meat, fish and cereal products |
Sodium | Present in extracellular fluid | Table salt, bacon, ham, potato crisps, cereals, marmite, soy sauce |
Sulphur | Energy metabolism, enzyme function, and detoxification | Cheese, eggs, nuts, onions, green leafy vegetables, fish, wheat germ |
Zinc | Contained in enzymes, transcription factors | Meat and meat products, bread, cheese and eggs, milk, cereal products |
Deficiencies of any mineral can lead to signs and symptoms of disease. Moderate excesses of sodium, potassium, calcium and chlorine are normally excreted by the kidneys, whereas excess intakes of other minerals can be harmful or impair absorption of other micronutrients (e.g. excess calcium inhibits absorption of iron and zinc; excess zinc inhibits absorption of copper) (Fairweather-Tait and Hurrell 1996).
In general, a balanced diet that contains a variety of foodstuffs and includes plenty of fresh fruit and vegetables should provide sufficient quantities of the minerals required for optimal health.
Two of the most important minerals for athletes are iron and calcium.
Iron is an essential element, which is required for oxygen transport (haemoglobin and myoglobin) and electron transport. It is found in the diet in two forms, haem iron and non-haem iron. Haem iron is generally found in animal foods and has greater bioavailability (10–35%). Non-haem iron, generally found in plant foods, has low bioavailability (2–10%) (Zijp et al. 2000).
Low iron levels can be due to inadequate diet, malabsorption or increased iron losses. To ensure an adequate iron intake, athletes should eat a variety of foods including red meat and green leafy vegetables. Iron absorption can be enhanced by the presence of vitamin C. Excessive intak es of iron are associated with gastrointestinal disorders including constipation, nausea, vomiting and diarrhoea, whilst chronic excess may lead to high body iron stores and increased risk of cardiovascular disease or cancer (FSA 2003). The FSA Expert Group on Vitamins and Minerals (2003) suggest that, for most people, an intake of approximately 17 mg/day would not be expected to produce adverse effects.
Calcium is another essential mineral for health and sports performance. It forms an integral part of bone structure, muscle contraction, blood clotting and transmission of nerve impulses. A deficiency can lead to rickets, reduced bone mineral density and osteoporosis, and problems with blood clotting.
The best dietary sources of calcium are milk and milk products, and green leafy vegetables. Overall absorption is generally poor and requires adequate vitamin D (FSA 2003).
Summary of micronutrients
Overall, a diet that is balanced and varied should meet the needs for vitamins and minerals for a healthy population. In athletes, the question of whether vitamins and minerals are required in greater amounts is more complex, and likely to be affected by the interaction between the total volume and intensity of sporting activity and the specific energy and nutrient intakes of each individual (Volpe 2006). It does appear, however, that a deficiency will have a negative impact on performance (Volpe 2006).
Water
Water is by far the most important nutrient. It accounts for 50–75% of body weight, depending on body fat content, with fat tissue containing approximately 20% water, lean tissue approximately 75%, and blood around 95%. Water has many crucial roles including temperature control (sweating), lubrication (brain, eyes, spinal cord and digestive system), a solvent for all biochemical reactions, and the transportation of nutrients and oxygen to, and carbon dioxide and other metabolic waste products away from, the working muscles. For optimal cardiovascular and thermoregulatory function athletes must therefore maintain sufficient body fluid levels and avoid dehydration, which results in a decrease in both cardiovascular (Gonzalez-Alonso et al. 1997) and strength and power performance (Jones et al. 2008). Water is gained from drink, food and metabolic reactions and is lost through urine, faeces, expired air and sweat.
Alcohol
Alcohol is a non-essential nutrient that has a range of negative effects for athletes. It causes intoxication, affects skill and co-ordination, leads to dehydration, de-motivates, inhibits recovery, lowers testosterone and provides an energy source (7kcal/gram) that can only be utilised once it has been deposited as fat. Overall, alcohol is highly detrimental to sporting performance, significantly reducing aerobic performance capacity for several hours after consumption (O’Brien and Lyons 2000; El-Sayed et al. 2005). Alcohol also acts as a diuretic and therefore may result in dehydration.
The importance of a balanced diet
For optimal health and performance it is essential that athletes get the right quantities and balance of all the nutrients. There are several tools and guidelines to ensure the correct quantities and balance of nutrients for health. The most common tool is the reference nutrient intake (RNI), which is the amount of a nutrient required to maintain adequate health for the majority of the population. RNIs are provided for energy, protein, vitamins and minerals. The advantages of using RNIs are that they give individuals a starting point to judge their diet in relation to a healthy population. RNIs, however, do not take into account the individual needs of athletes determined by the individual, the sport and the level of training. For athletes, a diet that is adequate for health may not be optimal for performance, due primarily to an increased energy expenditure.
The current UK government guidelines for health suggest a balance of approximately 55% carbohydrate, 15% protein and less than 30% fat (of which saturated fat should account for less than 10%) (FSA 2008). Again, these recommendations act as a good starting point for athletes but, as discussed later, there are a number of circumstances where these recommendations are modified. The optimal diet for an athlete will depend on the sport, activity levels, body size, weight gain or weight loss, and the type of training performed at any given time.
The food guide pyramid (Figure 14.2) is a common tool to help individuals achieve a healthy balance of foods within the diet. The pyramid shows the proportion and type of foods, which contribute to a healthy, balanced diet. At the base of the pyramid are carbohydrate-based foods, and requirements for fruit and vegetables. The pyramid reflects the percentage guidelines already outlined for the macronutrients in a healthy diet. Several alternative versions of the pyramid now exist to support specific groups (Painter et al. 2002).
Nutrition for performance
Optimal nutrition for performance is determined by what, when and how much an athlete eats. A diet that is adequate for health is not usually optimal for performance. For example, athletes will require more energy, greater quantities of certain nutrients (carbohydrates, protein) and will need to consume food at key times before, during and after training or competition.
Energy requirements for specific sports/training activities
Energy requirements for athletes are determined by their size, basal metabolic rate, the extra energy that is required for activities performed throughout the day (training and competition), recovery and adaptation from training and competition and whether the athlete is trying to gain or lose weight.
Mean total daily energy expenditures vary between different athletes. For example:
- male boxers (57Kg) expend 2900kcal; male weightlifters (110Kg), 4900kcal; female basketball players (61.4Kg), 3100kcal (Ismail et al. 1997).
- male cross-country skiers (during periods of hard training) use around 8600kcal/day (Sjodin et al. 1994).
- professional road cyclists expend over 6000kcal per day throughout the three-week Tour de France event, and use in excess of 9000kcal per day during hard mountain stages (Saris et al. 1989). This is reflected by high reported energy intakes (in excess of 5450 Kcal) during training and competition (García-Rovés et al. 2000).
In team sports, differences in activity levels will also vary with playing position, resulting in large variations in energy expenditure between individuals on the same team, which must be matched by energy intake. For example, Lundy et al. (2006) report that elite professional rugby league players consume between 2700 and 6900 kcal/day (mean 4230kcal/day) depending on size and position, although it is worth noting that this is ‘normative’ data and this level of consumption may not necessarily be optimal. In fact, Lundy et al. (2006) recommended that these athletes increase their energy intake through additional carbohydrate consumption.
Calculating energy needs
Energy needs can be calculated by the following formula:
PAL refers to the ratio of total energy required over 24 hours to the BMR over 24 hours. For example a PAL of 1.4 represents very low activity levels, 1.6 represents moderate activity levels, and 1.9 represents high activity levels. Most athletes will have a PAL of 1.9 or above.
Macronutrient requirements for performance
Energy needs are met from carbohydrate, fat and protein. The balance of use between these fuels, at any one time, depends on the intensity and duration of exercise. At the exercise intensities normally encountered during training and competition, carbohydrate is usually the primary energy substrate. To optimise performance athletes need to consume the right fuels at the right time.
Carbohydrate requirements for performance
The selection of fuel for muscular work is directly related to the intensity of effort with the higher the intensity, the greater the reliance on carbohydrate as a substrate (see Figure 14.3) (Romjin et al. 1993). As most sports are performed at high intensities carbohydrate becomes the primary source of energy.
Figure 14.3 Contribution of muscle glycogen, plasma glucose, plasma FFA, and muscle triglyceride to energy expenditure after 30 min of exercise at 25%, 65%, and 85% of maximal oxygen uptake in fasted subjects (from Romjin et al. 1993).
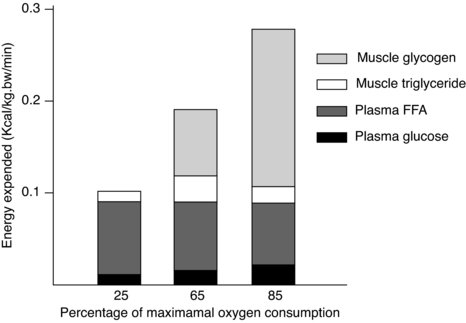
In the body, carbohydrate is stored primarily as muscle and liver glycogen. Carbohydrate stores, however, are limited to approximately 450g of muscle glycogen (with a range from 50g after exhaustive exercise to approximately 900g in a large, well-trained, well-rested, and well-fed athlete (Jeukendrup 2003, de Jonge and Smith 2008), 100–120g of liver glycogen, and 5g of circulating blood glucose. Just 2–3 hours of high-intensity activity may be enough to completely deplete these relatively limited glycogen reserves (Coyle et al. 1986). The status of the glycogen stores before activity, therefore, will determine the duration that high intensity exercise can be maintained (Astrand and Rodahl 1986). It is therefore important that, for endurance activities (Coyle et al. 1986) and high-intensity intermittent activities (Balsom et al. 1999), athletes aim to optimise their glycogen stores. Depending on the intensity and volume of training, this is normally achieved with an intake of 5–13g/kg.bw/day(see Table 14.4) (Jeukendrup and Gleeson 2004).
Pre-exercise
To delay fatigue, and therefore enhance performance, athletes should optimise muscle glycogen stores pre-exercise (Astrand and Rodahl 1986). In short, glycogen stores must be sufficient to allow completion of the event at the highest possible intensity. It is important to distinguish between maximal and optimal storage of glycogen. For events where glycogen depletion becomes a limiting factor for performance (e.g. the marathon, a soccer match) it is clearly desirable to maximise stores prior to the race or game. For shorter events, for example, a 10K race, maximising glycogen stores may not be desirable. Every gram of glycogen is stored with approximately 2.7 grams of water so any additional weight from excess glycogen storage has the potential to inhibit performance. Athletes should therefore only seek to ‘carbo-load’ for sports where glycogen depletion may become a limiting factor in performance.
Carbohydrate-loading
Athletes can maximise muscle glycogen stores through a carbohydrate loading regime (see Table 14.3). There are several protocols for doing this. Early research suggested athletes should perform an exhaustive bout or bouts of exercise followed by 3-4 days of very low carbohydrate intake or very low carbohydrate/high fat intake, followed finally by 3-4 days of extremely high carbohydrate intake (Ahlborg et al. 1967; Bergstrom et al. 1967). Although this did increase muscle glycogen stores, athletes were reluctant to train so hard and felt lethargic and irritable just a few days before competition. A later and more widely adopted protocol involved tapering training over a seven day period and increasing consumption of carbohydrate for three days before the race (Sherman et al. 1981). The advantage of this method was that athletes benefited from increased glycogen stores without the negative side effects. Most glycogen loading protocols used by athletes and researchers involve the Sherman et al. (1981) protocol, or variations thereof (Madsen et al. 1990; Widrick et al., 1993; Burke et al. 2000; James et al. 2001). More recently even higher glycogen levels have been achieved by the inclusion of a 3-minute intense bout of training (150s of cycling at 130% of VO2 peak followed by 30s of all-out cycling) 24-hours before the event followed by 10.3g/kg.bw/day of high-glycaemic index carbohydrates (Fairchild et al. 2002). The main advantage of this protocol is not that higher glycogen levels are reached, but that they are achieved in a much shorter time-frame (24 hours) and do not necessarily require an extended period of tapering. Whilst there may be obvious advantages to athletes that compete on a regular basis – for example, team sport athletes – there is, as yet, little evidence to support the use of this method in a practical setting over Sherman et al. (1981), particularly as the potential negative effects of the 3-minute intense bout 24-hours before competition are not yet fully realised.
Table 14.3 Suggested carbo-loading protocols for (1) an endurance event, and (2) a weekly match (for a team sports player)
Endurance event | |
Days before the event | Action |
7–10 days before the event | Start to taper training |
6–4 days before | Consume 7g/kg.bw/day |
From 3 days before | Consume 10–13g/kg.bw/day |
Weekly match | |
From 2 days before the match | Taper training |
Days 7–6 before the next match | To facilitate recovery from the previous match – consume 10g/kg.bw/day |
Days 5–3 before the next match | To maintain stores during training – consume 7–10/kg.bw/day |
From 2 days before the match | To ensure optimal levels of muscle glycogen prior to the next game – consume 10g/kg.bw/day |
Table 14.4 Summary and practical carbohydrate intake recommendations to replenish muscle glycogen during training
Volume/intensity of training | Approximate duration | Daily carbohydrate requirementsa |
Moderate training | 1–2 hours | 5–7g/kg.bw/day |
Heavy training | 2–3 hours | 7–10g/kg.bw/day |
Very heavy training | Over 3 hours | 10–13/kg.bw/day |
aFrom Jeukendrup and Gleeson (2004). |
Liver glycogen stores in particular are sensitive to dietary intake of carbohydrate and can be depleted by the overnight fast. To replenish liver glycogen stores, athletes should eat breakfast and/or consume a pre-competition meal. For example, consume 150–300g of moderate GI carbohydrate three to four hours beforehand. To maintain blood glucose, smaller amounts of carbohydrate (e.g. <50g, of low-moderate GI) can then be consumed in the hour prior to exercise.
Fructose (the sugar often found in fruit, honey and commercial sports drinks) has been suggested as a source of carbohydrate immediately prior to exercise as the insulin response is lower than with glucose (Maughan et al. 1997), however, for many athletes fructose can lead to gastrointestinal discomfort (Murray et al. 1989; Beyer et al. 2005).
During exercise
Whilst the benefits of pre-competition (or pre-training) carbohydrate loading are well established, there is also clear evidence for an ergogenic effect of carbohydrate feeding during an event (Coggan and Coyle 1991). Rather than reducing the rate of glycogen utilisation (Bergstrom et al. 1967; Tsintzas et al. 1996), carbohydrate ingestion appears to maintain blood glucose levels late in exercise, thus maintaining carbohydrate oxidation, and therefore performance, during endurance exercise (Coyle et al., 1986, Coggan and Coyle, 1991). Carbohydrate ingestion also improves endurance performance during intermittent high-intensity running in athletes with already high pre-exercise muscle glycogen concentrations (Foskett et al. 2008), and should therefore be considered an essential practice for team sport athletes.
Carbohydrate feeding during exercise is often best achieved through the use of sports drinks or carbohydrate gels. These are palatable, convenient to take, and generally tolerated well by athletes. Sports drinks typically contain around 60g of carbohydrate per litre, which is close to the maximum amount of exogenous carbohydrate that the body can absorb and oxidise per hour (1-1.1g/min) (Jeukendrup and Jentjens 2000; Wallis et al. 2007; Jeukendrup, 2008). For longer events, athletes can carry small snacks of easily digested high-carbohydrate foods (e.g. sports gels, jelly-beans, jam sandwiches). To identify what they like and what they can tolerate, athletes should practice eating and drinking during training. Depending on the priority (fluid delivery or carbohydrate delivery) athletes can vary the concentration of carbohydrate in the sports drink. For example, a 5% solution will be more appropriate for those athletes wishing to replenish both water and carbohydrate. A 10% solution will be more suited to carbohydrate delivery at the expense of gastric emptying (Maughan 1991) however, during exercise this may result in gastrointestinal distress in some individuals.
It is often difficult for team sport athletes to consume food or fluid during the game. Players and support teams must be organised and proactive during any stoppages, time-outs, injury breaks, and half-times as these breaks provide the perfect opportunity to consume an isotonic carbohydrate-based drink.
Post-exercise
To enhance recovery and provide the fuel for the next match or training session, athletes must restore their muscle glycogen stores as soon as possible post-exercise. If glycogen stores are low at the start of the next training session, muscle glycogen and overall carbohydrate utilisation will be reduced, lowering the exercise intensity that can be maintained.
The rate and extent of glycogen re-synthesis is dependent on the quantity, timing and type of carbohydrate ingestion, and on the nature of recovery (Maughan et al. 1997).
How much?
Glycogen re-synthesis depends on the quantity of dietary carbohydrate consumed. To replenish glycogen stores athletes should consume at least 1g/kg.bw immediately post-exercise (Ivy 1998) or a minimum of 1–1.85g/kg.bw of carbohydrate per hour in the first few hours post-exercise (Jentjens and Jeukendrup 2003) and maintain a daily intake of 5–7g/kg.bw/day during periods of moderate training (1–2 hours per day), 7–10g/kg.bw/day when training load is increased (2–3 hours per day), and 10–13g per kg/day during periods of hard or prolonged training (over 3 hours per day) (Jeukendrup and Gleeson 2004).
When?
Due to elevated enzyme activity (glycogen synthase) (Sherman et al. 1983; Doyle et al. 1993) and cell membrane permeability, the highest rates of muscle glycogen synthesis occur when athletes consume large amounts of carbohydrate (1.0–1.85 g/kg.bw/h) immediately post-exercise, followed by further feeding at 15–60 minute intervals thereafter for up to 6-hours post-exercise (Ivy 1998; Jentjens and Jeukendrup 2003). When carbohydrate feeding is delayed, lower rates of muscle glycogen synthesis will occur (Jentjens and Jeukendrup 2003). Therefore, to facilitate glycogen resynthesis, athletes should consume carbohydrates as soon as possible post-exercise.
What?
High glycaemic index snacks (eg. jaffa cakes, jelly beans, toast and jam) and, in particular, carbohydrate based drinks are convenient, easily and more rapidly absorbed and digested, and will provide sufficient carbohydrate until consumption of a larger meal. In order to maintain the high level of carbohydrate intake and glycogen resynthesis, the post-exercise meal should be based around complex carbohydrates such as pasta, rice or potatoes.
Type of recovery
Passive recovery appears to be a more effective strategy to optimise glycogen resynthesis, particularly in type I muscle fibres (Choi et al. 1994; Fairchild et al. 2003).
Carbohydrates and protein
Although some studies have shown enhanced glycogen resynthesis when carbohydrate feedings are combined with protein, as long as the carbohydrate feedings are adequate, and meet the recommendations, there appears to be no additional benefit on glycogen resynthesis or performance when protein is taken as well (Jentjens et al. 2001; Osterberg et al. 2008). Despite this there may be some additional benefit in terms of protein synthesis and muscle regeneration when both protein (10–20g) and carbohydrate (60–90g) are combined post-exercise (Griewe et al. 2001; Bolster et al. 2004; Dreyer et al. 2008).
Summary/Key points
- Athletes should aim to optimise glycogen levels pre-exercise via breakfast and a pre-exercise meal (low-moderate GI carbohydrates). See table below for examples.
- During exercise consume up to 70g/hour to maintain carbohydrate oxidation and exercise intensity (sports drinks, easily digestible snacks, see the table below for examples).
- Consume high glycaemic index and easily digested carbohydrates immediately post-exercise (1-1.85g/kg.bw).
- Consume 1g/kg.bw high glycaemic index carbohydrates within the next 2 hours post exercise.
Table 14.5 provides examples of carbohydrate-rich foods ideal for pre-, during and post-exercise.
Table 14.5 Example carbohydrate rich foods (providing approx. 50g carbohydrate per portion) for pre-, during-, and post-exercise
Pre | During | Post |
1 large bowl of cereal with milk – cornflakes, Frosties, Shreddies, branflakes, 3–4 Weetabix or Shredded Wheat | 750ml sports drink | 2 slices toast/bread with jam or honey |
1 cup of soup and a large bread roll | 1–2 gels or sports bars | 2–3 crumpets with jam or banana |
500ml fruit juice | 500ml fruit juice | 500ml fruit juice |
¾ can of baked beans on 2 slices of toast | 75g jelly babies | ¾ can of baked beans on 2 slices of toast |
1 bagel or 2 bread rolls with filling | 6 jaffa cakes | 250ml milkshake or fruit smoothie |
2 slices malt loaf / 2 cereal bars | 2 slices malt loaf/2 cereal bars | |
1 large baked potato | 300g mashed potato | |
1 packet dried fruit | 1/3 pizza with topping | |
1 bowl fruit salad with pot of fruit flavoured yogurt | 1 can of rice pudding | |
2 bananas | 6 jaffa cakes/1 mars bar | |
1 large currant bun |
Fat as a fuel for exercise
Whilst high-intensity exercise is fuelled predominantly by carbohydrate, at lower intensities, or late in prolonged exercise, fat becomes increasingly important (Klein et al. 1994; Horowitz and Klein 2000). In contrast to limited carbohydrate stores, fat stores are large, with an average person storing over 100,000 kcal of energy as fat, mainly as subcutaneous adipose tissue. Due to limits in the fat oxidation process, however, these stores cannot be fully utilised for exercise.
There are several steps involved in the oxidation of fat for energy:
1. lipolysis, where stored fat must be broken down to fatty acids for transport around the body
2. transport of fatty acids in the blood stream to the muscle cell
3. transport of fatty acids across the cell membrane and to the mitochondria for oxidation.
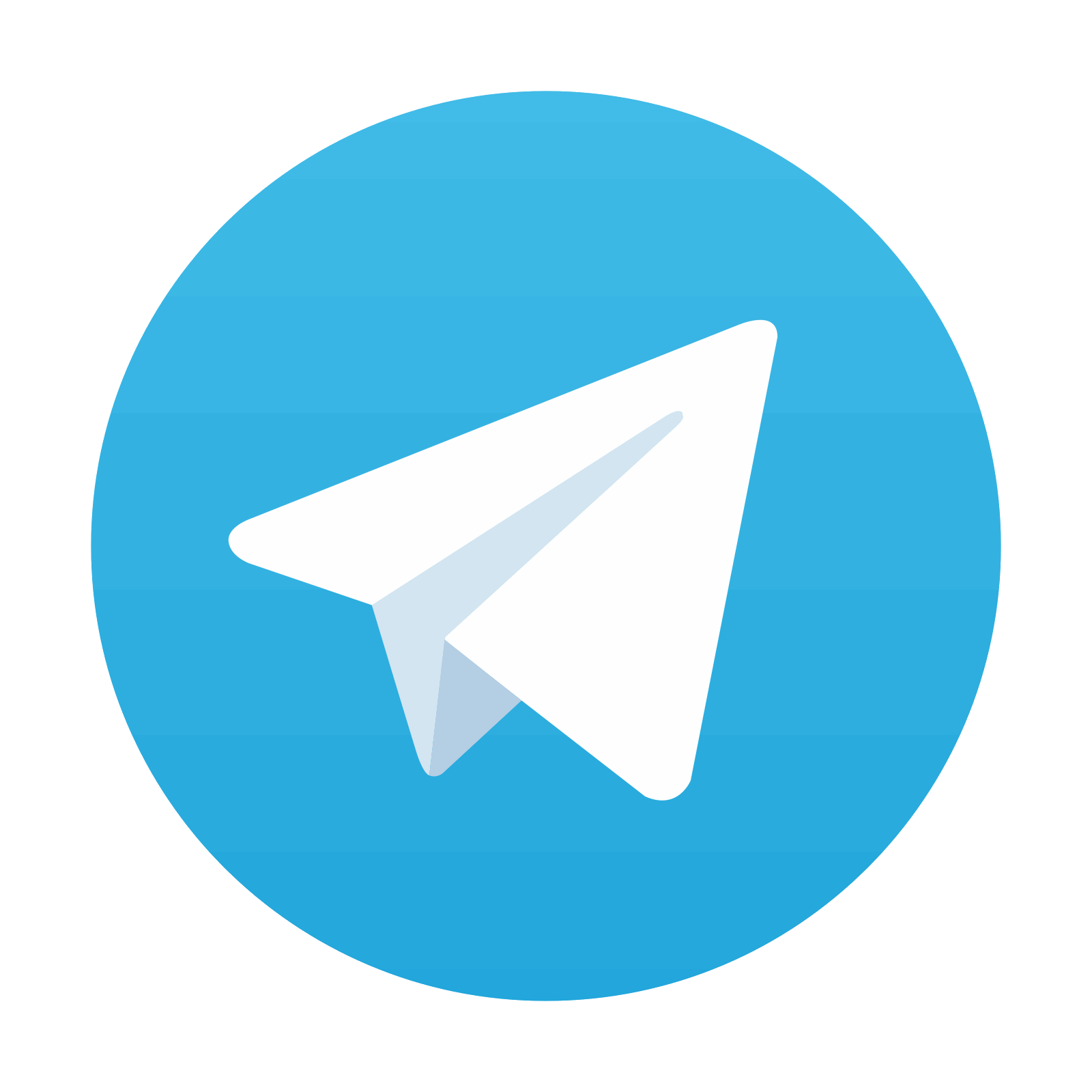
Stay updated, free articles. Join our Telegram channel

Full access? Get Clinical Tree
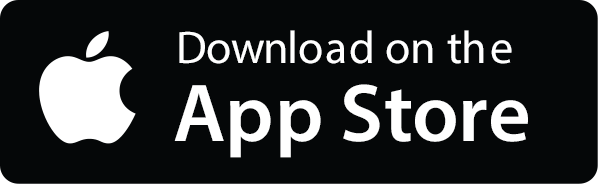
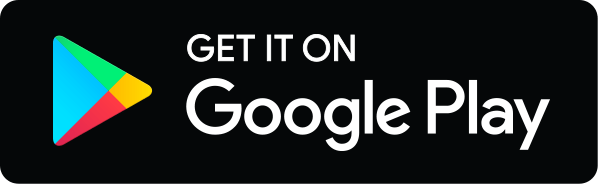