41 Key Points 1. The presence of hematoma at injury level on MRI is generally associated with the worst outcomes. 2. Significantly decreased fractional anisotropy (FA) values occur at injury levels in patients with acute cervical spine cord injury compared with normal volunteers. Tractography may be used to visualize residual tracts after injury in the near future. 3. Functional MRI studies of the brain show significant reorganizational changes in the sensorimotor cortex after spinal cord injury. Magnetic resonance imaging (MRI) is essential in the evaluation and management of spinal cord injury (SCI) and is considered the imaging modality of choice for identification of ligamentous injury, hematoma, disk compression, spinal cord edema, and myelomalacia. This chapter reviews the role of MRI in the evaluation of injury severity and prediction of recovery, with a focus on identification of parameters that have been found to be useful markers of injury severity. Chapter 3 discusses the clinical use of MRI in the determination of injury characteristics and classification and for the initial treatment planning for patients in the emergency room. This chapter focuses on identifying findings on conventional MRI that can be helpful in predicting outcome. It also describes emerging MRI technologies not currently routinely used in clinical care but likely to find utility in the near future. The pattern of MRI findings has been of great interest for assessment of injury severity and prognosis. Table 41.1 lists the most common qualitative MRI findings shown in peer-reviewed studies to correlate with injury severity and recovery.1–4 Lammertse et al.5 provide a systematic review. The presence of hematoma is generally associated with the worst outcomes.1,6–9 Traumatic spinal cord hematoma is best identified as focal decreased signal on T2-weighted (Fig. 41.1) or gradient echo images. Quantitative parameters shown to adversely impact recovery are the length of cord edema or signal change,2,3 extent or size of hematoma,1,4 and degree of spinal cord compression.3 The degree of spinal cord compression can be calculated in two ways10: The maximal canal compromise (MCC) and maximal spinal cord compression (MSCC) are defined by the following equations3,10: Table 41.1 Potential Predictive Markers in Spinal Cord Injury
Neuroimaging after Spinal Cord Injury: Evaluating Injury Severity and Prognosis Using Magnetic Resonance Imaging
Conventional Magnetic Resonance Imaging for Spinal Cord Injury
Qualitative MRI predictors |
• Presence of hematoma • Edema or signal change on T2-weighted images • Cord compression • Cord transection |
Quantitative MRI predictors |
• Maximal canal compromise • Maximal spinal cord compression • Length of edema • Length of hematoma |
Other potential predictors |
• Fractional anisotropy in diffusion tensor imaging studies • Tractography • N-acetylaspartate and N-acetylaspartate:creatine ratios on magnetic resonance spectroscopy studies |
Di represents the anteroposterior canal diameter at the level of maximum injury, Da is the anteroposterior canal diameter at the nearest normal level above the site of injury, and Db is the anteroposterior canal diameter at the nearest normal level below the site of injury; di is the anteroposterior spinal cord diameter at the level of maximum injury, da is the anteroposterior spinal cord diameter at the nearest normal level above the site of injury, and db is the anteroposterior spinal cord diameter at the nearest normal level below the site of injury. The use of the MCC and MSCC has been shown to have good interrater reliability and to be predictive of severity of injury.3,10 Figure 41.2 is an MRI scan of a 41-year-old patient with a C6-7 fracture dislocation SCI and illustrates severe spinal cord compression and canal compromise at the injury level.
Fig. 41.1 A 23-year-old female status post–motor vehicle accident with T3–5 spinal cord injury. Sagittal fast spin echo T2-weighted image (left) and sagittal short T1-weighted inversion recovery (STIR) images. The low signal in the middle of the cord (orange arrow) is hematoma. There is also significant edema (blue arrows).
Over all, MRI appears to be of tremendous value in augmenting the clinical exam and American Spinal Injury Association (ASIA) scale and motor scores, which are considered to be the best predictors of neurological recovery.7,11 Attention to details on the MRI will provide further information on prognostication in patients with the same ASIA grade or motor score. The main limitation of MRI in SCI prognostication is its inability to identify spared white matter tracts. This disadvantage may be overcome with the development of diffusion tensor imaging (DTI) technology.
Diffusion Tensor Imaging
DTI is a relatively new imaging modality that can permit the imaging of directional asymmetry in the diffusion of water protons to make maps of white matter fiber bundles. This is an established technique in the brain, and early reports suggest that it is likely to be of significant use in the evaluation of SCI and diseases. DTI has potentially two main uses in SCI. The first is tractography, which is a technique to visualize the white matter tracts and therefore is of interest in identifying residual pathways after injury.12 A second area of interest is generation of quantitative parameters with prognostication value. The fractional anisotropy is one of several quantitative parameters that are mathematically derived from the eigenvalues of the diffusion tensor. The role of quantitative parameters like the fractional anisotropy in predicting injury severity and prognosis is the subject of intense research. The fractional anisotropy is a value between 0 (isotropic or unrestricted diffusion along all axes) and 1 (restricted diffusion along a single axis). It is a measure of white matter axonal diameter, myelination, and fiber density. It can be calculated using the following formulas:
where and (λ1, λ2, λ3) are the eigenvalues of the diffusion tensor.
Fig. 41.2 A 41-year-old patient status post–motor vehicle accident with a C6–7 fracture dislocation injury. Sagittal fast spin echo T2 (left image) and sagittal short T1-weighted inversion recovery (STIR) showing significant dislocation, cord compression, and severe canal compromise at C6–7 (white bars).
Ellingson et al.13 performed DTI in 13 neurologically intact subjects and 10 subjects with chronic SCI (> 4 years postinjury). They found that the fractional anisotropy was significantly lower at the chronic lesion site, and the extent of reduction in FA appeared dependent on the completeness of the injury. Similarly, Shanmuganathan et al.14 reported significantly decreased lesional FA values in 20 symptomatic patients with acute cervical spine trauma compared with normal volunteers. To date there have been no studies of correlations between FA values and clinical scores in acute SCI patients, and a central role of DTI for injury prognostication is premature at the present time. Figure 41.3 illustrates a DTI study in a patient with a 9 year history of C7 ASIA B chronic SCI showing significantly reduced fractional anisotropy at the injury site. The corresponding tractography images illustrate few fibers below the injury site. There was no recovery of lower extremity motor function.
Fig. 41.3 A 61-year-old patient with a C7 American Spinal Injury Association (ASIA) B injury from a motor vehicle accident in 2000. (A) Sagittal T2-weighted image showing focal C7 signal change and myelomalacia. (B) Tractography images show few fibers (red and blue) below the lesion site. Diffusion tensor images are shown: ADC (C, left) and fractional anisotropy (C, right) are shown. There is significantly reduced fractional anisotropy at the C7 injury site.
Magnetic Resonance Spectroscopy
Magnetic resonance spectroscopy is commonly used to provide cellular and metabolic information in the brain and has proven useful in the differential diagnosis of intracranial pathology. Typical spectral peaks of interest are the N-acetylaspartate (NAA), choline (Cho), creatine (Cr), and lactate.15 NAA is considered a marker of neuronal density and loss. Decreases in NAA are reflective of axonal loss. Creatine is a marker of energy metabolism. Its peak is stable under a variety of pathological conditions and is therefore used as an internal control for calculating metabolite ratios, such as Cho:Cr and NAA:Cr ratios. Cho is a metabolic marker of membrane density and integrity and is influenced by phospholipid synthesis and degradation and cellular turnover. Lactate levels increase significantly under conditions of anaerobic metabolism, as in failure of aerobic oxidation during ischemia.
Recently, the technique has been applied in the evaluation of cervical spondylotic myelopathy by Holly et al.16 They performed magnetic resonance spectroscopy (MRS) in 21 patients with clinical and radiographic evidence of cervical spondylotic myelopathy (CSM). The patients underwent preoperative neurological examination, functional assessment, and cervical spine MRS. MRS spectra peaks for NAA, Cho, lactate (Lac), and Cr were measured using voxels placed at the C2 level. Thirteen age-matched healthy volunteers served as controls. The NAA:Cr ratio was significantly lower in patients with CSM than in controls. The Cho:Cr ratio was not significantly different between the two groups. Seven of the patients with CSM had a Lac peak, whereas no peaks were noted in the control group (p < 0.05). The authors concluded that the reduced NAA:Cr was likely due to axonal and neuronal loss, whereas the presence of Lac peaks in a third of the patients was supportive of the role of ischemia. Other studies have documented reduced NAA concentrations in multiple sclerosis patients.17 Further studies are needed to establish a prognostic role for MRS in acute SCI.
Functional Magnetic Resonance Imaging
Functional magnetic resonance imaging (fMRI) techniques can be used to measure the pattern and extent of activation in brain regions in response to motor and sensory tasks. Recently, several groups have performed fMRI of the spinal cord. Studies in healthy volunteers show activation patterns corresponding to anatomical location of the activated myotome, with a signal intensity proportional to the intensity of effort.18–20 Stroman et al.18 performed fMRI of the spinal cord in 27 volunteers with cervical or thoracic SCIs. Of these volunteers, 18 had complete injuries, and nine had incomplete injuries. Thermal stimulation at 10°C was applied to the fourth lumbar dermatome on each leg, and images were obtained of the entire lumbar spinal cord. Areas of neuronal activity were consistently observed in the lumbar spinal cord in response to the thermal stimulation, even when the subjects had no awareness of the sensation. Patients with complete SCI showed absent or diminished dorsal gray matter activity but had enhanced ventral activity, particularly contralateral to the stimulation. In another study, Kornelsen and Stroman21 performed fMRI of the spinal cord using active and passive lower limb movement tasks in 12 volunteers with cervical or thoracic SCIs. Activity was detected in all volunteers regardless of the extent of injury. During both active and passive participation, activity was seen caudal to the injury site, although the number of active voxels detected with passive movement was less than with the active movement task. Over all, there are limited clinical studies of spinal cord fMRI, but the technique has the potential for monitoring and anatomically localizing functional areas of intact cord within injured spinal cord.
Brain fMRI and Plasticity after Spinal Cord Injury
Functional MRI studies of the brain show significant reorganizational changes in the sensorimotor cortex (Table 41.2). Kokotilo et al. provide a systematic review.22 Two patterns of activation are generally observed. The first pattern involves alteration of volumes of activation in the motor and associated motor cortices and/or creation of new areas of activation. In the systematic review,22 five studies showed increased activation in several motor cortical areas. Areas of activation common to the five studies included bilateral primary motor cortex (M1), supplementary motor area (SMA), premotor area, cingulate motor area, and contralateral primary somatosensory cortex (S1). In addition, there were new areas of activation in bilateral cerebellum, thalamus, and basal ganglia compared with controls. Three studies reported activation patterns similar to controls, and two studies reported reduced activation in these areas.
The second reorganizational pattern involves changes in somatotopy. This generally takes two forms: posterior displacements of motor activation maxima into the primary somatosensory cortex23,24 and displacement of cortical representations of rostral muscles into areas previously represented by the deafferented area. Examples of the latter include activation of the leg area by hand movements25 or superior and medial shift of activation foci for tongue movements into the hand area.26 In a recent study of six patients with chronic SCI, Lotze et al. demonstrated a displacement of 11.9 mm of biceps brachii cortical maps into the thoracic deafferented area.27 The finding of posterior shifts in motor cortical activity in SCI patients compared with controls is in agreement with an electroencephalographic (EEG) study.28 Increased posterior displacement of motor activity may be related to increased somatomasensory activation due to neuropathic pain or increased contribution of S1 to the corticospinal tract deprived of M1 input.22 In general, mechanisms of reorganization are poorly understood.
Author and date | Study description | Results |
Curt et al., 200229 | fMRI study of nine patients with chronic SCI injuries, L1–4 | No change in somatotopy of upper limb representations; increased activation in M1, SMA, S1, cerebellum, parietal lobe |
Lotze et al., 199930 | fMRI study of four patients with subacute and chronic injuries | Change in somatotopy with cranial shift of elbow representation |
Turner et al., 200324 | fMRI study of 13 patients with chronic SCI injuries between T2 and L1 | Posterior shift of hand motor representation in SCI group |
Mikulis et al., 200226 | fMRI study of nine patients with chronic SCI, C4–7 | Superior and medial shift of tongue movement representations |
Alkadhi et al., 200531 | fMRI study of eight chronic SCI patients, T3–L1 | Greater activation in SCI patients on imagined foot movements |
Cramer et al., 200523 | fMRI study of 12 patients with chronic SCI, C5–T6 | Reduced activation on leg movement; posterior shift of sensorimotor activation during imagined movement |
Lotze et al., 200627 | fMRI study of six patients with chronic SCI T3–T11 | Displacement of elbow cortical maps into deafferented thoracic area |
Jurkiewicz et al., 200732 | Longitudinal fMRI study of six patients with acute C5–8 cervical injury | Initial injury associated with decreased contralateral M1 activation and increased secondary motor activations after injury; increased contralateral M1 activation and decreased secondary motor activation during recovery |
Jurkiewicz et al., 200933 | fMRI study of four patients with persistent paralysis after injury | Progressive decrease in M1 activation and decrease in activation in associated motor areas |
There are a significant number of contradictory results reported by brain fMRI studies in SCI patients. For example, although some studies reported increased motor cortical activation, others found no increases. In patients with thoracic injury, some studies reported that movements of unaffected upper extremity muscles generate greater activation volumes.25,29 Other studies failed to find increases in activation volumes of unaffected upper extremity muscles.30 Imaginary movements of lower extremity affected muscles seem to show increased activation in some studies31 but decreased activation in another study.23 The discrepancies in the literature may be due to differing age groups, injury types, injury levels, and rehabilitation regimens between studies.22
The amount and pattern of activation can be influenced by the completeness of the lesion, the level of injury, the use of rehabilitative interventions, and the time after injury. Kokotilo et al.22 found in their systematic review that 24/25 patients with posterior shifts in activation had complete injuries and suggested that posterior displacements may be more common than anterior displacements in complete SCI. They also noted that posterior shifts of activation maxima may be more common in thoracic injury level and higher lesions (70/83 patients), whereas medial shifts of activation foci tended to occur in lower lesions (24/36 patients). Using positron emission tomography ([15O]-H2O-PET), Bruehlmeier et al.25 studied paraplegics performing hand movements. The extent of activation of contralateral sensorimotor cortex, supplementary motor area, and ipsilateral cerebellum correlated with the number of disconnected spinal segments (i.e., patients with higher thoracic injuries had greater changes in cerebral activation). Curt29 showed greater activation in paraplegic patients compared with tetraplegic patients in response to movements of the wrist and concluded that differences were likely related to differential impairment of wrist movements in tetraplegic patients.
The amount of reorganizational plasticity is a dynamic process that evolves over time. Jurkiewicz et al. performed a longitudinal study of six patients with C5–8 SCI.32 Three patients were studied at 1, 3, 6, and 12 months after injury. Two patients were studied at 0.25, 1, 3, and 12 months and at 0.25, 3, and 6 months. The last patient was studied at 6, 9, and 12 months. Three patients had ASIA A injuries, and three had ASIA B injuries. During a right wrist extension task, fMRI was performed. The outcome was evaluated using ASIA motor scores. The authors reported greater activation of secondary sensorimotor cortical activity and minimal contralateral primary motor cortical (M1) activity at the initial scanning. During recovery, they found increased task-related activation in M1 activation and decreased activation in the secondary motor areas. Although the number of patients was small, the study demonstrated for the first time the evolution of sensorimotor cortical plasticity during recovery from traumatic cervical SCI. In a subsequent study in four tetraplegic patients with persistent paralysis, they found significantly decreased activation in M1 and progressive decrease in activation in the associated sensorimotor areas.33
Brain fMRI Changes after Training
The amount of cortical plasticity can also be influenced by training and rehabilitation. Winchester et al.34 performed fMRI studies before and after 12 weeks of robotic body weight–supported treadmill training in four subjects with chronic SCI. All four subjects showed increased activation of the sensorimotor cortex after training. Substantial improvement in locomotor ability was present in two subjects who showed significant increase in cerebellum. Although the number of subjects was small, it illustrates the potential of fMRI to identify brain areas where activation correlates with training effects.
Conclusion
A variety of neuroimaging tools discussed in this chapter have become available for assessment of SCI. Research using these tools is necessary to assess their value beyond the ASIA clinical exam. The availability of these neuroimaging technologies promises to significantly increase our ability to evaluate SCIs, predict recovery, and assess plasticity after injury.
Pearls
The presence of hematoma or extensive signal change on T2-weighted MRI is generally associated with poor outcomes.
Complete injuries do not disrupt ability of the brain activation with imaginary movements of the impaired muscle. Future technology may be able to harness the preservation of motor cortical activity for activation of a brain neuroprosthesis.
Complete injuries as determined by the ASIA clinical exam do not necessarily mean a complete loss of anatomical continuity of white matter tracts.
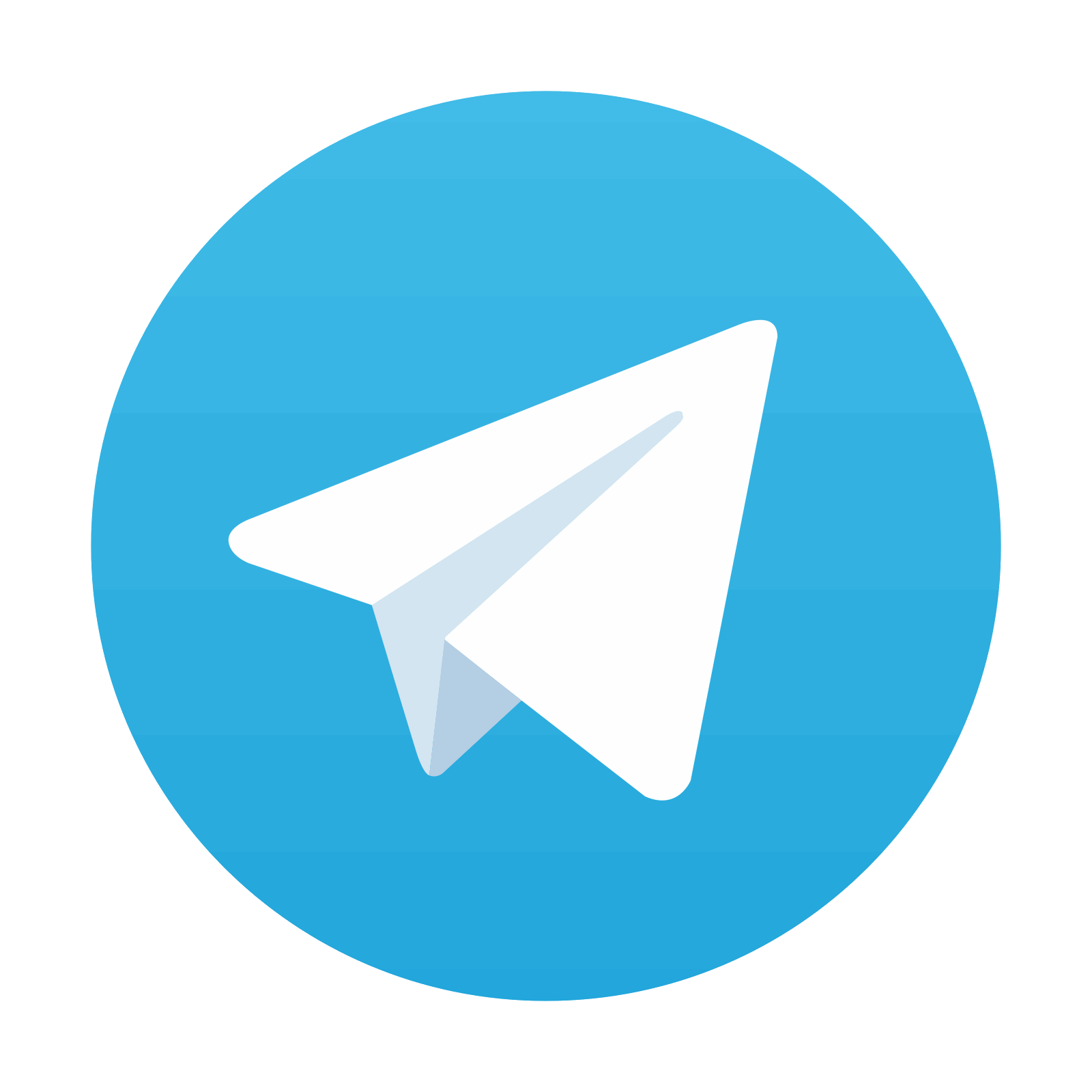
Stay updated, free articles. Join our Telegram channel

Full access? Get Clinical Tree
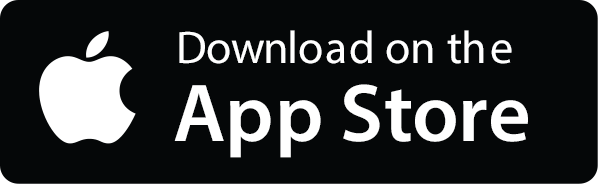
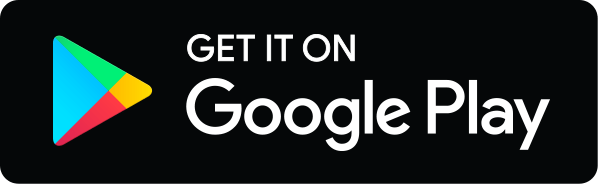