32 Key Points 1. Chondroitin sulfate proteoglycan, the major glial scar–associated matrix protein, is essential for repair at the hyperacute phase following injury and becomes a barrier to regeneration at the chronic stage. 2. Monocyte-derived macrophages, acting as immunoregulatory cells, are pivotal for spinal cord repair and display a distinct role from that of activated resident microglia. 3. An active dialogue takes place between the glial scar and monocyte-derived macrophages, which synchronizes the repair process. 4. Circulating CNS-specific recognizing T lymphocytes orchestrate monocyte recruitment to the injured spinal cord (this function of T cells is referred to as protective autoimmunity). The poor recovery following central nervous system (CNS) insults has been a major issue of research over the last 3 decades; numerous studies have attempted to resolve why such an indispensible, precious, and pivotal organ has such a poor potential for recovery. Several factors have been suggested in attempts to resolve this puzzle, but no fully satisfactory explanation has been found. The changes induced following injury have been collectively assumed to create a hostile microenvironment, which not only is nonpermissive for regeneration but also leads to further damage through a process known as secondary degeneration.1,2 Among such factors are the activation of local immune cells, often referred to as inflammation, and the formation of glial scar tissue following CNS injuries, which are generally considered to be major obstacles for survival, repair, and regeneration3–6 (Fig. 32.1-I1–4). Yet, these two processes are not unique characteristics of the CNS and are in fact pivotal components of the wound healing response in the peripheral nervous system, as well. Yet these two processes are not unique characteristics of the CNS and are, in fact, also pivotal components of the wound healing response in the peripheral nervous system. The perception of the CNS, an immune-privileged site, as a “tissue behind walls,” led to the general belief that any immune response at the injured CNS is destructive, and thus should be suppressed or eliminated. In addition, the well-documented growth-inhibitory nature of the glial scar led to the widespread conception of the glial scar as an obstacle to regeneration and, therefore, as an additional factor contributing to impaired CNS repair. Moreover, the observed spatial and temporal association between glial scar deposition and the local immune response was thought to support the negative roles of both these elements in recovery from CNS injury. Accordingly, research efforts were directed at attempts to block or even circumvent these two presumably hostile processes.7–12 Fig. 32.1 The immunological scenario following spinal cord injury; assistance from systemic immunity is essential. (I) The local immediate response to the insult. Central nervous system insult results in the immediate activation of the local resident microglia (1) and the astrocytes. This activation leads to the creation of a dense structure, termed the glial scar (2). The major matrix component at the glial scar is chondroitin sulfate proteoglycan (CSPG), which is secreted mainly by the reactive astrocytes, and thus accumulates at the lesion margin (3). At this early stage of recovery, CSPG is required to limit the spread of damage by creating a physical barrier. The activated microglia are professional phagocytes, a function that is essential for clearance of debris; however, these cells are also associated with secretion of toxic compounds, including proinflammatory cytokines, such as interleukin (IL)-1, tumor necrosis factor-α (TNF-α), and IL-6, reactive oxygen species (ROS), and nitric oxide (NO). Thus, if the microglial response is uncontrolled or prolonged, this leads to the death of surrounding neurons, a process termed secondary degeneration (4). CSPG contributes to the limitation of this vicious cycle by activating microglia to a beneficial phenotype, which is associated with high levels of insulin-like growth factor (IGF-1) and low levels of TNF-α (5). (II) The assistance from systemic immunity. Both the adaptive (lymphocytes; 1) and the innate (monocytes; 2) arms participate in the immunological response to the insults. The lymphocytes, and more specifically helper T cells, contribute to this scenario by augmenting the recruitment of blood monocytes to the CNS (3) and by regulating the phenotype of microglia via the secretion of Th-2 cytokines (4). Blood monocytes infiltrate the damaged parenchyma and locally differentiate to macrophages (5). These blood-derived macrophages locally acquire an antiinflammatory function, which is associated with the secretion of IL-10, and thereby regulate the microglial response (6). This essential regulatory role of blood-derived macrophages is critically dependent on their interaction with CSPG (7). In turn, the blood-derived macrophages contribute to the timely degradation of this matrix (8) with well-known growth-inhibitory properties, thereby contributing to regeneration. However, more recent studies, pioneered by our group, questioned this blanket view and further revisited the contribution of the local inflammatory response and the scar tissue to the repair of the injured CNS. Essentially, we found that both inflammation13–15 and scar tissue formation16,17 are required for healing following cord insults (Fig. 32.2), and it is their location, level, and timing that determine whether their effect is beneficial or detrimental to the recovering neuronal tissue. This chapter, by summarizing the current experimental studies in the field, presents both faces of these two fundamental responses and suggests a comprehensive view of when, where, and why immune cells and the glial scar are essential for repair following spinal cord insult, and under what conditions they can become obstacles. Although the immune system represents the defense mechanism of the body’s tissues against internal or external threats, irrespective of whether the potential or actual assailants are mechanical, chemical, or biological in nature, the effects of this system on CNS repair are still elusive. From an immunological point of view, the CNS enjoys a privileged status. One of the first lines of evidence for the immune-privileged status of the brain emerged from the studies of Shirai et al.18 demonstrating the successful growth of a rat sarcoma when transplanted into the mouse brain parenchyma, whereas subcutaneous or intramuscular grafts of the tumor were rapidly rejected. These observations, together with the perceived properties of the blood–brain barrier (BBB), resulted in the commonly accepted view of the brain as a sealed organ, deprived of immune interactions.19 Yet the understanding of this “privileged” status had been taken to an extreme as if no immune cell is allowed to enter the CNS from the circulation. We now know that this simplistic view is inaccurate because the degree of immune privilege is not uniform within the CNS, with some areas of the brain known to be quite active immunologically.20 In fact, it is now well established that continuous immunosurveillance occurs in unique compartments of the CNS. Fig. 32.2 The glial scar and blood-derived macrophages are essential for recovery from acute spinal cord injury. (A) Spatial association between the glial scar, represented by reactive astrocytes (red) and the blood-derived macrophages, shown in [Cx3cr1GFP > wt]BM chimeric mice carrying the green fluorescent protein (GFP) label under the control of a myeloid-specific promoter (green). (B) The spread of damage following either conditional ablation of blood-derived macrophages or (C) prevention of the glial scar matrix (CSPG) formation. (From Shechter R, London A, Varol C, et al. Infiltrating blood-derived macrophages are vital cells playing an anti-inflammatory role in recovery from spinal cord injury in mice. PLoS Med 2009;6(7):e1000113; Rolls A, Shechter R, London A, et al. Faces of chondroitin sulfate proteoglycan in spinal cord repair: a role in microglia/macrophage activation. PLoS Med 2008;5(8):e171. Reprinted with permission.) Partly because of the common view of the CNS as an immune-privileged site, the traditional belief was that any immune activity in the damaged CNS is harmful and must therefore be eliminated or suppressed.21–23 In support of this view, mounting evidence indicated that the local inflammatory response is associated with production of toxic substances, known to be produced by various leukocytes, such as reactive oxygen species (ROS) and proinflammatory cytokines. Moreover, possibly due to erroneous projections from inflammatory diseases like multiple sclerosis to other neurodegenerative conditions, it was commonly assumed that local inflammation represents a systemic inflammatory process24 that should be suppressed; therefore, it was persuasively suggested that the therapy of choice following injury should comprise systemic immunosuppressive drugs.23 These drugs were thought to have great promise as a potential therapy for spinal cord injuries. Disappointingly, anti-inflammatory drugs have a narrow therapeutic window, and only a modest effect in the best of cases. Furthermore, because in some cases such treatment even accelerated damage,25–28 a spirited debate has ensued, questioning the significance and efficiency of these sorts of systemic anti-inflammatory treatments. Moreover, potent immunosuppressive treatments were found to be accompanied by increased risk of infectious diseases, the leading cause of morbidity and mortality in patients following acute CNS injury.29 Therefore, the utility of steroids following acute CNS insults is generally limited, and steroids are no longer automatically administered.30 As will be discussed next, over the last few years, additional pieces were added to the puzzle by studies recognizing that the immune response to injury is not uniform in terms of the place, time, and identity of the responding cells. A decade ago, we reported that “alternatively activated” blood macrophages, when locally transplanted at the margin of a spinal cord lesion, resulted in improved recovery.13 The success of such macrophage transplantation was found to be dependent on the site of injection (no effect was found when cells were administered at the center of the lesion or far from its margins), the number of injected cells, and the time elapsed between the injury and the injection.31 The success of this manipulation was received with a high degree of skepticism, primarily due to the lack of appreciation of macrophage heterogeneity within the CNS, a well-established phenomenon in peripheral tissues,32,33 and due to the mistaken view of the entire local immune response as destructive within any immune-privileged site.21,22,34 Moreover, because the site of injury is spontaneously overwhelmed with locally activated microglia, the idea of deriving therapeutic benefit by adding macrophages seemed puzzling. A recent study in a mouse model of spinal cord injury suggested that the missing link lies in the distinction between the contribution of local versus systemic macrophages to both the widely accepted detrimental local inflammatory response and, more crucially, to its resolution.15 The basis of this perception is our discovery of a functional distinction between the CNS-resident macrophages, the so-called microglia, and their peripheral counterparts, the blood-derived macrophages. We found that the blood-derived macrophages, which are recruited to the site of the injury only following the insult (Fig. 32.1-II5), rather than contributing to the ongoing inflammation, terminate the local immune response by displaying an antiinflammatory function required for the regulation of the activated microglia whose activity is apparently poorly controlled15 (Fig. 32.1-II6). Accordingly, recovery from CNS insults is likely to involve similar phases to those occurring in wound repair, in which the first line of defense involves a local inflammatory response needed to clean the site of injury and to create optimal conditions for the repair process. Following this phase, there is an active process of immune termination. Suppressing the immune response at any of these stages is counterproductive and is likely to result in a chronic wound with no repair. However, according to our model, if timely termination does not occur, the blessing of the first phase becomes a curse; the bright side of the local immune response is eclipsed by its negative outcomes, as a prolonged proinflammatory response becomes neurotoxic to the surrounding intact tissue. Outside the CNS, these two phases involve recruitment of naive monocytes from the blood, which locally acquire inflammatory, followed by antiinflammatory, functions, during the first and second phase, respectively.35,36 According to our view, in the CNS, the first phase is mediated by the resident microglia without the need for assistance from circulating monocytes. Yet the termination phase critically depends on the timely and efficient recruitment of blood-derived monocytes. This does not negate the beneficial role of a “classically” activated microglial response at the early posttraumatic phase of the recovery. Rather, we argue that the mere presence of activated microglia/macrophages does not predict whether they will promote inflammation or induce its termination. This distinction calls for a careful definition of inflammation, which should be identified based on the activity of the relevant immune cell populations at specific time points, and not only based on their mere presence or number. These findings not only support the heterogeneity of the macrophage population at the lesion site but also indicate the essential function of blood-derived macrophages for CNS repair. This model requires an entirely different understanding of the role of macrophages in the resolution of CNS damage, versus that suggested previously. The lack of a clear distinction between the origins and functions of various macrophage subpopulations, and disregarding the need for blood-derived monocytes to mitigate damage that the microglia can no longer contain, can, in part, explain the failure of the systemic antiinflammatory drugs. Importantly and unexpectedly, we discovered that as much as these blood monocytes are needed for repair, their spontaneous recruitment is limited, unlike that of the activated resident microglia. Although the reason for the limited recruitment is not completely understood, systemic CD4+ T cells were found to be beneficial in boosting the levels of these cells15,37 (Fig. 32.1-II3). Although the specific distinction between the CNS-resident and blood-derived sub-populations is relevant only to macrophages, many other reports indicated the necessity of additional systemic immune cells of other lineages to enable repair. It was demonstrated almost a decade ago that adaptive immune elements, and more particularly T cells, have a neuroprotective role as part of the physiological response to the CNS trauma, perhaps insufficient in its natural state but amenable to boosting.14,38–42 It was further shown that T cells specific for CNS self-antigens, though not those reactive with irrelevant peptides, are responsible for such CNS protection. Based on these results, we have termed this phenomenon protective autoimmunity.43 Recently, it was shown that beneficial roles of adaptive immunity are not restricted to acute traumas because these cells were found to be essential players for resolution of chronic neurodegenerative diseases, as well.44,45 Intense research in this field revealed that these beneficial autoreactive T cells are responsible, at least in part, for the modulation of the microglial/macrophage phenotype46–48 (Fig. 32.1-II4). T cell–based vaccination results in shifting of the microglial/macrophage phenotype to a noncytotoxic beneficial one, which is mainly associated with low production of TNF-α and high levels of IGF-1.48 Using in vitro experimental systems, it was further demonstrated that the profile of the cytokines secreted from T cells can directly affect the characteristics of the microglia. Moreover, both following acute insult and in chronic degenerative conditions, it was shown that these autoreactive T cells are involved in boosting the spontaneous infiltration of blood monocytes to the injured nervous tissue (Fig. 32.1-II3), which, as described earlier, is essential for the regulation or termination of the local microglial response.15,37 The involvement of adaptive immunity as well as circulating monocytes in the process of repair calls for a reinterpretation of the assumption that destructive local inflammation derives from systemic inflammation. As already discussed, many studies reached opposing conclusions regarding the effects of the immune response, and thus of its inhibition, on repair. We suggest that, although CNS traumas are associated with detrimental local inflammation, this does not imply that the systemic immune system shares such an inflammatory state. Rather, we propose that the systemic immune response must be boosted/recruited to locally mitigate the inflammation and thereby reduce the damage.49 Accordingly, blocking the systemic immune response, as is often tested in the treatment of many neurodegenerative conditions, may be counterproductive by eliminating the capacity of the systemic immune cells to introduce essential regulating and restorative factors into the inflamed area. The glial scar consists predominantly of reactive astrocytes and microglia/macrophages embedded in extracellular matrix molecules, especially chondroitin sulfate proteoglycans (CSPGs).50,51 The glial scar tissue is mainly known for its inhibitory effect on axonal growth.52 Numerous studies attributed the negative aspects of the scar to the growth-inhibitory nature of some of its components. For example, CSPGs have been shown to induce neurite retraction and growth cone collapse in vitro.53 Similarly, studies that compared the effects of different types of astrocytes on neurons revealed that reactive astrocytes, which produce NG2 (also known as CSPG4), inhibit axonal growth.54 Moreover, the developmental role of CSPGs in the CNS is associated with the formation of boundaries because they prevent growing neurons from spreading to sites that are rich in these molecules.55 Other studies indicated that inducing the degradation of CSPGs using specific enzymes, or inhibiting their formation, results in a dramatic increase in axonal growth and regeneration.8,9,56 In light of its well-known activity in limiting axonal growth, the intensive secretion of CSPGs reported to follow CNS traumas has been largely blamed for the lack of axonal regeneration and detrimental outcomes.52 These findings led to general support for therapeutic approaches targeted at scar modulation and resolution. Various approaches have attempted to eliminate and reorganize the chemical components of the glial scar, or to regulate its purportedly negative effects. Such treatments include using degrading enzymes to eliminate scar components (especially CSPGs),8,57 blocking the activity of the growth-inhibitory components,58 regulating intracellular signals induced by the growth-inhibitory compounds,59,60 inhibiting astrocyte proliferation to attenuate scar formation,61 application of growth-inducing agents,62 and others. Obviously, all of these approaches have been based on the perception that the glial scar is an obstacle to recovery that should be modified, eliminated, suppressed, or circumvented. Yet recovery following injury has additional steps that precede regrowth, and the constructive role of the scar in these steps may have been overlooked, probably due to nonoptimal regulation of its resolution in the subsequent stage. Although the growth-inhibitory effect of the scar and of the matrix-bound proteoglycans is the most widely studied phenomenon associated with this issue, other studies have revealed that proteoglycans actually vary in their activities. Studies of developmental processes revealed that the structure of proteoglycans and their availability in the tissue are crucial determinants of their function. Thus, for example, growth-promoting features were demonstrated for oversulfated CSPGs,63,64 and trophic effects were attributed to several CSPGs in their soluble form.65,66 Moreover, as will be discussed in the next sections, accumulating evidence indicates that the scar tissue and its components might have an important role in the immediate response to CNS injury. Despite extensive characterization of the glial scar, there is still no uniform view of its properties and function in CNS recovery. As already discussed, although the scar is mainly recognized as a major growth inhibitor, recent evidence suggests that its participation in CNS repair is much more complex than previously appreciated. In a comprehensive review,17 which was based on our recent studies16 and other accumulating data,67 we proposed that the general view of the scar merely as a barrier for regeneration ignores its positive and protective effects during the acute phase of recovery. The following text summarizes the newly identified beneficial functions of this tissue and suggests how these can be reconciled with its well established growth-inhibitory role. In the acute phase after the injury, the scar tissue is required for sealing the site of injury and to enable tissue remodeling. Astrocytes form a dense scar tissue that has been suggested to demarcate the lesion area and to separate the injured toxic tissue from its still-healthy surroundings.68–70 This impermeable barrier also actively functions to restore homeostasis; astrocytes have an important scavenging activity, which is crucial for regulating excessive levels of glutamate and ions released as a result of injury.71,72 CSPGs, secreted at the lesion margins, create a diffusion barrier for molecules that are potentially harmful to the spared tissue, thereby attenuating the spread of neurotoxicity.68,73 Moreover, astrocytes supply tropic and metabolic support at the injury site.74–76 Owing to their adhesiveness to growth factors, proteoglycans—and more specifically CSPGs—capture these factors, increasing their focal concentration. An additional aspect of the glial scar that may be crucial for neuronal survival following injury relates to its interim activity in filling gaps in the lesion area, creating a scaffold for the vascularization network, and regulating the intensity of blood flow.77 Taken together, these finding indicate that the glial scar has an important role in the immediate response to insult. A series of recent studies indicated that the scar tissue has an additional role in regulating the immunological response at the lesion site boundaries. As mentioned earlier, the phenotype acquired by immune cells and their regulation are both crucial determinants of the functional outcomes of their activity. We recently showed that the scar tissue can control the functional, temporal, and spatial immune activity at the sites of axonal injury.16 Evidence supporting the notion that the glial scar affects immune activity has emerged from recent studies indicating that the scar is required to maintain a balanced inflammatory response. Using various techniques, including conditional ablation of predetermined cell types and specific knockdown transgenic mice, it was demonstrated that reduction of astrocyte migration/activation results in marked and widespread infiltration of inflammatory cells, emphasizing the role of astrocytes in immune regulation.67,69,70,78 Astrocytes can further contribute to immune regulation through the secretion of relevant immune-modulating molecules, such as cytokines79 and proteoglycans. By analogy to the elimination of astrocytes, inhibition of CSPG production using xyloside immediately after acute spinal cord injury results in an alteration of the immune response, manifested by decreased insulin-like growth factor (IGF-1) production by microglia/macrophages, and increased tumor necrosis factor-α (TNF-α) levels16 (Fig. 32.1-I5). Moreover, CSPG spatially and temporally controls the activity of infiltrating blood-derived macrophages16 because it is required for their compartmentalization around the lesion site and for their acquisition of an inhibitory phenotype (Shechter et al., unpublished data; Fig. 32.1-II7). These findings are in agreement with other reports indicating that, in the periphery, CSPGs regulate the motility and activation of macrophages,80 dendritic cells,81 and other immune cell types.82 By virtue of their adhesiveness to chemoattractive agents that are needed for recruiting and activating immune cells,83 proteoglycans capture these factors, increasing their focal concentration, and thereby target the circulating immune cells to the damaged area. This is especially important in the context of the injured CNS, which has low tolerance for immune activity; immune cells that are not confined to the injury site can cause further damage. According to this view, the glial scar, and more specifically CSPG, is needed for neuroprotection; but what about regrowth? As already discussed, although the glial scar participates in sealing the lesion site and modulating immune activity, it is also a major growth inhibitor. Thus many studies have reached opposing conclusions regarding the effects of this tissue and its components on repair. In our opinion, the timing of the scar generation and degradation are crucial in determining its effects.17 In the acute phase after the injury, the scar tissue is required for wound sealing and restoring homeostasis, thereby promoting neuroprotection, whereas in the subsequent phase, further recovery requires axonal regrowth, and thus in these stages, the tissue can benefit from scar degradation. Consistent with this time-dependent view of the scar, application of inhibitors of CSPG production immediately following the insult is counterproductive, whereas their delayed administration has beneficial effects.16 Similarly, it was shown that astrocyte ablation at different time points after injury has distinct effects on the outcome; astrocytes in the acute phase are crucial for recovery, whereas their presence in the chronic phase is inhibitory.67 Interestingly, recent lines of evidence suggest a mutual relationship between infiltrating blood-borne macrophages and the glial scar matrix as a self-contained regulatory mechanism essential for CNS repair. The recruited macrophages, which are activated by CSPG to their essential antiinflammatory phenotype (Fig. 32.1-II7), are induced by this matrix to produce proteases, such as MMP-13, that eventually degrade the matrix, resulting in the temporal restriction of the expression of this matrix molecule.84 Thus the bidirectional relationship between the glial scar and the immune response allows the temporal, spatial, and quantitative regulation of both elements, as required for repair. However, this regulatory process does not always work optimally—the recruitment of systemic immune cells is often insufficient and thus the feedback loop does not optimally operate in the absence of intervention. Although intensive efforts have been directed in attempts to reveal the individual roles of each of the participants in the local response to the insult, there is still no uniform view regarding their contribution to the repair process. Based on our recent studies and other publications over the last few years, we suggest that the debate, dilemma, and conflict all result from ignoring the fact that the response to any insult is a multistep dynamic process in which the needs of the tissue differ at each step (Fig. 32.3). Another source of confusion emerges from the fact that, in many studies, activation of the immune system was based on the use of bacteria or yeast-derived cell-wall components, assuming that these would induce strong immune activation. However, one should bear in mind that those activators, although they induce a strong response, do not mimic endogenous response to trauma but rather induce a different mode of activation suitable for coping with external threats. Undoubtedly, as mentioned earlier, an acute response to injury that is not terminated can turn into a chronic proinflammatory response that is destructive and shares many features with microorganism-induced activities. However, one should not attempt to make inferences from this to the participation of specific immune components in the early steps following the insult. Similarly, we do not argue against the deleterious effects of overwhelming accumulation of CSPG or of specific immune cells, but rather suggest that the timing of both phenomena should be carefully controlled according to the changing requirements of the ongoing dynamic repair process (Fig. 32.3). Over all, the “pearls” and the “pitfalls” of both phenomena, the immune response and glial scar, lie in the timing of their appearance and resolution, as well as the extent of their coordination with the tissue’s needs. Although in the immediate acute phase, the tissue can benefit from neuroprotection and from rescue of the surrounding cells from the toxic environment, in the subsequent phases, regeneration must be supported. Because CSPG and pro-inflammatory cells share opposing effects at these two stages, their timely regulation is essential. CSPG is required at the early stages of recovery to recruit and activate microglia/blood macrophages and to limit the spread of damage by creating a physical barrier, whereas in the chronic postinjury phase, or when present in excessive amounts, its presence inhibits axonal growth. Similarly, proinflammatory cells are needed as part of the initial response for engulfment of toxic components and the recruitment of other essential immune cells; however, their uncontrolled response can lead to neuronal death because their phenotype is associated with the secretion of neurotoxic compounds. Such temporal and spatial regulation is achieved, for example, by the mutual relationship between these two components; CSPG is needed to promote the termination of the proinflammatory response by activating the blood-derived macrophages to an antiinflammatory phenotype, whereas these monocyte-derived cells contribute to CSPG degradation in the subsequent phase (Shechter et al., unpublished data). Because these two phenomena are so closely interrelated, it seems that optimizing one would be sufficient to ensure that the other is synchronized. One such approach is boosting levels of recruited monocytes at the optimal timeframe after the insult.15 Fig. 32.3 The dynamic repair process as a function of the tissue’s needs, and the effects of both the scar and the immune response on these requirements. The main requirements for the rescue and repair at each phase are plotted as “tissue needs” (gray). The effects of the glial scar and the immune response are indicated. The blue box indicates potential beneficial effects, whereas the red box indicates a potentially harmful contribution. As discussed in the text, in the acute/immediate phase, both phenomena are essential for protection and for tissue sparing, whereas their uncontrolled levels and timing in the subsequent phases turn them into obstacles for repair and regeneration.
Glial Scar and Monocyte-Derived Macrophages Are Needed for Spinal Cord Repair: Timing, Location, and Level as Critical Factors
The History of the Understanding of Inflammation in the Context of CNS Repair
The Immunological Response to Injury: A Pivotal Friend If Well Controlled The Innate Component:
The Microglial/Macrophage Response
The Role of Adaptive Immunity: CD4+ T Lymphocytes
The Common Wisdom Regarding the Glial Scar
The Glial Scar: A Pivotal Friend for Protection and a Foe for Regrowth
A Protective Mechanical Barrier
An Immunoregulatory Function
When Does the Scar Tissue Become an Obstacle?
Conclusion
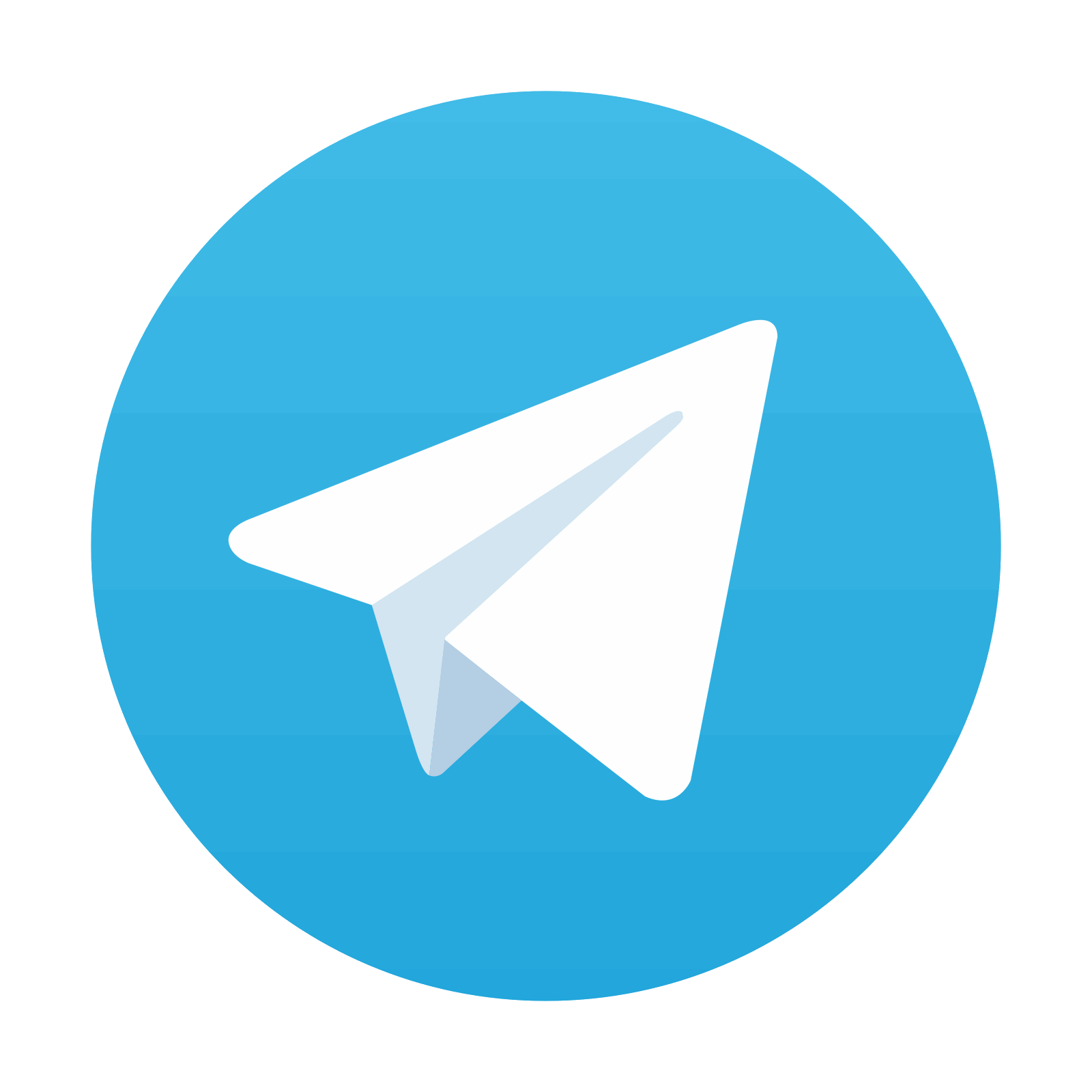
Stay updated, free articles. Join our Telegram channel

Full access? Get Clinical Tree
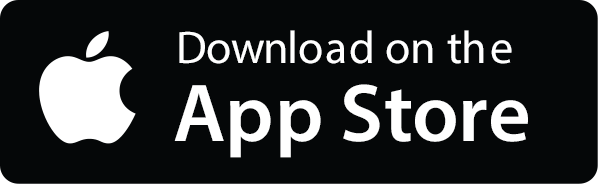
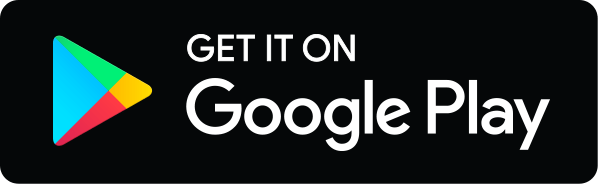